Abstract
Metoprolol succinate is a very potent drug for the treatment of hypertension but suffers from poor bioavailability due to its erratic absorption in lower GI tract. Therefore, in the present study, it was hypothesized that by formulating mucoadhesive particles, the residence time in the GIT and release of drug may be prolonged that will enhance the bioavailability of metoprolol succinate. Metoprolol succinate loaded chitosan microparticles were prepared by ionic gelation method. The optimized microparticles were coated with sodium alginate to form a layer over chitosan microparticles to increase the mucoadhesive strength and to release the drug in controlled manner. Coated and uncoated microparticles were evaluated for particle size, zeta potential, morphology, entrapment efficiency, drug loading and in vitro drug release. The coated microparticles showed comparatively less drug release in the 0.1 N HCl while sustained release in PBS (pH 6.8) as compared to uncoated microparticles. The in vivo study on albino rats demonstrated an increase in bioavailability of the coated microparticles as compared to marketed formulation. From the study it can be concluded that alginate coated chitosan microparticles could be a useful carrier for the oral delivery of metoprolol succinate.
Introduction
Metoprolol succinate is a β1-selective adrenergic blocker and antihypertensive agent. It has an oral bioavailability of 40%, because of its poor absorption in lower Gastrointestinal tract (GIT) . The drug is freely soluble in water and is administered at a dose of 100 mg daily (CitationKendall et al. 1991). Several oxidative pathways including a-hydroxylation, O-demethylation and N-dealkylation extensively metabolize the drug (CitationLennard et al. 1982). Conventional dosage form fails to maintain the drug plasma concentration over the extended period of time, due to short half-life ˜ 3 to 4 h (CitationBrain and Robert 1996). Mucoadhesive drug delivery system is one of the important tools to prolong the residence time of the drug at the absorption site, allowing intensified contact with the epithelial barrier (CitationHagerstrom et al. 2003).
A wide range of polymers from synthetic, semi synthetic and natural origin like carbopol, polycarbophil, sodium carboxymethylcellulose (SCMC), xanthan and hydroxyl propyl methyl cellulose (HPMC) (CitationPeh and Wong 1999) have been explored for the formulation of bioadhesive systems but none of these polymers possess all the characteristics of an ideal polymer for a bioadhesive system. Various delivery systems including tablets, films, patches, microspheres, gels, beads, etc. have been developed so far using these mucoadhesive polymers (CitationBoddupalli et al. 2010).
Microparticles have been potential to be used for targeted and controlled release drug delivery, but coupling of mucoadhesive properties with microparticles provides various additional advantages like efficient absorption and enhanced bioavailability of the drug due to high surface to volume ratio, a much more intimate contact with the mucus layer, specific targeting of the drug to the absorption site, better control of systemic drug delivery etc.
Chitosan, because of its unique properties like non- toxicity, antimicrobial characteristic, solubility, poly cationic character, physical attributes, biocompatibility and biodegradability, has gained much attention for oral delivery of therapeutics (CitationGeorge and Abraham 2006, CitationHe et al. 1998). It has already been reported that chitosan increased the absorption of drugs through intestinal epithelial membrane by opening the tight junctions. CitationPalanisamy et al. (2009) prepared chitosan microparticles of metoprolol succinate by cross linking technique. Result of the study showed that drug released over extended period of time with minimal dose however; a significant amount of drug was released in gastric environment. This degradation can be prevented by providing a superficial coating to the microparticles that may release minimum amount of drug in the stomach.
Sodium alginate has been explored for sustained, controlled and oral delivery of therapeutics. Further, it can protect chitosan particles from acidic environment thus drug release in the stomach will be reduced. Moreover, coating of chitosan microparticles with sodium alginate may increase the residence time in the intestine due to bioadhesive nature of the sodium alginate. Thus, bioavailability of metoprolol succinate might be improved.
Materials and methods
Metoprolol succinate was received from Akums Pharmaceutical Limited, Haridwar (Uttarkhand), India as a gift sample; Chitosan (low molecular weight) with deacetylation (DA) of ≥ 75% and viscosity of 20–300 cps (1 wt.% in 1% acetic acid at 25°C), Tri polyphosphate (TPP) were purchased from Sigma Aldrich (USA); Sodium alginate, Calcium chloride were purchased from Himedia (Mumbai, India). All the reagents used in the study were of analytical grade.
Preparation of alginate coated chitosan microparticles
Preparation of chitosan microparticles
Metoprolol succinate loaded chitosan microparticles were prepared by the ionic gelation method (CitationPatel 2010). Briefly, chitosan solution (1%) was prepared by dissolving it in aqueous acetic acid solution (0.5% v/v). Metoprolol succinate, in varying drug to polymer weight ratios of 1:5, 2:5, 3:5 and 4:5, was added to aqueous TPP solution (0.5%). TPP drug solution (10 ml) was added drop wise to the chitosan solution (20 ml) under continuous stirring at room temperature. The chitosan microparticles were formed spontaneously. After 30 min of continuous stirring, chitosan microparticles were collected by centrifugation (3K-30 High Speed Cooling Centrifuge, Sigma Laborzentrifugen, India) at 20,000 rpm for 30 min and supernatant so obtained was discarded (CitationXing et al. 2008).
Preparation of sodium alginate coated chitosan microparticles
Chitosan microparticles prepared in the first step were used for the preparation of alginate coated microparticles. Sodium alginate solutions in varying core (chitosan) to coat (sodium alginate) ratio were added drop wise into chitosan microparticles dispersion under mild agitation. After 10 min, dispersion was centrifuged at 10,000 rpm for 15 min, and the supernatant was discarded. Finally, alginate coated chitosan microparticles were re-dispersed into calcium chloride (CaCl2) solution for cross linking of the alginate layer present on the surface of chitosan microparticles.
Characterization of coated and uncoated chitosan microparticles
Size, size distribution, morphology and surface charge
Shape and surface morphology of microparticles was determined by scanning electron microscopy. The particle size and zeta potential of coated and uncoated microparticles were determined using particle size analyzer (DelsaTM Nano C, Beckman Coulter, Brea, CA). The zeta potential of particles was examined using ultrapure water as solvent. These measurements were run at least three times with independent particle batches.
Entrapment efficiency and drug loading
Drug entrapment efficiency of prepared chitosan microparticles was determined by indirect method. Briefly, microparticles suspensions were centrifuged at 20,000 rpm for 30 min, and the supernatants so obtained were measured for free metoprolol succinate by using UV- spectrophotometer (UV-1700-Pharmaspec, Shimadzu, Japan) at 222 nm. Quantifications were done by using the calibration curve, and entrapment efficiency and drug loading were calculated according to following formulas:
In vitro drug release
Drug release studies from sodium alginate coated and uncoated chitosan microparticles were performed in 0.1 N HCl and PBS (pH 6.8). Chitosan microparticles with known initial amount of drug were transferred into tubular cellulose membranes (D9777, Sigma-Aldrich Co LLC USA). Membranes were then incubated in 50 ml 0.1 N HCl and phosphate buffer (pH 6.8) reservoirs at 37°C with 35 rpm agitation in shaking incubator. The amount of metoprolol succinate released from the microparticles was determined at selected time intervals through spectrophotometric investigation with measurement at 222 nm.
In vitro mucoadhesion studies
Mucoadhesive property of drug loaded microparticles was evaluated using locally assembled and modified apparatus used earlier by CitationDesai and Kumar (2004).The mucoadhesive potential of each formulation was determined by measuring a force required to detach the formulation from gastric mucosal tissue. The apparatus consists of a modified 2-arm balance; one side of which contains glass slide attached to the pan in inverted position whereas second pan was used to place the weights. A section of gastric mucosa of albino rats was fixed on each of two glass slide using cyanoacrylate tissue adhesive. First slide placed below the height adjustable pan. While another slide with mucosal section was fixed in inverted position to the underside of the same pan. Microparticles were spread between the two glass slides. A preload of 100 g was applied for complete adherence of both the slides. The preload was removed and weight was kept rising at the second pan of the balance until slides get detached from each other. Mucoadhesion force expressed as the detachment stress in dyne/cm2 was determined according to the formula given below.
Where,
m = weight added to balance in gram.
g = acceleration due to gravity in cm/s2.
A = area of the tissue exposed.
In vivo studies
Male Wistar rats (200–250 g) were used for the pharmacokinetic studies. Before experiment, animals were kept in groups of six and fed with standard diet and free access to water. Animals were kept under fasting condition overnight prior to experiment. All experimental procedures were reviewed and approved by the animal and ethics review committee.
In vivo pharmacokinetic study
The animals (18) were divided into 3 treatment groups (n = 6 in each group). First group received marketed formulation whereas second and third groups received uncoated and alginate coated chitosan microparticles. The formulations were given to the rats by anesthetizing with light ether anesthesia and formulations were given orally through oral feeding cannula. Blood samples were withdrawn by retro-orbital venous plexus puncture at predetermined time intervals. The collected blood samples were centrifuged (10,000 rpm, 15 min) and serum was collected and stored at −20°C until analysis.
Determination of drug levels from plasma
The estimation of metoprolol succinate was performed on reverse phase high performance liquid chromatography (RP-HPLC) using diode array detector (DAD). A system of WATERS (Milford, USA) composed of 515 HPLC pump as a solvent delivery system equipped with autosampler with Rheodyne injection valve with a 20 μl loop, WATERS 2998 diode array detector (DAD). Detector was set at wavelength range of 190–400 nm. Separation was performed on an Agilent Eclipse XDB-C18 column 4.6 mm × 150 mm (5 μm) ODS. Chromatographic data were recorded. The chromatographic conditions used for the estimation of metoprolol succinate in the plasma are given below in .
Table I. Chromatographic conditions used for the estimation of metoprolol succinate.
Pharmacokinetic analysis
Serum concentration profiles of metoprolol succinate in individual rats were analyzed. Relative bioavailability (F) of metoprolol succinate was calculated using the following equation.
Maximum serum concentration (Cmax) and the time to reach Cmax (Tmax) were taken directly from the observed concentration versus time profiles. Serum concentration data of metoprolol succinate obtained from rats were pooled to provide mean concentration data. The area under the concentration time curve (AUC) and the area under the first moment curve (AUMC) were calculated using the linear trapezoidal rule. Mean residence time (MRT) was determined by dividing AUMC by AUC.
Statistical analysis
All the results are expressed as mean ± standard deviation. The treated groups were compared to control by analysis of variance (ANOVA) thanks to GraphPad PRISM® software (GraphPad Software Corp., San Diego, CA). The P value < 0.05 was considered as significant.
Results and discussion
Preparation of metoprolol succinate loaded microparticles
Chitosan has been explored by various researchers for the improvement of bioavailability through oral route. However, chitosan particles suffer from some drawbacks. In the acidic condition of stomach, chitosan loses its mucoadhesive property and release drug at rapid rate (CitationLi et al. 2008, CitationPaliwal et al. 2012). Hence, residence time in the GIT is decreased. Thus, it was hypothesized that by coating with a polymer which has low solubility at acidic pH and at the same time has high mucoadhesive property, the problem of chitosan can be solved. Therefore, in the present study, sodium alginate was used as coating polymer to increase the residence time in the GIT and to control the release of drug in the GIT.
The coated microparticles of metoprolol succinate were prepared by layer by layer technique. Chitosan microparticles were prepared by ionic gelation method using sodium TPP as precipitating agent. Chitosan's ability of quick gelling on contact with polyanions is due to the formation of inter and intramolecular cross linkages mediated by polyanion. Amino groups of chitosan in acidic solution undergo protonation. TPP (multivalent anion) during the preparation process, is electrostatically attracted to the NH3+ groups in chitosan to produce ionically cross-linked chitosan microparticles.
Prepared microparticles were characterized for size and zeta potential, shape and entrapment efficiency. Size of the particles was found to be in the range of 6–29 μm depending on the ratio of chitosan to TPP used during the formation of particles. Results of the study showed that at 1:4 (TPP:chitosan) ratio, the size of particles was least (6.18 μm) and as the ratio of TPP to chitosan increased from 1:4 to 1:10, size of particles also increased (28.5 μm). This may be ascribed to agglomeration of polymer solution. The particle morphology was characterized by SEM. showed that particles were spherical in nature. The effect of drug to polymer (chitosan) ratio was observed on the entrapment efficiency and particle size of the particles. It was found that at 2:5 (drug:polymer) ratio, highest entrapment efficiency (72.76 ± 0.85%) was observed and on further increasing the proportion of drug, entrapment efficiency was decreased. This may be ascribed to the saturation of polymeric matrix by drug. From the results of the loading efficiency, it was observed that at higher drug:polymer ratio loading efficiency is not increased, which further confirms the saturation of polymeric matrix. It was also observed that with the increase in drug concentration the particle size was also increased from 5.56 μm to 18.43 μm. The effect of drug to polymer ratio is shown below in .
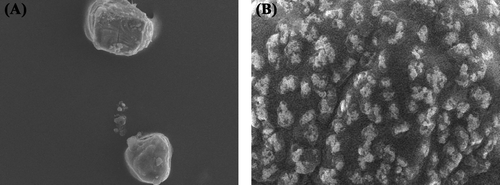
Table II. Effect of drug to polymer ratio on properties of chitosan microparticles.
The coating of alginate over the surface of chitosan microparticles was confirmed by the surface morphology analysis by SEM and by measuring zeta potential of particles. SEM images () of particles revealed a rough surface of particles that confirmed the presence of polymer coating over the surface. Further, zeta potential of the coated particles was found to be negative that was positive before coating (). The positive charge at the uncoated particles was due to chitosan. However, after coating with a polyanionic polymer (sodium alginate), the charge of particles reversed from positive to negative. These results are in accordance with study reported earlier by CitationLi et al. (2008) and CitationBagre et al. (2013). Thus, it can be concluded that surface of the chitosan microparticles have been coated with a thin layer of alginate. Further, the ratio of sodium alginate to chitosan also affects the stability of particles. It was observed that at 1:2 weight ratio of alginate to chitosan, the size of particles were least and on further increasing the amount of sodium alginate aggregation of the particles initiated. This may be due to higher viscosity of the sodium alginate solution.
Table III. Effect of alginate coating on microparticles.
In vitro mucoadhesion studies
In vitro mucoadhesion force of the coated and uncoated chitosan microparticles was determined by modified mucoadhesive force determination apparatus. The uncoated microparticles showed mucoadhesive strength of 19.89 ± 2.98 dyne/cm2 whereas alginate coated microparticles showed very high mucoadhesive strength of 25.48 ± 3.25 dyne/cm2. From the result, it can be suggested that due to high mucoadhesive property of alginate coated microparticles, the particle will remain for longer period of time in the GIT as compared to uncoated chitosan microparticles.
In vitro drug release of chitosan microparticles
The release profiles of metoprolol succinate from the coated and uncoated chitosan microparticles are shown in . Results of the studies showed that plain chitosan microparticles released more drug in the acidic environment (pH 1.2), that is, 83.4% of the total drug is released in 3 h whereas only 35.5% drug is released from alginate coated chitosan microparticles. Higher release may be ascribed to higher solubility of chitosan in the acidic pH that leads to the disruption of structural integrity of particles and release of the entrapped drug. However, after coating of the particles with sodium alginate, release of the drug in acidic pH is reduced. Coated microparticles also demonstrated extended release of the drug in intestinal pH, that is, 83.67% in 12 h as compared to 94.8% in 8 h from uncoated microparticles. The results obtained were in accordance with the study published earlier by CitationBorges et al. (2008).
In vivo pharmacokinetic study
In vivo plasma profile study in albino rats was carried out for 24 h and the effect of alginate coating on the pharmacokinetic profile of metoprolol succinate loaded chitosan microparticles was studied. depicts the plasma concentration profile after administration of marketed and microparticles (uncoated and coated) based formulations of metoprolol succinate. Results clearly indicated low bioavailability of marketed formulation that may be due to less absorption of metoprolol succinate through intestinal membrane. In contrast to this, plasma profile after administration of microparticles based formulations demonstrated higher bioavailability as compared to marketed formulation (P < 0.05). Further, coated microparticles showed higher bioavailability as compared to uncoated chitosan microparticles (P < 0.05). This might be ascribed to the slow release of the drug in the stomach and higher and sustained release of the drug at the site of absorption, that is, intestine, that increases the rate and extent of absorption. Further, mucoadhesive nature of the particles increased the release of the drug at the surface of intestinal mucosa which increases the concentration gradient that ultimately results in the increased bioavailability of metoprolol succinate. The corresponding pharmacokinetic parameters are listed in . The t1/2 of the drug was found to significantly increase from 3.04 h to 7.91 h in coated microparticles when compared to marketed formulation. Cmax of the microparticles based formulations, that is, 28.10 μg/ml for alginate coated, and 22.34 μg/ml for uncoated chitosan microparticles were found greater than that of marketed formulation, that is, 10.23 μg/ml. MRT of coated microparticles (12.15 h) was found higher than that of uncoated microparticles (9.85 h) and marketed formulation (5.29 h). This may be ascribed to the sustained release of the drug from coated microparticles as suggested earlier by CitationVenishetty et al. (2012).
Table IV. The pharmacokinetic parameters after the administration of metoprolol succinate formulations.
Results of the study clearly depicted that coating of chitosan microparticles with sodium alginate increased the residence time in the intestine and minimize the release of drug in the stomach. Moreover, the contact time in the intestine also increased because of the bioadhesive nature of both of the polymers, which ultimately results in the increased absorption and hence the bioavailability of the coated/layered microparticles. Further, the presence of chitosan increased the permeability of the endothelial membrane by opening tight junction between cells. Hence, bioavailability was found to be increased, that is, bioavailability of chitosan microparticles was 3.13 times higher than that of marketed formulation whereas it was 5.32 times higher in case of alginate coated chitosan microparticles.
Conclusion
The study aims at the preparation of sodium alginate coated chitosan microparticles to increase the bioavailability of metoprolol succinate. Chitosan microparticles were prepared by ionic gelation method and further used for coating. Release study showed that coated microparticles release drug at sustained rate which help in the absorption of drug through intestinal mucosa. Further, in vivo studies resulted in increased bioavailability of the metoprolol succinate which confirmed the potential of developed carrier systems. Thus, it can be concluded that these carriers might be a good carrier for the delivery of antihypertensive drug for better therapeutic efficacy.
Declaration of interest
The authors report no declarations of interest. The authors alone are responsible for the content and writing of the paper.
References
- Bagre AP, Jain K, Jain NK. 2013. Alginate coated chitosan core shell nanoparticles for oral delivery of enoxaparin: In vitro and in vivo assessment. Int J Pharm. 456:31–40.
- Boddupalli BM, Mohammed ZN, Nath RA, Banji D. 2010. Mucoadhesive drug delivery systemml: an overview. J Adv Pharm Technol Res. 1: 381–387.
- Borges O, Silva M, de Sousa A, Borchard G, Junginger HE, Cordeiro-da-Silva A. 2008. Alginate coated chitosan nanoparticles are an effective subcutaneousadjuvant for hepatitis B surface antigen. Int Immunopharmacol. 8:1773–1780.
- Brain BH, Robert JL. 1996. Catecholamines, sympathomimetic drugs and adrenergic receptor. In: Hardman JG, Limbird LE, Eds. Goodman and Gilman's, The Pharmacological Basis of Therapeutics. New York: Mcgraw–Hill. 1760p.
- Desai KH, Kumar TP. 2004. Preparation and evaluation of a novel buccal adhesive system. AAPS PharmSciTech. 5:1–9.
- George M, Abraham TE. 2006. Polyionic hydrocolloids for the intestinal delivery of protein drugs: alginate and chitosan -a review. J Control Release114:1–14.
- Hagerstrom H, Edsman K, Stromme M. 2003. Low -frequency dielectric spectroscopy as a tool for studying the compatibility between pharmaceutical gels and mucus tissue. J Pharm Sci. 92:1869–1881.
- He P, Davis SS, Illum L. 1998. In vitro evaluation of the mucoadhesive properties of chitosan microspheres. Int J Pharm. 166:75–88.
- Kendall MJ, Maxwell SR, Westergren, SA. 1991. Controlled release metoprolol. Clinical pharmacokinetic and therapeutic implications. ClinPharmcokinet. 5:319–330.
- Lennard MS, Silas, JH, Freestone S, Trevethick J. 1982. Defective metabolism of metoprolol in poor hydroxylators of debrisoquine. Br J Clin Pharmacol. 14:301–303.
- Li XY, Kong XY, Shi S, Zheng XL, Guo G, Wei YQ, Qian ZY. 2008. Preparation of alginate coated chitosan microparticles for vaccine delivery. BMC Biotechnol. 8:1–11.
- Palanisamy M, Khanam J, Kumar NA, Rani C. 2009. Chitosan microspheres encapsulated with metoprolol succinate: formulation and in - vitro evaluation. Res J Pharm Technol. 2:12–19.
- Paliwal R, Paliwal SR, Agrawal GP, Vyas SP. 2012. Chitosan nanoconstructs for improved oral delivery of low molecular weight heparin: in vitro and in vivoevaluation. Int J Pharm. 422: 179–184.
- Patel H. 2010. Ionotropic gelation technique for microencapsulation of antihypertensive drug. Webmed Central Pharm Sci. 1:1–10.
- Peh KK, Wong CF. 1999. Polymeric films as vehicles for buccal delivery: swelling, mechanical, and bioadhesive properties. J Pharm Pharma Sci. 2:53.
- Venishetty VK, Chede R, Komuravelli R, Adepu L, Sistla R, Diwan PV. 2012. Design and evaluation of polymer coated carvedilol loaded solid lipid nanoparticles to improve the oral bioavailability: a novel strategy to avoid intraduodenal administration. Coll Surf B Biointerfaces. 95:1–9.
- Xing YL, Xiang YK, Shuai S, Xiu LZ, Gang G, Yu QW, Zhi YQ. 2008. Preparation of sodium alginate coated chitosan microparticles, Preparation of alginate coated chitosan microparticles for vaccine delivery. BMC Biotechnol. 8:1–11.