Abstract
Gold nanoparticles have tremendous application in the area of nanotechnology that raises new possibility in the treatment of brain tumor. These nanoparticles can be used for selectively gaining access to tumor due to their small size and modifiability. Gold nanoparticles are functionalized with various molecules such as anticancer drug, transferrin and monoclonal antibody to produce nanocarriers. These nanocarriers have ability to deliver the drug at targeted site. Transferrin crosses the blood-brain barrier because of the receptor-mediated endocytosis. The monoclonal antibody facilitates the release of anticancer drug at targeted sites. This approach of delivery saves the normal cells surrounding the tumor.
Introduction
Nanobiotechnology, defined as biological applications of nanoscale material and structure (usually ranging from 1 to 100 nm), is emerging area of nanoscience and nanotechnology (CitationYezhelyev et al. 2006, CitationSaxena et al. 2010). Gold nanoparticles have tremendous application in sensor, medicine, catalysis and many emerging area of nanotechnology (CitationLim and Zhong 2006). Radiation and chemotherapy were used for the treatment of cancer but these involve harmful side effects such as lowered immune response to infection (CitationCalvert et al. 1993, CitationLeon et al. 2001, CitationShah and Schwartz 2001). Cancer tissue has to create its own blood supply if it has to grow bigger than few millimeters. This causes growth of tumor blood vessels in a process called tumor angiogenesis. Malignancies with the central nervous system pose unique chemotherapeutic problem because the blood–brain barrier (BBB) may limit penetration of antineoplastic drug into brain tumor (CitationSiegal et al. 1995, CitationGrossman 1991, CitationLevin et al. 1980). The major factors governing the passage of chemotherapeutic substance across the BBB (like molecular weight, polarity and lipid solubility) differ from the factors that influence the amount of drug that diffuse passively from capillaries into brain tumor. Other factors like permeability of capillaries with respect to the specific drug, the luminal surface area of the capillaries available for exchange, blood flow into the tumor, the concentration of unbound drug in the plasma and the length of time of drug circulations through the capillaries are also involved in passage of chemotherapeutic materials (CitationSiegal et al. 1995, CitationBlasberg and Groothius 1981). The above problems can be minimized by targeted drug delivery by smart metal nanovectors. Some scientists are working on photo-thermal tumor ablation therapy (CitationGobin et al. 2007, CitationLoo et al. 2005, CitationO’Neal et al. 2004). Photo-thermal tumor therapy is a new method for destruction of tumor (CitationBernardi at al. 2008). Infrared radiation absorbing nanoparticles are called nanoshells and these are a new class of optical tunable nanoparticles composed of a dielectric core (silica) coated with an ultrathin metallic layer (gold) (CitationOldenburg et al. 1999). This type of nanoshell was used in photothermal therapy for the destruction of tumor.
In this article, we will describe the use of biologically synthesized gold nanoparticles for targeted drug delivery because this can reduce the toxicity and have selective drug delivery properties. Gold nanoparticles may have advantages over other metallic particles in terms of biocompatibility and non-toxicity (CitationDhar et al. 20008, CitationShukla et al. 2005) and can be readily conjugated to a large range of biomolecules, such as amino acids (CitationSelvakannan et al. 2004), proteins/enzymes (CitationNiemeyer and Ceyhan 2001), DNA (CitationAlivisatos et al. 1996) and other molecular species without altering the biological activity of the conjugated species. The benefits of targeted drug delivery over conventional resection are numerous; most approaches are minimally or totally non-invasive, relatively simple to perform and have the potential of treating embedded tumors in vital regions where surgical resection is not feasible. Gold nanoparticles are functionalized according to their use. Several reviews concentrating on nanoparticles-biomacromolecules have been published. The nanoparticles featured a mixed monolayer composed of cationic ligands (tetra ethylene glycolylated) and fluorogenic ligands. The cationic nature facilitates the crossing of cell-membrane barrier and fluorophore probes possible drug release mechanism. The hydrophobic dye conjugated gold nanoparticles are non-fluorescent as the gold core quenches fluorescence via energy and/or electron transfer process (CitationAkbuga and Durmaz 1994). The development of colloidal gold (cAu) nanoparticle vector that targets the delivery of tumor necrosis factor (TNF) to a solid tumor growing in mice. The optimal vector, designated PT-cAu-TNF, consists of molecules of thiol-derivatized PEG (PT) and recombinant human TNF that are directly bound onto the surface of the gold nanoparticles (CitationPaciotti et al. 2004). The gold nanoparticles stabilized by heterobifunctional polyethylene glycol (PEG) were synthesized using about 15-nm spherical gold cores and covalently coupled to f19 monoclonal antibodies (CitationEck et al. 2008).
We have mainly focused on the treatment of brain tumor by using biologically synthesized gold nanoparticles as carrier for drug delivery. These gold nanoparticles are functionalized to be attached with other molecules for making them prominent nanocarrier. This nanocarrier has ability to cross BBB and delivered the drug at targeted cells only.
Brain tumor
Cancer is characterized by a reduction or loss of cellular control and normal maturation mechanism. Its features include excessive cell growth, undifferentiated cells and tissues and the ability to grow into neighboring tissues (CitationLubbe et al. 2001). Brain benign tumor can be removed and they seldom grow back. The border or edge of benign tumor can be seen clearly. Benign tumor cells do not spread to other part of body. However, benign tumor can produce pressure on sensitive areas of the brain and cause serious health problems. Malignant tumor is generally more serious and often is life threatening. They grow rapidly and spread to other healthy parts of brain. The cell of malignant tumor can break and spread to other parts of brain, to the spinal cord, or even to other parts of the body. The spread of cancer is called metastasis. The symptoms of brain tumor depend on tumor size, type and location. The treatment of brain tumor depends on their features like size, position, etc. Craniotomy is the usual treatment for the most brain tumor ().
Gliomas, the most prominent primary tumors of the brain are classified on the basis of their origin, for example, Ependymomas originated from ependymal cells, Astrocytomas from astrocytes (glioblastoma multiforme is the most common astrocytoma), and Oligodendrogliomas from oligodendrocytes, moreover, mixed gliomas, such as oligoastrocytomas, contain cells from different types of glia (CitationArko et al. 2006).
Brain tumor biology
Microglia are a type of glial cells that are the resident macrophages of the brain and spinal cord, and thus act as the first and main form of active immune defense in the central nervous system (CNS). Microglia are constantly excavating the CNS for damaged neurons, plaques, and infectious agents (CitationGehrmann et al. 1995). The brain and spinal cord are considered “immune privileged” organs in that they are separated from the rest of the body by a series of endothelial cells known as the BBB, which prevents most infections from reaching the vulnerable nervous tissue. In the case where infectious agents are directly introduced to the brain or cross the BBB, microglial cells must react quickly to decrease inflammation and destroy the infectious agents before they damage the sensitive neural tissue. Due to the unavailability of antibodies from the rest of the body (few antibodies are small enough to cross the BBB), microglia must be able to recognize foreign bodies, swallow them, and act as antigen-presenting cells activating T-cells.
Angiogenesis is a complex process resulting in new blood vessel formation. It is also a fundamental step in the transition of tumors from a dormant state to a malignant one. It involves several coordinated mechanisms including degradation of the basement membrane by proteases, proliferation, and migration of endothelial cells (ECs), lumen formation, basement membrane resembling, recruitment of pericyte or vascular smooth muscle cells (VSMCs), vascular maturation, and finally blood flow (CitationCarmeliet 2005, CitationJain 2003, CitationMarchuk et al. 2003). The extracellular matrix (ECM), the network of proteins surrounding cells in the body, was long believed to function mainly as inert scaffolding for tissue. ECM is a key “signaling molecule” crucial for the normal functioning of cells. That is, the ECM is one of the environmental factors (along with hormones) that communicate with a cell nucleus, modifying nuclear structures and leading to selective gene expression (CitationChen et al. 2000). The angiogenic process is complex and involves endothelial cell proliferation and migration, degradation of the blood vessel basement membrane and associated extracellular matrix. Following endothelial cell proliferation and early tube formation, newly formed vessels differentiate into arterioles and venules, necessary to provide blood supply to tumors. Remodeling of the ECM is an integral component of the angiogenic process. A variety of mechanisms have been documented about how the ECM plays a pivotal role in regulating angiogenesis. Sooner or later during the development of cancer the primary tumor mass spawns pioneer cells that move out, invade adjacent tissues, and circulate to distant organs where they may form new colonies known as invasiveness of cancer.
Macrophages play important role in tumor growth. Macrophages are versatile, plastic cells which respond to micro-environmental signals with distinct functional programs (CitationMantivani et al. 2004). Macrophages have a pleiotropic biological role which includes antigen presentation, target cell cytotoxicity, removal of debris and tissue remodeling, regulation of inflammation, induction of immunity, thrombosis and various forms of endocytosis (CitationAuger and Ross 1992). Macrophages are prominent in tumor tissues and called tumor associated macrophages (TAMs) which are reprogramed by cancer cells. In fact, TAMs actually release a number of promitogenic factors that stimulate the tumor cell proliferation (CitationLewis and Murdoch 2005). Because of their high concentration in tumor tissues, TAMs provide an ideal target for anti-cancer therapies, but selectively delivering drugs to kill TAMs with minimal side-effects is a major hurdle. Nanotechnology is a promising area for the treatment of tumor/cancer more especially by targeted drug delivery to minimize the side effect.
Gold nanoparticles
The bulk gold cannot be used in biomedical application because of their large size; it cannot cross the membrane barrier. But gold has good properties for medical application because of their chemical and physical characteristics. Nanoparticles often behave much differently than bulk samples of the same materials, resulting in unique electrical, optical, chemical, biological, and mechanical properties (CitationCrabtree et al. 2003, CitationKrolikowska et al. 2003, CitationZhao and Stevens 1998).
The gold nanoparticles were synthesized by reduction of chloroauric acid (H[AuCl4]). The formation of gold nanoparticles can be observed by color change of liquid, usually either an intense red (for particles less than 100 nm), or a dirty yellowish color (for large particles). Generally, metal nanoparticles are synthesized and stabilized by using chemical methods such as chemical reduction (CitationKrishna and Goia 2009, CitationTripathi et al. 2010), electrochemical techniques (CitationRodriguez-Sanchez et al. 2000), photochemical reactions in reverse micellers (CitationTaleb et al. 1997) and now days via green chemistry route (CitationAra et al. 2009). Use of plant in synthesis of nanoparticles is quite novel leading to truly green chemistry which provide advancement over chemical and physical method as it is cost effective and environment friendly and in this method there is no need to use high pressure, energy, temperature, and toxic chemical. Now a days we can synthesize the gold nanoparticles by bacteria, fungi, and plant extract (CitationLengke and Southam 2006, CitationXin et al. 2013, Gérrard et al. 2013, CitationRaliya and Tarafdar 2013, Zhang et al. 2012, CitationAhmad et al. 2003, Daniela 2013). Gold nanoparticles can be capable of delivering large molecules, without restricting themselves as nanocarriers of only small molecular drug. The tunable size and functionality of gold nanoparticles make them a useful scaffold for efficient recognition and delivery of biomolecules at targeted site. They have shown success in delivery of peptides, proteins, or nucleic acid like DNA or RNA (CitationGhosh et al. 2008).
Biosynthesis of gold nanoparticles
The emergence of nanotechnology and its applications in agriculture, medical, cancer therapy, diagnostics, drug delivery, and tissue engineering have opened up potential benefits to mankind. Many unknown biological processes are now being studied to understand the formation of new nanomaterials and their antibacterial activity (CitationTripathi et al. 2010). Biogenic gold nanoparticles were functionalized to use as biosensing enhancement (CitationTorres-Chavolla et al. 2010). Chemical methods have been used for the synthesis of nanostructured materials (CitationJana et al. 2001, CitationLin et al. 2004, CitationEl-Sayed 2001). Chemical methods have some demerits like being toxic in nature, costly, non-environmental friendly, etc. Here we describe the various biological reducing as well as capping agents for the synthesis of gold nanoparticles. Many microorganisms, both unicellular and multicellular have been reported to produce inorganic materials either intracellularly or extracellularly. Moreover the preparation of nanoparticles of desired quality with low cost is difficult by conventional methods and offer potential benefits. Biological methods of nanostructured material synthesis are nontoxic and environment-friendly and thus some well known examples cited in literature are as follows.
Bacteria
Some bacteria like Methylosinus trichosporium 3011 have been reported to synthesize gold nanoparticles (CitationXin et al. 2013). Rhodopseudomonas capsulata has been screened and found to successfully produce gold nanoparticles of different sizes and shapes (Gérrard et al. 2013).
Fungi
Use of various fungi also has been made to obtain large quantities of metal nanoparticles. Fusarium oxysporum has been used to synthesize gold and silver nanoparticles (CitationAhmad et al. 2003, CitationMukherjee et al. 2002).
Algae
Various Algae have been used to obtain metal nanoparticles. Such as Sargassum wightii has been used to synthesize gold nanoparticles (CitationSingaravelu et al. 2007). Chlorella vulgaris have been reported to synthesize single-crystalline gold nanoplates (CitationXie et al. 2006).
Plants
Various Plant extracts have also been used to synthesize the metal nanoparticles. Gold and silver nanoparticles are synthesized by Neem (Azadirachta indica) extract (CitationShankar et al. 2004). Alfalfa plants extract was used for the synthesis of gold nanoparticles (CitationGardea-Torresdey et al. 2002). Lemongrass extract was used to synthesize Au core-Ag shell nanoparticles (CitationRai et al. 2007) and lemongrass extract was reported for the synthesis of triangular gold nano-prisms (CitationShankar et al. 2004).
The formation of nanoparticles was characterized by using UV-Vis spectroscopy, dynamic light scattering (DLS) and Fourier transform infrared spectroscopy (FTIR). The morphology of silver nanoparticles was confirmed by transmission electron microscopy (TEM).
Functionalization of gold nanoparticles
Nanotechnology is the development and improvement in the use of fluorescent marker for the diagnostic and screening purpose. Drug delivery systems (DDS) provide useful adjuncts for therapeutics, including drugs, nucleic acid, and proteins (CitationLanger and Tirrell 2004). DDS can also be used for target specific tissues and cell types through appropriate functionalization. A diverse range of materials have been used as carrier, including linear polymer (CitationSershen and West 2002), dendrimers (CitationPaleos et al. 2004), and nanomaterials like nanoparticles (CitationLuo and Saltzman 2000). Mixed monolayer-protected clusters with gold cores provide desirable attitudes for use as carriers in DDS:
(1) Gold core is essentially inert and non-toxic (CitationConnor et al. 2005, CitationJoshi et al. 2006).
(2) Monodispersed nanoparticles can be formed with sizes 1.5–10 nm (CitationVerma and Roltella 2005).
(3) The monolayer can be tailored with a range of ligands, providing effective cellular uptake, controlled payload release and targeting a specific cell.
Biologically synthesized gold nanoparticles can be used as a nanocarrier for anticancer drug delivery in brain tumor. Gold nanoparticles will be functionalized with various materials to provide free group for the attachment of desirable materials. The functionalized gold nanoparticles will allow the desired molecule to bind on it. The anticancer drug and specific antibody (monoclonal antibody) are attached on the surface of functionalized gold nanoparticles. The monoclonal antibody facilitates the release of anticancer drug at specific site. The hetero-bifunctional PEG ligands contain a dithiol group for stable anchoring onto the gold surface and a terminal carboxy group for coupling of antibodies to the outside of the PEG shell (CitationEck et al. 2008). Ortho-pyridyl-disulfide-n-hydroxysuccinimide polyethylene glycol polymer (OPSS-PEG-NHS) was used to tether antibodies onto the surface of gold nanoparticles (CitationLoo et al. 2004). Gold nanoparticles are surface functionalized by hetero-bifunctional poly(ethylene glycol) having a thiol group on one terminus and a reactive functional group on the other to use as a flexible spacer. Coumarin, a model fluorescent dye, was conjugated to one end of the PEG spacer and gold nanoparticles were modified with coumarin-PEG-thiol (CitationShenoy et al. 2006). Gold nanoparticles were functionalized by thiol-derivatized PEG for attachment of Tumor Necrosis Factor (TNF). TNF conjugated gold nanoparticles were used in treatment of tumor in mice (CitationPaciotti et al. 2004). The targets of drug can be visualized by use of fluorescent nanomaterials having exceptional photostability, allowing the emission of fluorescent light over a long time without a rapid decline in emission (i.e., photobleaching). Gold nanoparticles were functionalized to produce negative charge on the surface of nanoparticles so that anticancer drug, monoclonal antibodies, and transferrin will be attached on it (). The above bioconjugated nanocarrier molecules facilitate to cross the BBB by receptor-mediated endocytosis. This type of approach will deliver the drug at targeted site and protect the normal cells surrounding the tumor.
Drug targeting
Nanocapsules mean sandy nanoparticles that consist of shell and a space, in which desired substances may be placed. Drug filled nanocapsules can be covered with antibodies or cell-surface receptors that bind to the cancer or various cells and release their biological compound on the contact with that tissue. Nanoparticulates in drug delivery systems can exploit a characteristic of solid tumors such as the enhanced permeability and retention (EPR) effect in which tumor tissues show peculiar characteristic properties such as hyper vasculature, defective vascular architecture and a deficient lymphatic drainage which lead macromolecules and particulates to be accumulated preferentially and to be retained for a longer time in tumor (CitationNatalie et al. 2007); this type of transportation according to the size of nanoparticles is known as passive targeting (). But, nanoparticles can be modified to target inherent characteristics of cancer cells such as rapid proliferation and particular antigen presentation (CitationMoghimi et al. 2001) and this type of targeting is known as active targeting (). The nanocapsules are attached to bioadhesive materials which is performed either by incorporating a bioadhesive material in the pH-sensitive microsphere matrix, or by using a bioadhesive material in the nanocapsules matrix in conjunction with a bioadhesive material in the microsphere matrix. Thus, nanocapsules were designed to release the drug over an extended period of time by dissolving/swelling the microsphere at a pH that is typically found in or near cancerous tissue.
Mechanism of drug delivery across BBB
The BBB is a homeostatic defense mechanism of the brain that restricts the passage of solutes. The brain cells are tightly packed so that drugs have to move through the cells themselves and astrocytes (star shaped glial cells in brain) provide biochemical support of endothelial cells which form the BBB (CitationPerdan et al. 2009). This approach makes powerful barrier and very few drug can cross it. BBB has receptors for several substrates like insulin and transferrin, etc. These receptors regulate the entry of molecules inside the brain. Receptor mediated drug delivery is a prominent method to deliver the drug across the BBB.
Nanotechnology manipulates the complex biological systems with high selectivity and timing than the conventional pharmacological approaches such as BBB (CitationModi et al. 2009). Some previous reporters have used transferrin for receptor mediated drug delivery to cross the BBB (CitationYang et al. 2005, CitationLi and Qian 2002, CitationQian et al. 2002). The intratumoral drug distribution is an important issue with conventional method because of physiological properties of tumor like their cellular packing and limited diffusion of drug. The distribution of drug can be enhanced by active targeting. In active targeting the drug will release inside the cell by the action of monoclonal antibody. Biologically synthesized gold nanoparticles are functionalized to upload the drug, transferrin, and monoclonal antibody (). This nanocarrier will be able to transfer the anticancer drug to targeted site only because antibody has the higher affinity with the tumor cells. BBB cannot allow any molecule to enter into the brain, but the above suggested nanocarrier is conjugated with transferrin which is attached to transferrin receptor of BBB () and cross the barrier by endocytosis (CitationYang et al. 2005).
Figure 5. Diagrammatic representation of receptor-mediated endocytosis to cross the BBB and deliver the drug at targeted site only. (A) Representation of BBB, (B) Intravenously injection of nanocarrier, (C) Transferrin attached to BBB receptor. (D, E & F) Receptor mediated endocytosis of nanocarrier and reached into brain by exocytosis, (G) Finally, nanocarrier attached with tumor cells only through monoclonal antibody.
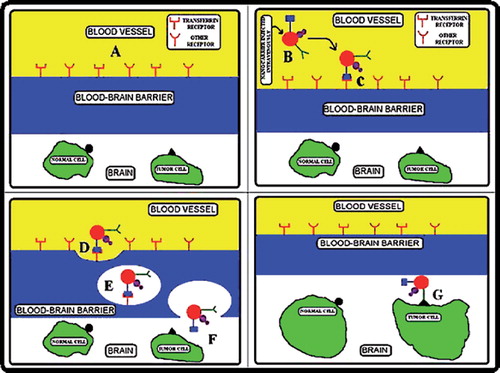
Conclusions
In this review article we have described the targeted drug delivery by biologically synthesized gold nanoparticles for the treatment of brain tumor. We have witnessed the use of biologically synthesized gold nanoparticles in developing a new generation of more effective brain tumor therapies capable of overcoming the many biological, biophysical, and biomedical barriers that the body stages against conventional brain tumor therapies. Biologically synthesized gold nanoparticles are functionalized to attach with anticancer drug, transferrin, and monoclonal antibody. Transferrin has ability to cross the blood–brain barrier (BBB) by receptor-mediated endocytosis. The use of nanoparticles conjugated to monoclonal antibodies allows the possibilities of simultaneously detecting multiple molecular targets in brain tumor, on which treatment decisions can be made. This approach of targeted drug delivery is a promising method to deliver drug at targeted site and save the normal cells surrounding the tumor.
Acknowledgements
Authors are thankful to Dr R. P. Singh (Advisor) and Dr L. M. Bhardwaj (HOI), Amity Institute of Nanotechnology, Amity University, Noida, India for their encouragement and giving precious advice continuously for the above review article.
Declaration of interest
The authors report no conflicts of interest. The authors alone are responsible for the content and writing of the paper.
References
- Ahmad A, Mukherjee P, Senapati S, Mandal D, Khan MI, Kumar R, Sastry M. 2003. Extra-cellular biosynthesis of silver nanoparticles using the fungus, Fusarium oxysporum. Colloid Surf B. 28:313–318.
- Akbuga J, Durmaz G. 1994. Preparation and evaluation of cross linked chitosan microspheres containing furosemide. Int J Pharma. 11: 217–222.
- Alivisatos AP, Peng X, Wilson TE, Loweth CL, Bruchez Jr MP, Schultz PG. 1996. Organization of ‘nanocrystal molecules’ using DNA. Nature. 382:609–611.
- Ara BN, Mondal MS, Basu S, Laskar RA, Mand D. 2009. Biogenic synthesis of Au and Ag nanoparticles using aqueous solutions of Black Tea leaf extracts. Colloid Surf B. 71:113–118.
- Arko L, Katsyv I, Park GE, Luan WP, Park JK. 2006. Experimental approaches for the treatment of malignant gliomas. Pharmacol Ther. 128:1–36.
- Auger MJ, Ross JA. 1992. The Biology of Macrophage. Oxford: Oxford University Press, pp. 2–74.
- Bernardi RJ, Lowery AR, Thompson PA, Blaney SM, West JL. 2008. Immunonanoshells for targeted photothermal ablation in medulloblastoma and glioma. J Neurooncol. 86:165–172.
- Blasberg RG, Groothius DR. 1981. Chemotherapy of brain tumor. Physiological and pharmacokinetic considerations. Semin Oncol. 13:70–82.
- Calvert H, Judson I, Van der Vijgh WJF. 1993. Cancer Surveys: Pharmacokinetics and Cancer Chemotherapy. New York: Cold Spring Harbor Laboratory Press, pp. 189–218.
- Carmeliet P. 2005. Angiogenesis in life, disease and medicine. Nature. 438:932–936.
- Chen M, Schmeichel K, Mian IS, Lelièvre SA, Petersen OW, Bissell MJ. 2000. AZU-1: A candidate breast tumor suppressor and biomarker for tumorigenic reversion. Mol Biol Cell. 11:1357–1367.
- Connor EE, Mwamuka J, Gole A, Murphy CJ, Wyatt MD. 2005. Gold nanoparticles are taken up by human cells but do not cause cytotoxicity. Small. 1:325–327.
- Crabtree JH, Brruchette RJ, Siddiqi RA, Huen IT, Handott LL, Fishman A. 2003. Construction and use of stencils in planning for peritoneal dialysis catheter implantation. Perit Dial Int. 23:395–398.
- Correa-Llantén DN, Muñoz-Ibacache SA, Castro ME, Muñoz PA, Blamey JM. 2013. Gold nanoparticles synthesized by Geobacillus sp. strain ID17 a thermophilic bacterium isolated from Deception Island, Antarctica. Microb Cell Fact. 12:1–6.
- Dhar S, Reddy EM, Shiras A, Pokharkar V, Prasad BLV. 2008. Natural gum reduced/stabilized gold nanoparticles for drug delivery formulations. Chem Eur J. 14:10244–10250.
- Eck W, Craig G, Sigdel A, Ritter G, Old LJ, Tang L, et al. 2008. PEGylate gold nanoparticles conjugated to monoclonal F19 antibodies as targeted labeling agents for human pancreatic carcinoma tissue. ACS Nano. 2:2263–2272.
- El-Sayed MA. 2001. Some interesting properties of metals confined in time and nanometer space of different shapes. Accounts Chem Res. 34:257–264.
- Gardea-Torresdey JL, Parsons JG, Gomez E, Peralta-Videa J, Troiani HE, Santiago P, Jose Yacaman M. 2002. Formation and Growth of Au Nanoparticles inside Live Alfalfa Plants. Nano Lett. 2:397–401.
- Gehrmann J, Matsumoto Y, Kreutzberg GW. 1995. Characterisation of two new monoclonal antibodies directed against rat microglia. Brain Res Rev. 20:269–287.
- Ghosh P, Han G, De M, Kim CK, Rotello VM. 2008. Gold nanoparticles in drug delivery applications. Adv Drug Deliv Rev. 60:1307–1315.
- Gobin AM, Lee MH, Halas NJ, James WD, Drezek RA, West JL. 2007. Near-infrared resonant nanoshells for combined optical imaging and photothermal cancer therapy. Nano Lett. 7:1929–1934.
- Grossman SA. 1991. Chemotherapy of brain tumor. In: Salcman M, Ed. Neurobiology of Brain Tumor. Concepts in Neurosurgery. Baltimore: Williams & Wilkins, pp. 321–340.
- Jain RK. 2003. Molecular regulation of vessel maturation. Nat Med. 9:685–693.
- Jana NR, Gearheart L, Murphy CJ. 2001. Wet chemical synthesis of high aspect ratio cylindrical gold nanorods. J Phys Chem B. 105: 4065–4067.
- Joshi HM, Bhumkar DR, Joshi K, Pokharkar V, Sastry M. 2006. Gold nanoparticles as carriers for efficient transmucosal insulin delivery. Langmuir. 22:300–305.
- Krishna B, Goia Dan V. 2009. Silver nanoparticles for printable electronics and biological applications. J Mater Res. 24:2828–2883.
- Krolikowska A, Kudelski A, Michota A, Bukowska J. 2003. SERS studies on the structure of thioglycolic acid monolayers on silver and gold. Surf Sci. 532:227–232.
- Langer R, Tirrell D. 2004. Designing materials for biology and medicine. Nature. 428:487–492.
- Lengke M, Southam G. 2006. Bioaccumulation of gold by sulfate-reducing bacteria cultured in the presence of gold(I)-thiosulfate complex. Geochim Cosmochim Acta. 70:3646–3661.
- Leon X, Quer M, Orus C. 2001. Results of salvage surgery for local or regional recurrence after larynx preservation with induction chemotherapy and radiotherapy. Head Neck-J Sci Spec. 23:733–738.
- Levin VA, Patlak CS, Landahl HD. 1980. Heuristic modeling of drug delivery to malignant brain tumor. J Pharmacokinet Bio-pharm. 8:257–296.
- Lewis C, Murdoch C. 2005. Macrophage responses to hypoxia: implications for tumor progression and anti-cancer therapies. Am J Pathol. 167:627–635.
- Li H, Qian ZM. 2002. Transferrin/transferrin receptor-mediated drug delivery. Med Res Rev. 22:225–250.
- Lim SI-I, Zhong C-J. 2006. Molecularly-mediated assembly of nanoparticles. Gold Bull. 40:59–66.
- Lin XZ, Terepka AD, Yang H. 2004. Synthesis of silver nanoparticles in a continuous flow tubular microreactor. Nano Lett. 4:2227–2232.
- Loo C, Lin A, Hirsch L, Lee MH, Barton J, Halas N, West J, Dreze KR. 2004. Nanoshell-enabled photonics-based imaging and therapy of cancer. Technol Cancer Res Treat. 3:33–40.
- Loo C, Lowery A, Halas N, West J, Drezek R. 2005. Immunotargeted nanoshells for integrated cancer imaging and therapy. Nano Lett. 5:709–711.
- Lubbe AS, Alexiou C, Bergemann C. 2001. Clinical applications of magnetic drug targeting. J Surg Res. 95:200–206.
- Luo D, Saltzman WM. 2000. Enhancement of transfection by physical concentration of DNA at cell surface. Nat Biotechnol. 18:893–895.
- Mantovani A, Allavena P, Sica A. 2004. Tumour-associated macrophages as a prototypic type II polarized phagocyte population: role in tumour progression. Eur J Cancer. 40:1660–1667.
- Marchuk DA, Srinivasan S, Squire TL, Zawistowski JS. 2003. Vascular morphogenesis: tales of two syndromes. Hum Mol Genet. 12:97–12.
- Modi G, Pillay V, Choonara YE, Ndesendo VMK, Toit LC, Naidoo D. 2009. Nanotechnological applications for the treatment of neurodegenerative disorders. Prog Neurobiol. 88:272–275.
- Moghimi SM, Hunter AC, Morray JC. 2001. Long-circulating and target-specific nanoparticles: theory to practice. Pharmacol Rev. 53:283–288.
- Mukherjee P, Senapati S, Mandal D, Ahmad A, Khan MI, Kumar R, Sastry M. 2002. Extracellular synthesis of gold nanoparticles by the fungus Fusarium oxysporum. ChemBioChem. 5:461–463.
- Natalie P, Praetorius Mandal TK. 2007. Engineered nanoparticles in cancer theraphy. Recent Pat Drug Deliv Formul. 1:37–51.
- Niemeyer CM, Ceyhan B. 2001. DNA-directed functionalization of colloidal gold with proteins. Angew Chem Int Edit. 113:3798–3801.
- Oldenburg SJ, Jackson JB, Westcott SL, Halas NJ. 1999. Infrared extinction properties of gold nanoshells. Appl Phys Lett. 75: 2897–2899.
- O’Neal DP, Hirsch LR, Halas NJ, Payne JD, West JL. 2004. Photo-thermal tumor ablation in mice using near infrared-absorbing nanoparticles. Cancer Lett. 209:171–176.
- Paciotti GF, Myer L, Weinreich D, Goia D, Pavel N, McLaughlin RE, Tamarkin L. 2004. Colloidal Gold: A novel nanoparticle vector for tumor directed drug delivery. Drug Deliv. 11:169–183.
- Paleos CM, Tsiourvas D, Sideratou Z, Tziveleka L. 2004. Acid- and salt-triggered multifunctional poly(propylene imine) dendrimer as a prospective drug delivery system. Biomacromolecules. 5:24–52.
- Perdan K, Lipnik-Stangelj M, Krzan M. 2009. The impact of astrocytes in the clearance of neurotransmitters by uptake and inactivation. Adv Planar Lipid Bilayers Liposomes. 9:211–215.
- Poinern GEJ, Chapman P, Shah M, Fawcett D. 2013. Green biosynthesis of silver nanocubes using the leaf extracts from Eucalyptus macrocarpa. Nano Bull. 2:130101–130107.
- Qian ZM, Li H, Sun H, Ho K. 2002. Targeted drug delivery via the transferrin receptor-mediated endocytosis pathway. Pharmacol Rev. 54:561–567.
- Rai A, Chaudhary M, Ahmad A, Bhargava S, Sastry M. 2007. Synthesis of triangular Au core-Ag shell nanoparticles. Mater Res Bull. 42:1212–1220.
- Raliya Ramesh, Tarafdar JC. 2013. Biosynthesis of gold nanoparticles using Rhizoctonia bataticola TFR-6. Adv Sci Eng Med. 5:1–4.
- Rodriguez-Sanchez L, Blanco MC, Lopez-Quintela MA. 2000. Electrochemical synthesis of silver nanoparticles. J Phys Chem B. 104: 9683–9688.
- Saxena A, Tripathi RM, Singh RP. 2010. Biological synthesis of silver nanoparticles by using onion (Allium cepa) extract and their antibacterial activity. Dig J Nanomater Bios. 5:427–432.
- Selvakannan PR, Mandal S, Phadtare S, Gole A, Pasricha R, Adyanthaya SD, Sastry M. 2004. Water-dispersible tryptophanprotected gold nanoparticles prepared by the spontaneous reduction of aqueous chloroaurate ions by the amino acid. J Colloid Interf Sci. 12:97–102.
- Sershen S, West J. 2002. Implantable, polymeric systems for modulated drug delievery. Adv Drug Del Rev. 54:1225–1235.
- Shah MA, Schwartz GK. 2001. Cell cycle-mediated drug resistance: an emerging concept in cancer therapy. Clin Cancer Res. 7: 2168–2181.
- Shankar SS, Rai A, Ahmad A, Sastry M. 2004. Rapid synthesis of Au, Ag, and bimetallic Au core-Ag shell nanoparticles using Neem (Azadirachta indica) leaf broth. J Colloid Interf Sci. 275:496–502.
- Shenoy D, Fu W, Li J, Crasto C, Jones G, DiMarzio C, Sridhar S, Amiji M. 2006. Surface functionalization of gold nanoparticles using hetero-bifunctional poly(ethylene glycol) spacer for intracellular tracking and delivery. Int J Nanomedicine. 1:51–57.
- Shukla R, Bansal V, Chaudhary M, Basu A, Bhonde RR, Sastry M. 2005. Biocompatibility of gold nanoparticles and their endocytotic fate inside the cellular compartment: a microscopic overview. Langmuir. 21:10644–10654.
- Siegal T, Horowitz A, Gabizon A. 1995. Doxorubicin encapsulated in sterically stabilized liposomes for the treatment of a brain tumor model: biodistribution and therapeutic efficacy. J Neurosurg. 83: 1029–1037.
- Singaravelu G, Arockiamary JS, Ganesh VK, Govindaraju K. 2007. A novel extracellular synthesis of monodisperse gold nanoparticles using marine alga, Sargassum wightii Greville. Colloid Surf B. 57:97–101.
- Taleb A, Petit C, Pileni MP. 1997. Synthesis of highly monodisperse silver nanoparticles from AOT reverse micelles: a way to 2D and 3D self-organization. Chem Mater. 9:950–959.
- Torres-Chavolla E, Ranasinghe RJ, Alocilja EC. 2010. Characterization and functionalization of biogenic gold nanoparticles for biosensing enhancement. IEEE Trans Nanotech. 9:533–538.
- Tripathi RM, Saxena A, Gupta N, Kapoor H, Singh RP. 2010. High antibacterial activity of silver nanoparticles against E. coli MTCC 1302, S. typhimurium MTCC 1254, B. subtilis MTCC 1133 and P. aeruginosa MTCC 2295. Dig J Nanomater Bios. 5:323–330.
- Verma V, Roltella VM. 2005. Surface recognition of bio macromolecules using nanoparticle receptors. Chem Commun. 3: 303–312.
- Xie J, Lee JY, Wang DIC, Ting YP. 2006. Identification of active biomolecules in the high-yield synthesis of single-crystalline gold nanoplates in algal solutions. Small. 3:672–682.
- Xin J-Y, Cheng D-D, Zhang L-X, Lin K, Fan H-C, Wang Y, Xia C-G. 2013. Methanobactin-mediated one-step synthesis of gold nanoparticles. Int J Mol Sci. 14:21676–21688.
- Yang PH, Sun X, Chiu JF, Sun H, He QY. 2005. Transferrin-mediated gold nanoparticles cellular uptake. Bioconjugate Chem. 16:494–496.
- Yezhelyev MV, Gao X, Xing Y, Al-Hajj A, Nie S, O’Regan RM. 2006. Emerging use of nanoparticles in diagnosis and treatment of breast cancer. Lancet Oncol. 7:657–667.
- Zhang YX, Zheng J, Gao G, Kong YF, Zhi X, Wang K, Zhang XQ, Cui Da X. 2011. Biosynthesis of gold nanoparticles using chloroplasts. Int J Nanomedicine. 6:2899–2906.
- Zhao G, Stevens JSE. 1998. Multiple parameters for the comprehensive evaluation of the susceptibility of Escherichia coli to the silver ion. Biometals. 11:27–32.