Abstract
Context and objective: The aim of the present research was to fabricate triamcinolone acetonide (TA)-Eudragit® RS100 nanostructures using the electrospraying method. Materials and methods: The physicochemical properties of the electrosprayed formulations as well as drug release patterns were assessed. The particle size and morphology were evaluated using scanning electron microscopy. X-ray crystallography and differential scanning calorimetry were also conducted to investigate the crystallinity and polymorphic alterations of the drug in the formulations. Probable chemical interactions between the drug and the carrier during the preparation process were analyzed using FT-IR spectroscopy. The drug release kinetic was also considered to predict the release mechanism. Results and discussion: Increasing the concentration of injected polymer solution resulted in the formation of more fibers and fewer beads, with the particle diameter ranging from 60 nm to a few micrometers based on the drug: polymer ratio. The drug crystallinity was notably decreased during the electrospraying process; however, no interaction between drug and polymer was detected. The electrosprayed formulations with 1:10 drug: polymer ratio showed an almost similar drug release rate compared to the pure drug, while those with 1:5 ratio revealed slower release profiles. The release data were best fitted to the Weibull model, so that the corresponding shape factor values of the Weibull model were less than 0.75, indicating the diffusion controlled release mechanism. Conclusion: Our findings revealed that TA loaded Eudragit® RS100 nanofibers and nanobeads were properly prepared by the electrospraying method, which is a simple, surfactant-free and cost effective technique for producing drug: polymer nanostructures.
Introduction
The concept of novel drug delivery has rapidly grown and attracted the interest of scientists from different fields of science. Targeted and controlled delivery of the drugs, decreased toxicity and side effects as well as patient compliance are a few among many of the advantages of novel drug delivery systems (CitationPrisant et al. 1992, CitationReddy 2000, CitationMidhun et al. 2011). Nanocarriers, as one of the major novel drug delivery systems, have been investigated and benefited from for more than 20 years, thanks to their outstanding features such as high surface area and increased solubility (CitationKaparissides et al. 2006, CitationKocbek et al. 2006, CitationSnopczynski et al. 2009, CitationAkhgari et al. 2013). Different techniques have been developed to prepare nanoparticles including emulsification solvent evaporation (CitationJavadzadeh et al. 2010), template synthesis (CitationKrishna Sailaja et al. 2011), aerosol flow reactor (CitationEerikäinen et al. 2003), supercritical fluid, nanoprecipitation and electrospraying (CitationRao and Geckeler 2011, CitationSailaja et al. 2011, CitationSode et al. 2013). Electrospraying is rapidly growing as a popular technology for the fabrication of submicron polymeric structures containing different molecules. In comparison with traditional production techniques, electrospraying has the potential to fabricate reproducible particles with regulated particle size distribution and morphology (CitationBock et al. 2012). The device used for the electrospraying of polymers is simply composed of three main parts: a high voltage DC power supplier, syringe and collector. In brief, this method takes advantage of a high voltage electrical field (approximately 10–30 KV) to induce electrical charge on the surface of a polymer solution. The dropped solution would then form a liquid jet due to electrostatic repulsion forces and fly towards the grounded collector screen. The solvent evaporates by its movement to the collector, and depending on the solution's viscosity, nanofibers or nanobeads take shape on the collector (CitationDoshi and Reneker 1995, CitationCui et al. 2006, CitationAdibkia et al. 2007b, CitationYu et al. 2009). It was reported that the characteristics of the electrosprayed particles were also related to other parameters like drug: polymer ratio, flow rate, voltage and the distance between needle and collector (CitationPham et al. 2006, CitationMartins et al. 2008, CitationBhardwaj and Kundu 2010). Therefore, aside from the simplicity and cost effectiveness of this technique, electrospraying also has the advantage of managing the morphology and physicochemical properties of the product by optimizing the operating conditions, thus making it applicable on an industrial scale. Electrospraying and electrospinning have already been used for biomedicine, optical electronics (CitationWang et al. 2002), tissue engineering scaffolds (CitationLuu et al. 2003), protective clothing (CitationSubbiah et al. 2005) and filtration (CitationRajesh and Natarajan 2009). In contribution to the pharmaceutical industry, this method was applied to prepare nanoformulations of diclofenac sodium(CitationShen et al. 2011), ketoprofen (CitationYu et al. 2013), salicylic acid (CitationNguyen et al. 2012), lidocaine hydrochloride (CitationIgnatova et al. 2013) ibuprofen (CitationPires et al. 2013, CitationShi et al. 2013) naproxen (CitationCanbolat et al. 2014) and doxycycline (CitationChaturvedi et al. 2013). Eudragit® RS100 is a cationic copolymer of poly (ethylacrylate, methyl–methacrylate and chlorotrimethyl– ammonioethyl methacrylate). This polymer is almost insoluble at physiologic pH; however, its hydrophilic characteristics make the polymer become a little swollen. The presence of quaternary ammonium groups accounts for the polycationic nature of Eudragit® RS100, making it a suitable carrier to encapsulate the anionic drugs and also attach to the negatively charged cells (CitationAdibkia et al. 2007a, CitationLoveymi et al. 2012). Thus, Eudragit®RS100 seems to be an appropriate polymer for prolonged and localized delivery of a desired medicine to some physiologic fluids. Eudragit® RS100 has been previously applied in the delivery of anti-inflammatory drugs to the eye (CitationPignatello et al. 2002b, CitationAdibkia et al. 2007b, Citation2011). Triamcinolone acetonide (TA) is a synthetic corticosteroid with a potent anti-inflammatory effect. It is administered as an injectable suspension for the treatment of different inflammatory conditions including arthritic disorders, macular edema and diabetic retinopathy (CitationBeer et al. 2003, CitationMoshfeghi et al. 2003). TA nanoformulations have been prepared using emulsification/solvent diffusion (CitationSabzevari et al. 2013), high pressure homogenization (CitationAraujo et al. 2010, CitationAraújo et al. 2011), interfacial polymerization (CitationKrause et al. 1986) and o/w emulsion- solvent evaporation (CitationKadam et al. 2012); however, to the best of our knowledge TA-Eudragit® RS100 nanoparticles have not been prepared by the electrospraying method. In the present work, TA-Eudragit® RS100 nanofibers and nanobeads were formulated and the physicochemical characteristics of the nanoformulations were assessed.
Materials and methods
Materials
TA was purchased from Crystal Pharma (Spain). Eudragit® RS100 from Degussa (Darmstadt, Germany) was received as a gift from Akbarieh Co. (Tehran, Iran). All other chemicals were of analytical grade or the best grade available.
Preparation of the nanofibers and nanobeads
A custom-designed electrospraying system was used for preparation of the TA–Eudragit® RS100 electrosprayed samples. The electrospraying apparatus was equipped with a high-voltage DC power supply (Fanavaran Nano-Meghyas, Iran). The polymer and drug were co-dissolved in ethanol at room temperature with drug: polymer ratios of 1:5 and 1:10, so that the polymer solution concentrations were 10, 15 and 20% (w/v). The solutions were dropped through a 5 mL syringe attached to a circular-shaped polyethylene capillary tube with an inner diameter of 0.1 mm and an injection rate of 5 ml/h. The applied voltage was controlled in 25 kV between the spraying needle and grounded Teflon screen. The distance between the nozzle and collector was adjusted to10 cm.
Scanning electron microscopy
A scanning electron microscope (SEM; Tescan, Brno Czech) operating at 20 KV was used to characterize the shape and surface morphologies of the electrosprayed samples. Prior to examination, the samples were mounted on aluminum specimen stubs and coated with gold under argon atmosphere by means of a sputter coater.
Differential scanning calorimeter
DSC60 (Shimadzu, Japan) was used to evaluate the thermal behavior of the drug and polymer in the formulations. The accurately weighed samples (5 mg) were placed in aluminum pans and sealed. Thermograms were achieved at a scanning rate of 20°C/min, covering a temperature range from 25 up to 250°C. Aluminum oxide and indium powders were employed as reference and standard, respectively. The thermograms were recorded and analyzed via TA60 software.
X-ray powder diffraction
X-ray diffractograms of the pure drug, polymer, physical mixture and the electrosprayed formulations were obtained using an automated diffraction system (Siemens, Model D5000, Germany). Measurements were carried out under exposure of Cu Kα radiation of wavelength 1.5405A°, at 40 kV, 30 mA. The scanning rate was 0.06° min − 1, over the range of 2θ = 5–35°.
Fourier transformed infrared spectroscopy
The fourier transformed infrared spectroscopy (FT-IR) analysis was performed to verify the possibility of a chemical interaction between drug and polymer. The analysis was conducted with the FT-IR spectrophotometer (Shimadzu 43000, Shimadzu) by the KBr disk method (2 mg sample in 200 mg KBr). The scanning range was 400–4000 cm− 1 and the resolution was 2 cm− 1. The spectra were obtained by averaging 32 scans.
Dissolution study and drug release kinetic
The release rates of TA from the physical mixtures, pure drug and prepared nanoformulations with the drug: polymer ratios of 1:5 and 1:10, were determined using the USP apparatus II, paddle stirrer. The dissolution medium was 300 ml phosphate buffer (pH = 6.8) and the temperature was controlled at 37°C with continuous orbital mixing (50 rpm). All the examined formulations contained an equivalent of 20 mg of TA. At specified time intervals, 3 ml of media was taken by syringe and filtered through a cellulose acetate membrane (Whatman, UK) with the pore diameter of 20 nm. The volume removed was replaced with fresh buffer and the concentration of TA was assayed at 254 nm using a UV spectrophotometer (Shimadzu, Kyoto, Japan). The amount of TA in the samples was calculated using a calibration curve. The cumulative amount of the TA released was calculated for 8 h and plotted against time. Data obtained from in vitro release studies were fitted into nine common kinetic models including: zero order, first order, Higuchi, power law, Hixson-Crowell, Square root of mass, three seconds root of mass, the Weibull and Linear probability (CitationWagner 1969, CitationCosta and Sousa Lobo 2001, CitationBarzegar-Jalali 2008), to predict the mechanism of drug release from the prepared formulations . The squared correlation coefficient (RSQ) and percent error (PE) were presented for each model as well.
Results and discussions
Particle size and morphology
In the current study, the electrospraying process was employed to encapsulate TA, a water-insoluble drug, into Eudragit® RS100. SEM images of the prepared nanoformulations are shown in . The results of this study as well as previous works indicated that polymer solution concentration was the key factor in the morphology of the fabricated nanostructures (CitationHuang et al. 2003, CitationPham et al. 2006). In lower concentrations, the formulations were mainly composed of discrete beads and a few fibers, with the bead size varying from about 60 nm in the drug: polymer ratio of 1:5 to a few micrometers in the ratio of 1:10. Nevertheless, in higher polymer solution concentrations (20%), more fibers and fewer beads were observed. The length of the fibers was several micrometers and the fiber diameter depended on the drug: polymer ratio. Fiber diameter was about 70 nm in a drug: polymer ratio of 1:5 and increased several fold in the drug: polymer ratio of 1:10. Formation of the beads at low concentrations (10%) of polymer solution is explained by the fact that the surface tension of the liquid is stronger than its viscoelastic forces, which initiates the instability of the liquid jet and breaks the jet into separate droplets. Conversely, when the polymer concentration is high enough, the surface tension drops below the critical instability amount and so the liquid jet is preserved, resulting in the formation of the fibers (CitationYu et al. 2006). Therefore, polymer viscosity becomes the most important process parameter which affects the fiber size. Surface morphology of the electrosprayed nanostructures pointed out that larger beads were obtained in the 1:10 ratio. The concavities present on the surface of the beads were possibly due to the solvent evaporation whereas smaller beads were nearly spherical with smooth surfaces. In the case of fibers, it was noted that the formulations with drug: polymer ratio of 1:10 had smoother surfaces in comparison with the 1:5 ratio. Similar results were reported for collagen (CitationHuang et al. 2001), cellulose acetate (CitationLiu and Hsieh 2002), PLGA (CitationKim et al. 2005), and polyethylene oxide (CitationFrey and Li 2007) by other researchers.
Differential scanning calorimeter
Differential scanning calorimeter (DSC) thermograms of pure TA, Eudragit® RS100, physical mixture as well as drug loaded nanofibers and nanobeads are presented in . The large endothermic peak at about 290°C in the TA thermogram corresponded to its melting point, indicating that the intact TA was in a crystalline anhydrous state. The small endothermic peak at around 65°C represented the Eudragit® RS100 glass transition temperature. The characteristic endothermic peak in the drug thermogram disappeared in the physical mixture due to dilution effect of the polymer and/or the interaction between drug and polymer, induced by heating. Thermograms of the electrosprayed samples exhibited no clear peaks which could be explained by the decrease in drug crystallinity and/or solvation of the drug in the melted carrier and/or the heat-induced interaction between drug and polymer (CitationAdibkia et al. 2007b, CitationJavadzadeh et al. 2010). Comparable results were reported for itraconazole electrospuns (CitationVerreck et al. 2003), as well as other size reduction processes including cogrinding of gliclazide (CitationBarzegar-Jalali et al. 2010b), quasi-emulsion solvent diffusion of azithromycin and clarithromycin (CitationMohammadi et al. 2010, Citation2011), and solvent evaporation/extraction of piroxicam and naproxen (CitationAdibkia et al. 2007b, Citation2011).
X-ray powder diffraction
X-ray diffractograms of pure TA, polymer, physical mixture of drug and polymer and the prepared nanoformulations are demonstrated in . The pure drug spectrum exhibited sharp peaks at about 2°θ: 9, 14, 17 and 24 degrees that were characteristic of the crystalline structure of TA whereas the Eudragit® RS100 diffractogram contained no distinctive peaks due to its amorphous nature. The decreased intensity of the TA peaks in the physical mixture spectrum was explained by the dilution effect mentioned before. The X-ray powder diffraction (XRPD) analysis of prepared nanofibers and nanobeads revealed no clear peaks due to drug amorphization (CitationPignatello et al. 2002a, CitationAdibkia et al. 2007c). Therefore, the XRPD findings confirmed the DSC results. Similar results have previously been reported for the AZI-PLGA nanoparticles prepared by the quasi-emulsion solvent diffusion technique (CitationJasanada et al. 2002, CitationBarzegar-Jalali et al. 2010a, CitationMohammadi et al. 2010).
Fourier-transform infrared spectroscopy
Possible interactions between the drug and polymer were evaluated by an fourier-transform infrared spectroscopy (FT-IR) analysis. The FT-IR spectra of the pure drug, physical mixture and the prepared formulations are displayed in . The TA spectrum, with the empirical formula of C24H31FO6, contained characteristic bands including the bands in the range of 3650–3200 cm− 1 for the OH stretch, below 3000 cm− 1 for the C–H stretch of sp2 and sp3 carbons, at about 1700 cm− 1 for the C = O stretch, in the range of 1600–1690 cm− 1 for the C = C stretch, in the range of 1000–1300 cm− 1 for the C–O stretch of ether and at about 1050 cm− 1 for the C–F stretch. Comparing the spectra for the prepared formulations with those for the physical mixture and pure drug clarified the maintenance of major bands as well as the absence of new ones. These findings revealed the lack of any chemical interaction between the drug molecules and polymer during the electrospraying process. Similar results were reported for TA-loaded nanolipid carriers (CitationAraujo et al. 2010) and TA-PLGA microparticles (Citationda Silva-Junior et al. 2009).
Dissolution study and drug release kinetic
The drug release profiles of the pure TA, physical mixtures, as well as the prepared nanofibers and nanobeads are presented in . The release pattern for pure drug and physical mixtures exhibited no noteworthy difference. The time at which 50% of drug was released (t50%) together with Q15min and Q30min (percent drug dissolved within 15 and 30 min respectively) were also determined for all of the samples ().
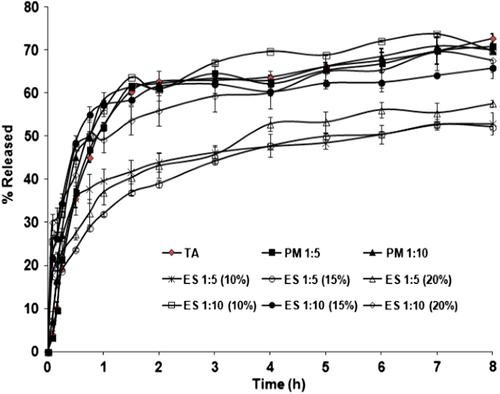
Table I. Model-independent parameters of the different samples.
The electrosprayed formulations with the drug: polymer ratio of 1:10 revealed a release rate comparable with pure TA; however, the corresponding dissolution curves were composed of a biphasic release pattern; namely, the initial burst release within the first hour and the subsequent plateau release. The biphasic release profile is a common phenomenon in many nano drug delivery systems (CitationLeo et al. 2000). The burst release could be explained by the high surface area as well as the accumulation of the drug molecules on the superficial layers of the particles, whereas the plateau phase is elucidated by the dissolution and diffusion of the drug from the inner layers (CitationYu et al. 2009, CitationMohammadi et al. 2010, CitationMidhun et al. 2011). The faster release rate in the case of 1:10 drug: polymer ratios may partially contribute to a more homogeneous and finer dispersion of drug molecules in the polymer matrix. This finding was similarly reported for diclofenac- Eudragit® RS100 nanoparticles (CitationBarzegar-Jalali et al. 2012). Contrarily, the drug: polymer ratio of 1:5 led to a slower release pattern in comparison with pure TA and physical mixtures, in which the drug entrapment in the polymer matrix was probably the dominant mechanism of drug release. Similar results were obtained for the Naproxen-Eudragit® RS100 nanoparticles (CitationAdibkia et al. 2011).
A kinetic study of drug release could be helpful in obtaining one or two physically meaningful parameters that are used for comparative purposes and in correlating release parameters with important factors such as bioavailability. In addition, a kinetic parameter can be used to assess the influence of formulation factors on the drug release (CitationBarzegar-Jalali et al. 2008). According to the RSQ and PE values (), it was concluded that the Weibull was the best fitting model. The value of the exponent β in this model is an important indicator to estimate the drug release mechanism through the polymer matrix. The values of β ≤ 0.75 specify Fickian diffusion, while β values in the range of 0.75–1 determine a combined mechanism of Fickian diffusion and swelling-controlled release (CitationAdibkia et al. 2007b). As indicated in , the resulting β values were less than 0.75, signifying that diffusion was the dominant mechanism of drug release from the electrosprayed samples.
Table II. Parameters of the different kinetic models; squared correlation coefficients (RSQ); and percent error (PE) for the formulations F1–F6.
Conclusion
The SEM results revealed that TA-Eudragit® RS100 nanobeads and nanofibers could be fabricated using the electrospraying method. However, the size and morphology of the particles depended on some parameters, specifically the concentration of the polymer solution applied, and the drug: polymer ratio, which could be optimized to produce the ideal formulation for intended applications. DSC and XRPD studies pointed out that drug crystallinity was notably decreased during the preparation process. The FT-IR spectra revealed no chemical interaction between TA and Eudragit® RS100 during the electrospraying procedure. Dissolution studies demonstrated a biphasic release profile for the formulations with drug: polymer ratio of 1:10. Slower release patterns were observed for the formulations with the drug: polymer ration of 1:5. The kinetic study suggested that the Weibull was the best fitting model and that diffusion was the dominant mechanism of drug release.
Acknowledgement
The authors would like to thank the Drug Applied Research Center, Tabriz University of Medical Sciences, Tabriz, Iran. This article is based on a thesis submitted for a Pharm D degree in Faculty of Pharmacy, Tabriz University of Medical Sciences, and Tabriz, Iran.
Declaration of interest
The authors report no declarations of interest. The authors alone are responsible for the content and writing of the paper.
References
- Adibkia K, Javadzadeh Y, Dastmalchi S, Mohammadi G, Niri FK, Alaei-Beirami M. 2011. Naproxen–Eudragit® RS100 nanoparticles: Preparation and physicochemical characterization. Colloids Surf B Biointerfaces. 83:155–159.
- Adibkia K, Omidi Y, Siahi MR, Javadzadeh AR, Barzegar-Jalali M, Barar J, et al. 2007a. Inhibition of endotoxin-induced uveitis by methylprednisolone acetate nanosuspension in rabbits. J Ocul Pharmacol Ther. 23:421–432.
- Adibkia K, Shadbad MRS, Nokhodchi A, Javadzedeh A, Barzegar-Jalali M, Barar J, et al. 2007b. Piroxicam nanoparticles for ocular delivery: physicochemical characterization and implementation in endotoxin-induced uveitis. J Drug Target. 15:407–416.
- Adibkia K, Siahi Shadbad MR, Nokhodchi A, Javadzedeh A, Barzegar-Jalali M, Barar J, et al. 2007c. Piroxicam nanoparticles for ocular delivery: physicochemical characterization and implementation in endotoxin-induced uveitis. J Drug Target. 15:407–16.
- Akhgari A, Heshmati Z, Sharif Makhmalzadeh B. 2013. Indomethacin electrospun nanofibers for colonic drug delivery: preparation and characterization. Adv Pharm Bull. 3:85–90.
- Araujo J, Gonzalez-Mira E, Egea M, Garcia M, Souto E. 2010. Optimization and physicochemical characterization of a triamcinolone acetonide-loaded NLC for ocular antiangiogenic applications. Int J Pharm. 393:168–176.
- Araújo J, Nikolic S, Egea MA, Souto EB, Garcia ML. 2011. Nanostructured lipid carriers for triamcinolone acetonide delivery to the posterior segment of the eye. Colloids Surf B Biointerfaces. 88:150–157.
- Barzegar-Jalali M. 2008. Kinetic analysis of drug release from nanoparticles. J Pharm Pharm Sci. 11:167–177.
- Barzegar-Jalali M, Adibkia K, Valizadeh H, Shadbad MR, Nokhodchi A, Omidi Y, et al. 2008. Kinetic analysis of drug release from nanoparticles. J Pharm Pharm Sci. 11:167–177.
- Barzegar-Jalali M, Alaei-Beirami M, Javadzadeh Y, Mohammadi G, Hamidi A, Andalib S, Adibkia K. 2012. Comparison of physicochemical characteristics and drug release of diclofenac sodium–eudragit® RS100 nanoparticles and solid dispersions. Powder Technol. 219:211–216.
- Barzegar-Jalali M, Valizadeh H, Nazemiyeh H, Barzegar-Jalali A, Siahi Shadbad MR, Adibkia K, Zare M. 2010a. Reciprocal powered time model for release kinetic analysis of ibuprofen solid dispersions in oleaster powder, microcrystalline cellulose and crospovidone. J Pharm Pharm Sci. 13:152–161.
- Barzegar-Jalali M, Valizadeh H, Siahi Shadbad MR, Adibkia K, Mohammadi G, Farahani A, et al. 2010b. Cogrinding as an approach to enhance dissolution rate of a poorly water-soluble drug (gliclazide). Powder Technol. 197:150–158.
- Beer PM, Bakri SJ, Singh RJ, Liu W, Peters GB III, Miller M. 2003. Intraocular concentration and pharmacokinetics of triamcinolone acetonide after a single intravitreal injection. Ophthalmol. 110: 681–686.
- Bhardwaj N, Kundu S. 2010. Electrospinning: a fascinating fiber fabrication technique. Biotechnol Adv. 28:325–347.
- Bock N, Dargaville TR, Woodruff MA. 2012. Electrospraying of polymers with therapeutic molecules: state of the art. Prog Polym Sci. 37:1510–1551.
- Canbolat MF, Celebioglu A, Uyar T. 2014. Drug delivery system based on cyclodextrin-naproxen inclusion complex incorporated in electrospun polycaprolactone nanofibers. Colloids Surf B Biointerfaces. 115:15–21.
- Chaturvedi T, Srivastava R, Srivastava A, Gupta V, Verma PK. 2013. Doxycycline poly E-caprolactone nanofibers in patients with chronic periodontitis–a clinical evaluation. J Clin Diagn Res,.7:2339.
- Costa P, Sousa Lobo JM. 2001. Modeling and comparison of dissolution profiles. Eur J Pharm Sci. 13:123–133.
- Cui W, Li X, Zhu X, Yu G, Zhou S, Weng J. 2006. Investigation of drug release and matrix degradation of electrospun poly (DL-lactide) fibers with paracetanol inoculation. Biomacromolecules. 7:1623–1629.
- da Silva-Junior AA, de Matos JR, Formariz TP, Rossanezi G, Scarpa MV, do Egito EST, de Oliveira AG. 2009. Thermal behavior and stability of biodegradable spray-dried microparticles containing triamcinolone. Int J Pharm. 368:45–55.
- Doshi J, Reneker DH. 1995. Electrospinning process and applications of electrospun fibers. J Electrostatics. 35:151–160.
- Eerikäinen H, Watanabe W, Kauppinen E, Petri Ahonen P. 2003. Aerosol flow reactor method for synthesis of drug nanoparticles. Eur J Pharm Biopharm. 55:357–360.
- Frey MW, Li L. 2007. Electrospinning and porosity measurements of nylon-6/poly (ethylene oxide) blended non-wovens. J Eng Fibers Fabrics. 2:31–37.
- Huang L, Nagapudi K, Apkarian RP, Chaikof EL. 2001. Engineered collagen-PEO nanofibers and fabrics. J Biomater Sci Polym Ed. 12:979–993.
- Huang ZM, Zhang YZ, Kotaki M, Ramakrishna S. 2003. A review on polymer nanofibers by electrospinning and their applications in nanocomposites. Compos Sci Technol. 63:2223–2253.
- Ignatova М, Rashkov I, Manolova N. 2013. Drug-loaded electrospun materials in wound-dressing applications and in local cancer treatment. Expert Opin Drug Deliv. 10:469–483.
- Jasanada MSB, Garcia IL, Mari FF. 2002. Azithromycin preparation in its noncryst alline and crystalline dihydrate forms. U.S. Patent 6, 451,990 B1.
- Javadzadeh Y, Ahadi F, Davaran S, Mohammadi G, Sabzevari A, Adibkia K. 2010. Preparation and physicochemical characterization of naproxen–PLGA nanoparticles. Colloids Surf B Biointerfaces. 81:498–502.
- Kadam RS, Tyagi P, Edelhauser HF, Kompella UB. 2012. Influence of choroidal neovascularization and biodegradable polymeric particle size on transscleral sustained delivery of triamcinolone acetonide. Int J Pharm. 434:140–147.
- Kaparissides C, Alexandridou S, Kotti K, Chaitidou S. 2006. Recent advances in novel drug delivery systems. J Nanotechnol Online. 2:1–11.
- Kim HS, Kim K, Jin HJ, Chin IJ.Morphological Characterization of Electrospun Nano-Fibrous Membranes of Biodegradable Poly (L-lactide) and Poly (lactide-co-glycolide). Macromolecular Symposia. 2005. Wiley Online Library, 145–154.
- Kocbek P, Baumgartner S, Kristl J. 2006. Preparation and evaluation of nanosuspensions for enhancing the dissolution of poorly soluble drugs. Int J Pharm. 312:179–186.
- Krause HJ, Schwarz A, Rohdewald P. 1986. Interfacial polymerization, a useful method for the preparation of polymethylcyanoacrylate nanoparticles. Drug Dev Ind Pharm. 12:527–552.
- Krishna Sailaja A, Amareshwar P, Chakravarty P. 2011. Different techniques used for the preparation of nanoparticles using natural polymers and their application. Int J Pharm Pharm Sci. 3:45–50.
- Leo E, Forni F, Bernabei MT. 2000. Surface drug removal from ibuprofen-loaded PLA microspheres. Int J Pharm. 196:1–9.
- Liu H, Hsieh YL. 2002. Ultrafine fibrous cellulose membranes from electrospinning of cellulose acetate. J Polym Sci Part B Polym Phy. 40:2119–2129.
- Loveymi BD, Jelvehgari M, Zakeri-Milani P, Valizadeh H. 2012. Design of vancomycin RS-100 nanoparticles in order to increase the intestinal permeability. Adv Pharm Bull. 2:43.
- Luu YK, Kim K, Hsiao BS, Chu B, Hadjiargyrou M. 2003. Development of a nanostructured DNA delivery scaffold via electrospinning of PLGA and PLA-PEG block copolymers. J Control Release. 89: 341–353.
- Martins A, Reis RL, Neves NM. 2008. Electrospinning: processing technique for tissue engineering scaffolding. Int Mater Rev. 53:257–274.
- Midhun B, Shalumon K, Manzoor K, Jayakumar R, Nair S, Deepthy M. 2011. Preparation of budesonide-loaded polycaprolactone nanobeads by electrospraying for controlled drug release. J Biomater Sci Polym Ed. 22:2431–2444.
- Mohammadi G, Nokhodchi A, Barzegar-Jalali M, Lotfipour F, Adibkia K, Ehyaei N, Valizadeh H. 2011. Physicochemical and anti-bacterial performance characterization of clarithromycin nanoparticles as colloidal drug delivery system. Colloids Surf B Biointerfaces. 88:39–44.
- Mohammadi G, Valizadeh H, Barzegar-Jalali M, Lotfipour F, Adibkia K, Milani M, et al. 2010. Development of azithromycin– PLGA nanoparticles: Physicochemical characterization and antibacterial effect against Salmonella typhi. Colloids Surf B Biointerfaces. 80:34–39.
- Moshfeghi DM, Kaiser PK, Scott IU, Sears JE, Benz M, Sinesterra JP, et al. 2003. Acute endophthalmitis following intravitreal triamcinolone acetonide injection. Am J Ophthalmol. 136:791–796.
- Nguyen TT, Ghosh C, Hwang SG, Chanunpanich N, Park JS. 2012. Porous core/sheath composite nanofibers fabricated by coaxial electrospinning as a potential mat for drug release system. Int J Pharm. 439:296–306.
- Pham Q, Sharma U, Mikos A. 2006. Electrospinning of polymeric nanofibers for tissue engineering applications: a review. Tissue Eng. 12:1197–1211.
- Pignatello R, Bucolo C, Ferrara P, Maltese A, Puleo A, Puglisi G. 2002a. Eudragit RS100 nanosuspensions for the ophthalmic controlled delivery of ibuprofen. Eur J Pharm Sci. 16:53–61.
- Pignatello R, Bucolo C, Ferrara P, Maltese A, Puleo A, Puglisi G. 2002b. Eudragit RS100® nanosuspensions for the ophthalmic controlled delivery of ibuprofen. Eur J Pharm Sci. 16:53–61.
- Pires LR, Guarino V, Oliveira MJ, Ribeiro CC, Barbosa MA, Ambrosio L, Pêgo AP. 2013. Ibuprofen-loaded poly (trimethylene carbonate-coϵ-caprolactone) electrospun fibres for nerve regeneration. J Tissue Eng Regen Med.
- Prisant LM, Bottini B, DiPiro JT, Carr AA. 1992. Novel drug-delivery systems for hypertension. Am J Med. 93:S45–S55.
- Rajesh KP, Natarajan TS. 2009. Electrospun polymer nanofibrous membrane for filtration. J Nanosci Nanotechnol. 9:5402–5405.
- Rao JP, Geckeler KE. 2011. Polymer nanoparticles: preparation techniques and size-control parameters. Prog Polym Sci. 36: 887–913.
- Reddy KR. 2000. Controlled-release, pegylation, liposomal formulations: new mechanisms in the delivery of injectable drugs. Ann Pharmacother. 34:915–923.
- Sabzevari A, Adibkia K, Hashemi H, Hedayatfar A, Mohsenzadeh N, Atyabi F, et al. 2013. Polymeric triamcinolone acetonide nanoparticles as a new alternative in the treatment of uveitis:In vitro and in vivo studies. Eur J Pharm Biopharm. 84:63–71.
- Sailaja AK, Amareshwar P, Chakravarty P. 2011. Different techniques used for the preparation of nanoparticles using natural polymers and their application. Int J Pharm Pharm Sci. 3:45–50.
- Shen X, Yu D, Zhu L, Branford-White C, White K, Chatterton NP. 2011. Electrospun diclofenac sodium loaded Eudragit® L 100-55 nanofibers for colon-targeted drug delivery. Int J Pharm. 408: 200–207.
- Shi Y, Wei Z, Zhao H, Liu T, Dong A, Zhang J. 2013. Electrospinning of Ibuprofen-Loaded Composite Nanofibers for Improving the Performances of Transdermal Patches. J Nanosci Nanotechno. 13: 3855–3863.
- Snopczynski T, Goralczyk K, Czaja K, Strucinski P, Hernik A, Korcz W, Ludwicki JK. 2009. [Nanotechnology–possibilities and hazards]. Rocz Panstw Zakl Hig. 60:101–111.
- Sode K, Tanaka M, Suzuki Y, Kawakami H. 2013. Nickel nanoparticle chains inside carbonized polymer nanofibers: preparation by electrospinning and ion-beam irradiation. Nanoscale. 5:8235–8241.
- Subbiah T, Bhat GS, Tock RW, Parameswaran S, Ramkumar SS. 2005. Electrospinning of nanofibers. J Appl Polym Sci. 96:557–569.
- Verreck G, Chun I, Peeters J, Rosenblatt J, Brewster ME. 2003. Preparation and characterization of nanofibers containing amorphous drug dispersions generated by electrostatic spinning. Pharm Res. 20:810–817.
- Wagner JG. 1969. Interpretation of percent dissolved-time plots derived from in vitro testing of conventional tablets and capsules. J Pharm Sci. 58:1253–1257.
- Wang X, Drew C, Lee SH, Senecal KJ, Kumar J, Samuelson LA. 2002. Electrospun nanofibrous membranes for highly sensitive optical sensors. Nano Lett. 2:1273–1275.
- Yu DG, Li XY, Wang X, Chian W, Liao YZ, Li Y. 2013. Zero-order drug release cellulose acetate nanofibers prepared using coaxial electrospinning. Cellulose. 20:379–389.
- Yu DG, Zhu LM, White K, Branford-White C. 2009. Electrospun nanofiber-based drug delivery systems. Health (N. Y.). 1:67–75.
- Yu JH, Fridrikh SV, Rutledge GC. 2006. The role of elasticity in the formation of electrospun fibers. Polymer. 47:4789–4797.