Abstract
A gastro-retentive capsule has been prepared which is retained in the stomach for a period of 24h, providing a vehicle for the controlled delivery to the upper intestines. These “gastro cocoons” can resist passage through the sphincter of the stomach, and can retain a high drug payload (30%). They are made from oppositely charged polyelectrolytes and can swell to twice their initial volume. They are strong and also can resist 550 N of compressive force. They are based on filled pharmaceutical capsules which are visible to X-rays. Using ambroxol hydrochloride as a model drug linear, zero-order, release curves were obtained.
Introduction
The controlled delivery of existing, and novel, drugs is used in the gastrointestinal (GI) tract in order to avoid side effects linked with higher doses and to provide a more effective, patient-friendly therapy. The majority of work carried out to date involves the controlled released drug delivery in once-daily dosage forms (Chen et al. 2013, Kornmann et al. 2011, Donatucci et al. 2011). In these examples the major part of the drug is absorbed during its approximately 24 h of residence time in the colon (Wei et al. 2013). The time in the stomach and the upper intestines is, typically, 2 h though highly variable between individuals as well as function of the food content of the stomach (Giri et al. 2013).
The latest generation of new chemical entities (NCE) is primarily produced via biotechnology, with two-thirds of these drugs no longer absorbed in the colon. This has created the need for a significantly prolonged residence time of the drug in the stomach of the upper intestinal tract. To maintain the market of once-daily dosage for the NCE, new controlled drug delivery systems for gastro-retentive release are required.
The need to focus on gastro-retentive drug delivery systems and a device which can release drugs over a considerably prolonged period in a close to uniform manner has been extensively noted (Moes 1993). Many researchers believe the delivery vehicle should be retained in the stomach based on its size. There are many publications, and even products which have been tested clinically, that are based on the concept of muco adhesion (Mohana Raghava Srivalli et al. 2013, Ali et al. 2014, Laffleur 2014, Iqbal et al. 2012). This not been proven to work due to the very rapid renewal of the mucus tissue of the stomach, thus providing too short a residence time (Solomonidou et al. 2001). Other systems described focus on unfolding of drug-coated sheets with the result that the sheets do not biodegrade and may not leave the stomach. For example there are technologies described for the production of such “folded” capsules which can subsequently expand, though these do not discuss drug delivery. One method is based on unfolding and releasing of macromolecules (Verma et al. 2014).
Some recent disclosures describe extended release tablets intended for upper GI absorption involving a polymer-coated solid tablet (Yang 2013). Others describe a gastro-retentive floating tablet where gas is liberated, rendering the tablet too large to pass the pyloric sphincter (Gharti et al. 2012, Rao and Lakshmi 2014). Some technologies describe the use of gelatin capsules which can expand in the stomach and are used as diagnostic tools. More recently drug delivery systems based on burst release of valsartan followed by a gastro-retentive swollen portion including alginates and polyacrylates have been described (Kshirsagar et al. 2011). Certain investigators have also developed combinations of some of the previously described mechanisms for gastro retention such as adhesion and floatation (Umamaheswari et al. 2002). Indeed, the floating and effervescent systems have been widely described also in the scientific literature. Most of these systems involve polyelectrolytes. The concept of complexing polymers to form an insoluble, though temporally degradable matrix, has not been described and will be the objective of the present work.
Some description of gastro-retentive systems where drugs of certain polarity can be retained has been disclosed over the past several years. For example glycerol monooleate, a surface active agent, with polyethylene glycol and stearic acid could modulate the release of some model drugs such as diazepam in floating systems (Kumar et al. 2004). The reference basically notes that the chemistry of the retentive agent has to be tuned to the polarity of the drug, which would not be the case if one used a degradable swollen complex. Mixing of gums and cellulose-based material with cross-linked polyacrylate provided tablet that floats and provides zero-order release in dogs over 24 h periods (Patel et al. 2009). This tablet showed no signs of change in physical appearance and, therefore was not degrading.
For drugs with poor gastric solubility self–micro- emulsifying delivery systems have been described where a drug is enclosed in a polymer matrix. The concept is described though neither tested nor shown to breakdown or change the drug delivery (Patel and Vavia 2010). Indeed, while the idea of polyelectrolyte complexation is well known, for over half a century, most literature uses polyanion and polycation microcapsules to demonstrate controlled release in laboratory experiments though without any evidence that gastro retention is possible, nor in sizes of capsules which could, reasonably, be retained. Alginate beads, a complex between a polyanion and salt, have been studied in gastro retention (Singh et al. 2010) though the liquefaction of alginate is sensitive to certain compounds (e.g., citrate) and an alginate bead would degrade irregularly depending on food intake and pH compared with a polyanion complexed with a polycation.
Liposomes have been used, with alternative polyanion and polycation coatings to form so-called onion micro- or nano-sized capsules, though there is no evidence that these have been applied for gastro retention (Gao et al. 2011, Rajput et al. 2010). The scientific literature has many examples where natural products such as gums or starches are used, sometimes with viscosifiers such as swelling polyacrylates (Vilivalum et al. 2000). However, there does not seem to be any systems which involve oppositely charged polymers which swell in the stomach, for an insoluble complex and subsequently degrade. Further, there seems to be very little research on synthetic polymers, though certainly many of these are approved for food and pharmaceutical applications. Indeed, the main academic thrust seems to be based on using polymers, mostly natural though sometimes in combination with synthetics, to produce gas and make retention based on floatation. Polyelectrolyte complexation using retention based on size seems not to have been elaborated. Furthermore, to the authors' knowledge, no description has been identified which can increase the absorption of a poorly absorbed drug, such as those being highly water soluble, in the upper GI.
The present study is aimed at controlling residence times in the stomach. Herein we will describe a gastro-retention system where the volume of the device prevents passing through the sphincter. Specifically, a solid conventional capsule based on HPMC or gelatin (Cole et al. 2002, Curatolo et al. 2011, Bussemer et al. 2003, Karataş and Bekmezci 2013, Tyagi et al. 2013) is a vehicle for the mix of drug and excipients as the materials (polymers). These swell when contacted with gastric fluid and unfold for the controlled creation of a gastro-retentive “cocoon”.
Experimental
Materials employed
Polyelectrolytes were prepared by free radical polymerization, as detailed in Pantchev and Hunkeler (2004). Briefly, acrylamide (AAM) solutions with either acrylic acid (AA) or dimethlyaminoethylacrylate methyl chloride (DMAEA-MQ) were prepared via inverse-suspension polymerization with the final product isolated by precipitation using acetone (Acros Organics, 99+%). Polymers with various compositions were evaluated as summarized in . Polymers, once produced, were sieved so that an estimate of the size distribution could be obtained.
Table I. Characteristics of polymers synthesized.
Cocoon preparation
Cocoons were prepared by filling gelatin or HPMC capsules (size 5 to 1000) manually with a precise amount of pre-mixed polymer powder. The size of the polymer powders were estimated prior to their application via optical microscopy (Swift, 100 × magnification), with one of the experimental series designed to evaluate if the particle size influenced the swelling kinetics and ultimate cocoon size. Two granulometries (500 and 900 μm) were compared to a standard, non-sieved, particle size distribution.
Cocoon testing
Simulated gastric fluid was prepared as a solution of 0.1N HCl, made from 37% hydrochloric acid (Fluka, > 36.5%) in deionized water (conductivity below 0.6 μS).
Cocoons were placed into 200 mL of simulated gastric fluid (in a 400 mL beaker). A series of 12 beakers were thermostated to 37 ± 0.1°C in a VWR Grant model bath. Cocoons were photographed periodically (initially every 15 min for 3 h, followed by hourly observations for 8 h and then a final measurement at 16 and 24 h). The size of the cocoon was estimated and, for some experiments when the cocoon formation was reasonable (i.e. the polymers were not dissolving) cocoons were withdrawn, weighed and no longer used.
In order to simulate the effect of a payload, cocoons were filled with coated ambroxol hydrochloride pellets (size 500 μm, 50 mg drug payload). These were selected based on the ease of detection, for example using liquid chromatography.
Polymer characterization
Polymers were characterized by static light scattering with the results shown in . The hydrodynamic diameters were measured using Dynamic Light scattering (DLS) (Zeta SIZER Nano ZS, Malvern Instr. UK). This technique is suited for size measurement of nanoparticles, including macromolecules. Light scattered by the particles is acquired at 173 (backscattering) and the fluctuations of the intensity of the scattered light, resulting from the Brownian motion of the particles, is used to construct the correlation function from which the size distribution of the macromolecules is extracted using CONTIN method. The particle size has been estimated by sieving using an Endecott Sieve Shaker (London, UK).
Cocoon characterization
The mechanical properties of swollen cocoons were evaluated using a TA-XT2 Texture Analyzer (Stable Micro Systems, Godalming, UK) equipped with a force transducer of 1 mN resolution running Texture Exponent 32 software. Settings were identical for all capsules. AD40 problem (50 mm) with a compression speed of 8 mm/s and a compression height of 8 mm was applied. Ten compression cycles were applied per run with a residual compression space of 2 mm. Both HPMC and gelatin capsules were tested (Sizes 0, 1, and 5) at swelling times of 30, 60, 120, and 240 min (in 0.1 N HCl at 37°C). In addition to measuring the force under compression, the number of cycles needed for partial rupture (loss of integrity of the capsule) or full rupture were also recorded. As an example, a size 1 HPMC capsule, swollen for 30 min, partially ruptured in 5 cycles and completely ruptured in 10 cycles.
Cocoons of different sizes and chemistries were x-rayed to determine their potential visibility. Specifically, Sizes 0, 1, and 5 gelatin capsules (un and swollen for 2h in 0.1 N HCL at 37°C) were tested using a General Electric MSI.1250 IV x-ray apparatus.
Control release experiments
The cocoons were formed by pre-mixing the polymer or polymers with the controlled release pellets at total ratio of 70 wt% polymer and 30 wt% pellets (ambroxol hydrochloride pellets) as described above. This mixture was then placed into a Size 0 HPMC pharmaceutical capsule.
The capsule was placed for 24 h in a Sotax AT7 Smart (Allschwil, Switzerland) dissolution tester which permits the egress of a drug to be quantified as a function of time (Pharma Centrum, University of Basel). In the dissolution tester capsules were held in a 2 × 2 cm stainless steel basket which rotates in simulated gastric fluid (0.1N HCl). Samples of the supernatant were withdrawn automatically, as a function of time, and injected into an HPLC (Ultrospec 3100pro; Amersham Biosciences, New Jersey). The detection was using UV at 306 nm where the ambroxol hydrochloride peak has a strong signal. The system was operated with Dissocontrol V0.04 software (University of Basel, Division of Pharmaceutical Technology, Basel, Switzerland).
Animal experiments
Two dogs were used (mongrel females, 9–12 months old). Each were given three gelatin cocoons (Size 1 or 0) and sacrificed after 4 h. The dogs were being used for another experiment. The stomach was opened in order to explant the cocoon.
Results
Evaluation of the effect of polymer chemistry and stoichiometry
Polymers of various charges were investigated (). The combination of sodium acrylate anionic polymers and quaternary ammonium cationics is seen to lead to swellable, stable, cocoons. Specifically, the data indicate that anionic polymers with 30 wt % charge (X0) and 40 wt % charge (Y0) were provided capsules with good mechanical resistance with adequate swelling both of which will be quantified in a subsequent section.
Normally polyelectrolytes complexes form between a flexible polymer, such as the polyacrylamide-co-sodium acrylate and one more rigid in nature with permanent charges (e.g. polyacrylamide-co-DMAEM-MQ) (Prokop et al. 1988). This is seen in as the X0, with approximately 24 mol% charge (30 wt%) complexes very well with a polycation with 51 mol% (70 weight%) charge (CHMW). The slight imbalance in stoichiometry between the polyanion and polycation provides for a highly swellable cocoon as a completely tight symplex often results in a precipitate or even soluble molecule, neither of which would be desirable. When the polycation had lower charges (FHMW: 23 wt% and GHMW 13 mol%) cocoons could still be formed though they became mechanically weak, lose their total integrity, and decompose rapidly. They also gave lower swelling extents.
Table II. Summary of the evaluation of polymer chemistry on cocoon formation.
Another reason why the polyanion has a lower charge than the polycation is that polyelectrolyte complexation often requires a secondary adhesion force to supplement the Coulombic binding. The high AAM level in, for example, X0 (76 mol%) permits for hydrogen bonding (Prokop et al. 1988). Overall the optimal chemistry was found to be an equimass mixture of CHMW and X0. Providing for an excess of either the polycation or polyanion did not result in cocoon formation ().
Swelling
shows the swelling behavior of some CHMW-X0 cocoons of various sizes. The initial integrity of the floating capsules (a) soon gives way to either swelling or dissolution, the latter clearly undesirable (b). After 16h and 47m some cocoons are seen to remain clearly visible (c). Ideally, one would like to see a large, swollen cocoon in a clear solution, as the presence of turbidity indicates that some polymer was lost due to incomplete complexation. All capsules, with the exception of the Size 00 HPMC were seen to give acceptable cocoon formation.
Figure 1. Photograph of CHMW-X0 capsules of various sizes in 0.1N HCl, at 37°C. The time is indicated by the clock (h:m:s). Upper left: Gelatin 1; Upper right: Gelatin 00; Lower left: HPMC 00; Lower middle: Gelatin 000; Lower right: Gelatin-0EL CS. (a) Outset of the experiment, (b): after 31m, (c) after 16h47m.
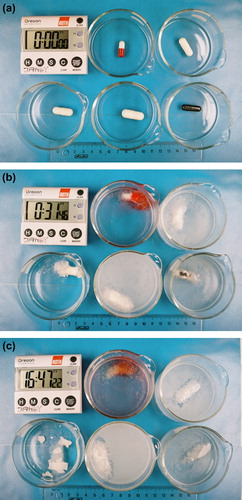
shows swelling behavior of various capsules over time. After 1h all capsules increased their volume by at least 200% (a), with the Size 1 HPMC providing the largest capsules after 5h. The Size 000 Gelatin capsules provide the highest weight gain, while the Size 1 HPMC capsules show the most durability, with 70% of their maximum weight remaining after 3h. All capsules increased weight at least four-fold (b).
Figure 2. Swelling behavior of several capsules over time, (a) percentage volume increase and (b) weight gain.
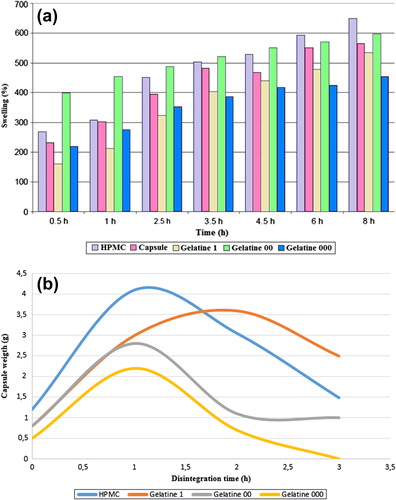
Overall, either the HPMC or the gelatin capsule can be employed in producing cocoons, with Sizes between 1 and 0. Sizes 2–5 produced cocoons that were likely too small to be retained in the stomach while the larger cocoons would be more difficult from a patient clinical perspective and offered no advantage in terms of physical properties. This will be discussed further in the in vivo section of this publication.
Effect of drug loading on properties
summarizes behavior of capsules loaded with 50 mg(cellets of 500 μm containing 50 mg of ambroxol hydrochloride and coated with hydroxypropyl methylcellulose to provide controlled release properties). The swelling extent of capsules with drug levels of up to 30% mimicked the polymer-only cocoons, indicating that a reasonable drug loading could be obtained.
Table III. Summary of the effect of payload on swollen cocoon properties.
Compression testing
reveals that as the capsules swell their mechanical resistance diminishes. This is true for both chemistries evaluated (gelatin, HPMC). Initially all capsules withstood between 700 and 1300 N of force, with the gelatin having approximately 10% more mechanical resistance. The swollen cocoons from Size 0 capsules still had good resistance even after 4 h. illustrates the effect of particle size of the initial polymers on mechanical resistance. As the particles swell, those made with 900 μm polymers tended to withstand degradation more. Specifically, after 240 min, cocoons made from 500 μm polymers still had resisted 550 N of compressive forces, while those from 900 μm particles could only support 240 N. This shows that the optimal packing in the capsule is obtained with polyelectrolytes of 500 micron, the bigger one leaving too much space inbetween them. However, for smaller capsules the cocoon was less sensitive to the polymer particle size and mechanical behavior was similar for all sizes (190–260 N after 240 min).
Figure 4. Mechanical resistance to compression as a function of the particle size of the polymers used to fill the capsules.
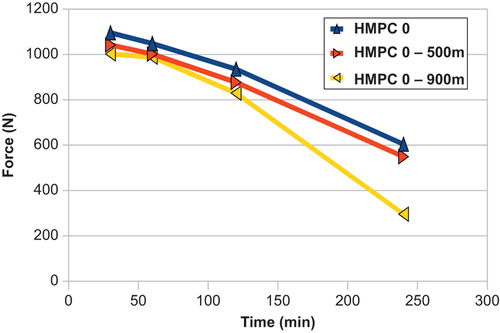
shows the effect of compression cycles on the rupture of a typical capsule. In this particular case a Size 1 HPMC capsule is seen to begin to rupture after five cycles (a) and be completely breached after 10 cycles (b).
Time release
shows time release experiments on coated ambroxol hydrochloride pellets either individually or in cocoons over a 24-h period. Two different gastro cocoons were tested:
Figure 6. Controlled release experiments of ambroxol hydrochloride controlled released pellets alone and in HPMC size 00 capsules.
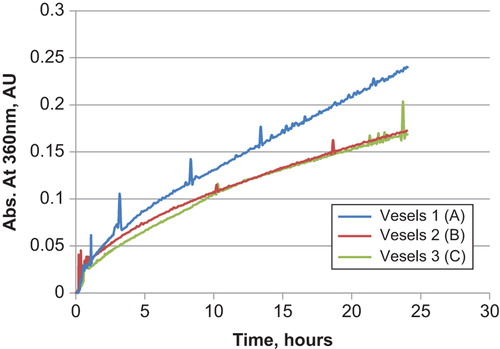
HPMC Size 0 capsule filled a polymer containing an anionic polymer with 40 wt% charge (Y0) and a cationic with 80 wt% charge (BHMW), at equimass. Vessels 2 and 3 are repeat studies.
HPMC Size 0 capsule filled with an anionic polymer with 30 wt% charge (X0) and a cationic polymer with 70 wt% charge (CHMW) at equimass.
A single polymer system based on a cationic polymer with 40% charge (FHMW).
Linear, so-called zero-order, release profiles were obtained in all cases. The release profiles are virtually identical indicating that the cocoon is not interfering in drug delivery. Furthermore, one can observe that the various chemistries employed, including those with an imbalance of cationic and anionic polymers, do not retain the drug and that the cocoon is a delivery vehicle without changing the release kinetics.
In-vitro and in-vivo imaging
Capsules were x-rayed, both dry and swollen, to evaluate whether this technique could be used to track capsules in animal experiments. It was determined that all but the smallest, Size 5, gelatin capsules were visible. shows a relatively clear image of a Size 1 capsule, which reasonable from the point of view of ingestion. While the image is blurry, one can identify the structure and this would be sufficient to demonstrate gastro retention, as discussed subsequently the in vivo experiments.
In vivo experiment was carried out using female mongrel dogs. In both animals all three capsules were retrieved and looked intact 4 h after ingestion ().
Conclusions
Cocoons can be formed, by polyelectrolyte complexation, which swell rapidly and extensively. These are likely to provide gastric retention in humans (tested only in the canine model) owing the physical size of the swollen polymer gel, which would be too large to pass the sphincter of the stomach. Capsules made from Size 1 or 0 HPMC or gelatin capsules had good mechanical properties, with compressive resistances of over 200 N after 4 h.
When loaded with ambroxol hydrochloride a zero-order release was observed. Therefore it appears that the combination of oppositely charged polyelectrolytes, in powder form, in a typical pharmaceutical capsule (HPMC) is able to swell rapidly and grow into a stable matrix which is mechanically durable. This provides a protective, degradable envelope which does not hinder drug delivery and is likely to be flushed from the stomach after 48–72 h as the symplex slowly dissolves. In the meantime, the polymer retains the drug and prevents it from passing into the GI tract, providing for gastro retention. When the drug is suitably coated for controlled release, which is now a commonly available technology, a uniform delivery to the upper colon should be achievable.
Acknowledgments
The authors would like to thank Klaus Eichler, for his work on the project since the outset as well as Diogo Figueiredo for his assistance in the library as well as with graphics. We would also like to thank Dr. Redouan Mahou of the EPFL (Lausanne) for providing access to the mechanical testing equipment, Peter Langguth, Pharmaceutical Technology & Biopharmaceutics Institut, Johannes Gutenberg-University Mainz, Germany, for his work on the swelling of the capsules, and Prof. Gra a Rasteiro and Ms. Maria Jo o Travassos, both of the Department of Chemical Engineering, University of Coimbra, for the light scattering measurements.
Control-release experiments were carried out at the University of Basel's Pharma Centrum courtesy of Dr. Maxim Puchkov. X-ray testing as well as the canine studies were graciously carried out my Prof. Farzaneh Sharifi Aghdas and her team at the Shahid Beheshti University of Medical Sciences (Tehran). The animal experiments were carried out by Mohammad Naji of the Urology and Nephrology Research Center (UNRC), Shahid Beheshti University of Medical Sciences, Tehran, Iran. The animal facility was located at the Department of Clinical Sciences, Faculty of Veterinary Medicine, University of Tehran, Tehran, Iran.
Declaration of interest
The authors report no declarations of interest. The authors alone are responsible for the content and writing of the paper.
References
- Ali MS, Pandit V, Jain M, Dhar KL. 2014. Mucoadhesive microparticulate drug delivery system of curcumin against Helicobacter pylori infection: Design, development and optimization. J Adv Pharm Technol Res. 5: 48–56.
- Bussemer T, Dashevsky A, Bodmeier R. 2003. A pulsatile drug delivery system based on rupturable coated hard gelatin capsules. J Control Release. 93:331–339.
- Chen C, Han CH, Sweeney M, Cowles VE. 2013. Pharmacokinetics, efficacy, and tolerability of a once-daily gastroretentive dosage Form of gabapentin for the treatment of postherpetic neuralgia. J Pharm Sci. 102:1155–1164.
- Cole ET, Scott RA, Connor AL, Wilding IR, Petereit HU, Schminke C, et al. 2002. Enteric coated HPMC capsules designed to achieve intestinal targeting. Int J Pharm. 231:83–95.
- Curatolo W, Liu P, Johnson BA, Hausberger A, Quan E, Vendola T, et al. 2011. Effects of food on a gastrically degraded drug: azithromycin fast-dissolving gelatin capsules and HPMC capsules. Pharm Res. 28:1531–1539.
- Donatucci CF, Brock GB, Goldfischer ER, Pommerville PJ, Elion-Mboussa A, Kissel JD, Viktrup L. 2011. Tadalafil administered once daily for lower urinary tract symptoms secondary to benign prostatic hyperplasia: a 1-year, open-label extension study. BJU Int. 107:1110–1116.
- Gao J, Reibetanz U, Venkatraman S, Neu B. 2011. Biofunctionalization of polyelectrolyte microcapsules with biotinylated polyethylene glycol-grafted liposomes. Macromol Biosci. 11:1079–1087.
- Gharti K, Thapa P, Budhathoki U, Bhargava A. 2012. Formulation and in vitro evaluation of floating tablets of hydroxypropyl methylcellulose and polyethylene oxide using ranitidine hydrochloride as a model drug. J Young Pharm. 4:201–208.
- Giri TK, Verma S, Alexander A, Ajazuddin, Badwaik H, Tripathy M, Tripathi DK. 2013. Crosslinked biodegradable alginate hydrogel floating beads for stomach site specific controlled delivery of metronidazole. Farmacia. 61:533–550.
- Iqbal J, Shahnaz G, Dünnhaupt S, Müller C, Hintzen F, Bernkop-Schnürch A. 2012. Preactivated thiomers as mucoadhesive polymers for drug delivery. Biomaterials. 33:1528–1535.
- Karataş A, Bekmezci S. 2013. Evaluation and enhancement of physical stability of semi-solid dispersions containing piroxicam into hard gelatin capsules. Acta Pol Pharm. 70:883–897.
- Kornmann O, Dahl R, Centanni S, Dogra A, Owen R, Lassen C, Kramer B. 2011. Once-daily indacaterol versus twice-daily salmeterol for COPD: a placebo-controlled comparison. Eur Respir J. 37:273–279.
- Kshirsagar SJ, Patil SV, Bhalekar MR. 2011. Statistical optimization of floating pulsatile drug delivery system for chronotherapy of hypertension. Int J Pharm Investig. 1: 207–213.
- Kumar MK, Shah MH, Ketkar A, Mahadik KR, Paradkar A. 2004. Effect of drug solubility and different excipients on floating behaviour and release from glyceryl monooleate matrices. Int J Pharm. 272:151–160.
- Laffleur F. 2014. Mucoadhesive polymers for buccal drug delivery. Drug Dev Ind Pharm. 40:591–598.
- Moes AJ. 1993. Floating delivery and other potential gastric retaining systems, current status on targeted drug delivery to the gastrointestinal tract. Capsugel Library. 97–112.
- Mohana Raghava Srivalli K, Lakshmi PK, Balasubramaniam J. 2013. Design of a novel bilayered gastric mucoadhesive system for localized and unidirectional release of lamotrigine. Saudi Pharm J. 21:45–52.
- Pantchev I, Hunkeler D. 2004. Influence of quaternary ammonium salt addition on the surface activity of polyacrylic dispersants. J Appl Polym Sci. 92:3736.
- Patel A, Modasiya M, Shah D, Patel V. 2009. Development and in vivo floating behavior of verapamil HCl intragastric floating tablets. AAPS PharmSciTech. 10:310–315.
- Patel A, Vavia P. 2010. SMEDDS incorporated polymer matrix: a floating dosage form solution for drugs with poor gastric solubility. Pharm Tech Eu. 22:1–5.
- Prokop A, Hunkeler D, Powers AC, Whitesell RR, Wang TG. 1988. Water Soluble Polymers for Immunoisolation II: Evaluation of Multicomponent Microencapsulation Systems. Adv Polym Sci 136:54.
- Rajput G, Majmudar F, Patel J, Thakor R, Rajgor NB. 2010. Stomach-specific mucoadhesive microsphere as a controlled drug delivery system. Sys Rev Pharm. 1:70–78.
- Rao KR, Lakshmi KR. Design, development and evaluation of clopidogrel bisulfate floating tablets. Int J Pharm Investig. 2014 4:19–26.
- Singh B, Sharma V, Chauhan D. 2010. Gastroretentive floating sterculia–alginate beads for use in antiulcer drug delivery. Chem Eng Res Design. 88:997–1012.
- Solomonidou D, Cremer K, Krumme M, Kreuter J. 2001. Effect of carbomer concentration and degree of neutralization on the mucoadhesive properties of polymer films. J Biomater Sci Polym Ed. 12:1191–1205.
- Tyagi VK, Singh D, Pathak K. 2013. Semisolid matrix-filled hard gelatin capsules for rapid dissolution of amlodipine besilate: Development and assessment. J Adv Pharm Technol Res. 4:42–49.
- Umamaheswari RB, Jain S, Tripathi PK, Agrawal GP, Jain NK. 2002. Floating bioadhesive microspheres containing acetohydroxamic acid for clearance of Helicobacter pylori. Drug Deliv. 9: 223–231.
- Verma S, Nagpal K, Singh SK, Mishra DN. 2014. Unfolding type gastroretentive film of Cinnarizine based on ethyl cellulose and hydroxypropylmethyl cellulose. Int J Biol Macromol. 64: 347–352.
- Vilivalum VD, Illum L, Iqbal K. 2000. Starch capsules: an alternative system for oral drug delivery. Pharm Sci Tech Today. 3:64–69.
- Wei YM, Xue ZK, Wang P, Zhao L. 2013. Formulation and pharmacokinetic evaluation of once-daily sustained-released system of nifedipine with solid dispersion and coating techniques. Arch Pharm Res 36:864–873.
- Yang Y. 2013. In vitro bioequivalence approach for a locally acting gastrointestinal drug: lanthanum carbonate. Mol Pharm. 10: 544–550.