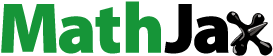
Abstract
The construction of an L-lysine biosensor on ammonium-selective poly(vinylchloride) (PVC) membrane electrode is described in this study. The construction procedure occurs in two stages: (I) the preparation of ammonium-selective poly(vinylchloride) (PVC) membrane electrode and (II) the chemical immobilization of lysine oxidase on this ammonium-selective electrode by using glutaraldehyde. The ammonium ions produced after enzymatic reaction were determined potentiometrically. The sensitivity of the lysine biosensor against ammonium ions and lysine were studied. The response time, linear working range, reproducibility and life time of the biosensor were also determined. The interfering effect of other amino acids on the biosensor performance was also studied and potentiometric selectivity coefficients were calculated. Although the biosensor responded mainly against tyrosine, a lot of amino acids and ascorbic acid that can be present in some real samples did not show any important interference. Additionally, lysine assay in commercial pharmaceutical lysine tablets and capsules was also successfully carried out. The results were in good agreement with previously reported values.
Introduction
In man and other mammals, L-lysine is one of the essential amino acids and it must be a part of the food intake. Insufficient intake of L-lysine can lead to the development of severe diseases. Therefore, it is often added as a dietary supplement to foods and drugs and is used in medicine for preparing infusion solutions as well. Owing to this, the lysine content of foods can be used as an index of nutritional quality or the evaluation of food processing techniques (CitationAkyilmaz et al. 2007), as it is easily damaged by heat treatment and storage conditions (CitationCarpenter and Booth 1973).
The development of analytical methods for the determination of lysine in food products as well as for fast on-line monitoring of the effects of processing and storage conditions on lysine content is thus becoming increasingly important. In this respect, several analytical methods have been proposed for quantitative analysis of lysine in food, including liquid chromatography (CitationThio and Tompkins 1989), reverse phase liquid chromatography (Cubedo CitationFernández-Trapiella 1990) and high performance liquid chromatography (CitationJones and Gilligan 1983). Chromatographic methods, however, require expensive instruments and reagents, long pre-treatment/purification stages and highly trained operators.
Recently, biosensor methods have been developed for L-lysine detection. It would be most advantageous if these sensors are rapid, cheap and easy to make. Lysine biosensors have been constructed mainly by using several lysine-specific enzymes for bioactive components until now ().
Table I. The biosensors developed for L-lysine detection.
The most popular techniques use the enzyme L-lysine oxidase (LOx) and measure either the consumption of oxygen with Clark-type oxygen electrodes, or the formation of hydrogen peroxide (H2O2) with platinum or gold electrodes. Other enzymes have also been used, namely lysine decarboxylase and lysine dehydrogenase. A potentiometric lysine biosensor was constructed by immobilizing L-lysine decarboxylase and used lysine determination in grains and foodstuffs (CitationWhite and Guilbault 1978). Because L-lysine dehydrogenase used to prepare amperometric lysine determination required NAD+ cofactor and a suitable soluble redox mediator in the solution, it was not economical, easy or usable (CitationDempsey et al. 1992). In the case of these lysine-specific enzymes, the sensitivities are less than that of sensors employing LOx (CitationKelly et al. 2000).
The potentiometric detection methods are very advantageous with respect to the simplicity of the technique and the low cost of the instrumentation. A lot of enzymatic reactions of nitrogen-containing compounds can be monitored potentiometrically from the detection of the ammonium released enzymatically, with a suitable sensor. As a result of this, the analysis of several biochemical compounds can be carried out with potentiometric biosensors.
The aim of this work was to develop a lysine-specific potentiometric biosensor based on a PVC ammonium electrode and to carry out a cost effective, rapid, interferent-free method for L-lysine determination in the commercial pharmaceutical lysine capsule and tablet. After the oxidation reaction of L-lysine with L-lysine oxidase (LOx) immobilized on the biosensor, the ammonium ions produced from the reaction were determined potentiometrically.
The buffer concentration, buffer pH, lifetime, response time and optimum working range of the proposed biosensor were determined. The interference effects of ascorbic acid, some ions and amino acids on the biosensor were also determined.
Materials and methods
Reagents and apparatus
L-lysine oxidase from Trichoderma viride (E.C.1.4.3.14) (25 U/mg), 1-ethyl-3-(3-dimetylaminopropyl) carbodiimide (EDC), Trizma Base, Trizma HCl, L-lysine monohydrate, ammonium chloride, monosodium glutamate, L-glutamine, L-alanine, L-arginine, L-valine, L-ascorbic acid, L-histidine, L-methionine, L-serine, L-glycine and L- cysteine were obtained from Sigma Chem. Co. (St. Louis, U.S.A). Nonactin, bis(2-ethylhexyl) sebacate (DOS), poly(vinylchloride) (PVC) and palmitic acid were obtained from Fluka. Tetrahydrofuran (THF) was purchased from Merck (Darmstadt, Germany). All other chemicals used were of analytical reagent grade. Standard solutions and buffer solutions were prepared with deionized water.
For the lysine assay, 1000 mg of tablets and amino 75 capsules were purchased from SOLGAR. Potential and pH measurements were carried out with an ORION 4 Star Benchtop pH-ion meter. The potential values were given against the Ag/AgCl double junction reference electrode (ORION 90-02).
Preparation of the lysine biosensor
The sensors were prepared in two stages:
Preparation of ammonium-ion selective membrane electrode: First of all, 118 mg PVC, 13.6 mg palmitic acid, 12.6 mg nonactin and 255.8 mg bis(2-ethylhexyl) sebacate (DOS) were prepared by dissolving in 5 mL of tetrahydrofuran (THF). The solution was poured on a glass plate inside a glass ring (42 mm diameter). After 24 h of drying at room temperature (20–25°C), the membrane was formed (approximately 0.5 mm thickness). Disks of 5 mm diameter were cut out and attached to the glass electrode body. The inner electrode solution was 1.0 × 10− 2M ammonium chloride (pH 7.5). We used modified ammonium-selective membrane electrode prepared in a previous study (CitationKarakuş et al. 2005).
Chemical immobilization of L-lysine oxidase enzyme onto ammonium-selective membrane electrode surfaces: After 4.4 mg of L-lysine oxidase enzyme was dissolved in 1.0 mL of bidistilled water, 100 μL of this solution was mixed with 0.5 mg of 1-ethyl-3- (3-dimethylaminopropyl) carbodiimide. Ten microliter of this solution was dropped on the membranes of ammonium-selective electrodes and left overnight in a refrigerator at 4°C. Then, 10 μL of 2.5% glutaraldehyde solution was deposited on the surface of this biosensor. The biosensor was left for 30 min and the electrode surface was washed with bidistilled water to remove the excess glutaraldehyde. Then, 10 μL of second stock lysine oxidase enzyme solution (4.4 mg LOx/1 mL bidistilled water) was deposited on the lysine biosensor surface. The biosensor was left overnight at 4°C. To remove the excess unbounded enzymes, all types of biosensors were left for 1 h in a vigorously stirred TRIS buffer (10 mM, pH 7.5). When not in use, biosensors were stored in a refrigerator at 4°C.
Potentiometric measurement
Potentiometric measurements were carried out by varying lysine concentration in the optimum working condition of the prepared lysine biosensor. Ten millimolar of TRIS buffer (pH 7.5) was used as a working buffer solution and potential measurements were made with the proposed lysine biosensor against the Ag/AgCl reference electrode. The prepared lysine biosensor is presented in .
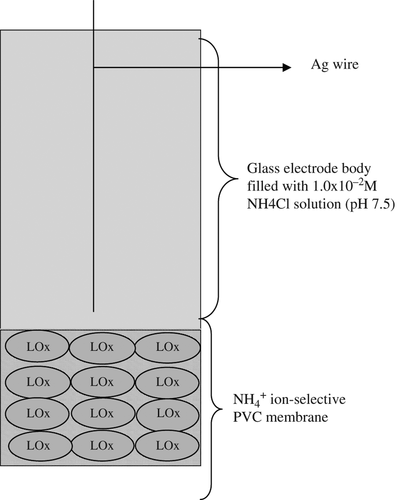
The following electrochemical cell was formed with the proposed lysine biosensor using an Ag/AgCl reference electrode.
After our prepared biosensor and Ag/AgCl reference electrode were immersed together to a depth of 1.5 cm in a lysine solution that was stirred by a magnetic stirrer, the mV values of the solution were determined by using an Orion pH/ion-meter. The amount of L-lysine in the solution was assayed by determining ammonium ions produced after the LOx enzyme reaction. The calibration curve was also obtained by plotting the potential values of a series of standard lysine solutions against the logarithm of lysine concentrations. All the experimental studies were carried out at room temperature (20–25°C).
Application of the lysine biosensor to commercial lysine capsules and tablets
The lysine quantities in commercial lysine capsules and tablets were determined separately by using the standard addition method. A tablet of Solgar (100 mg of L-lysine salt/tablet) and Amino 75 capsule (75 mg of each amino acid/capsule) were dissolved in 25 ml of TRIS buffer (10 mM, pH 7.5). The lysine biosensor was immersed in the 10 mM TRIS buffer (pH 7.4) containing a certain amount of these samples. Then, stock lysine solution (10 − 3M pH 7.5) was added to this solution step by step. After each step, potential values against the added amount (mL) of lysine solution were plotted, and the amount of ammonium was determined by using our prepared ammonium-selective lysine biosensor. To check our results obtained from the proposed lysine biosensor, the values obtained were compared with reported values.
Results and discussion
Ammonium and lysine detection with the lysine biosensor
The prepared novel potentiometric lysine biosensor based on the monitoring of the ammonium enzymatically released was successfully used in determining lysine in commercial lysine capsules and tablets.
First of all, we tested whether our prepared lysine biosensor responds to ammonium-ions and plotted a calibration graph with standard ammonium calibration solutions prepared with 10 mM TRIS buffer (pH 7.5), because we assayed the amount of lysine by determining the ammonium ions produced after enzymatic reaction (). Then, the calibration graph was plotted with the lysine calibration solution prepared with 10 mM TRIS buffer (pH 7.5) ().
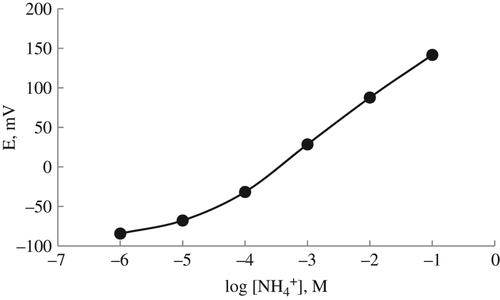
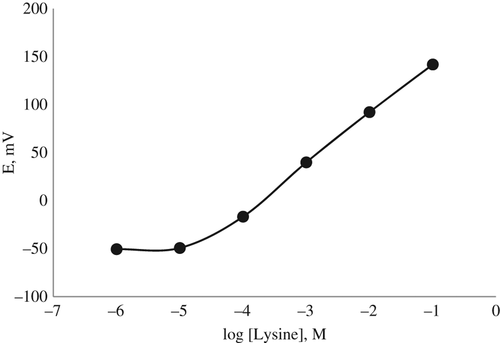
As it can be seen in and , the responses of the lysine biosensor to the ammonium ions and lysine are the same in the concentration ranges of 1.0 × 10− 1–1.0 × 10− 5M. The slopes of the biosensor were 53.18 ± 1.2 mV/p[NH4+] and 49.14 ± 0.58 mV/log[lysine], close to the Nernstian value of 59 mV/substrate concentration. Therefore, we decided that the lysine biosensor can be used for lysine determination in real samples.
CitationDempsey et al. (1992) determined the linear working range of amperometric lysine biosensor prepared by immobilizing lysine decarboxylase between 7 × 10− 8 and 7 × 10− 4M. In a study by CitationLavagnini et al. (1993), the linear range of the lysine biosensors based on LOx enzyme was reported as 1 × 10− 6–2 × 10− 3M; CitationMarconi et al. (1996) reported their prepared potentiometric lysine biosensor prepared by immobilizing LOx as 3 × 10− 5–1 × 10− 3M. It can be said that our prepared lysine biosensor based on the PVC membrane can be used for the determination of lysine in biological samples, with much more advantages because it has a large operating range of 1.0 × 10− 1–1.0 × 10− 5M.
Effect of buffer concentration
For examination of buffer concentration on the response of lysine biosensor, the slopes of the biosensor were measured at six different buffer concentrations varying from 5 to 30 mM. Nernstian responses for all lysine biosensors were obtained at 10 mM TRIS buffer ().
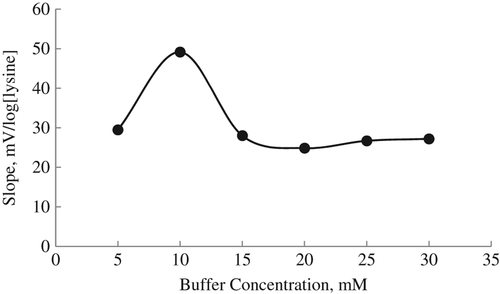
As shown in , the lysine biosensor shows maximum slope value at 10 mM of buffer concentration. Therefore we selected 10 mM as optimum buffer concentration and used 10 mM of TRIS buffer in all experimental studies. We also showed the decrease in biosensor response when the buffer concentration of our biosensor increased. Although we tried to conduct the study with phosphate buffer, we could not be obtain good results. Saurina et al. also selected 10 mM TRIS buffer as the optimum buffer concentration because the stability of the working buffer solution and the linear working range of the biosensor were higher at this concentration (CitationSaurina et al. 1998).
Effect of pH
The influence of pH on the lysine biosensor's response was studied in 10 mM TRIS buffer at six different pH values between 6.0 and 9.0. The sensitivities of the biosensor measured as the slope of the linear part of the calibration curve recorded under stirring are shown in as a function of pH.
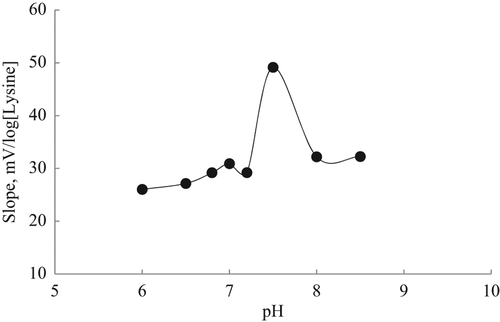
As it can be seen in , the slope of the biosensor increased with the pH increase until the levelling of pH at 7.5. After pH 7.5, the response of the biosensor gradually decreased because the LOx enzyme immobilized on the biosensor denaturated gradually with increasing pH and the time of incubation. Free LOx enzyme has same optimum pH value and it is seen that the immobilization procedure of the LOx enzyme on the ammonium-selective PVC membrane electrode didn't show any negative effect for the optimum pH value of free LOx enzyme. It was also determined that the optimum pH of lysine biosensor was 7.5 in the literature (CitationGuerrieri et al. 2007, CitationAkyilmaz et al. 2007). Consequently, pH 7.5 was selected as the most suitable and optimum pH value for the lysine biosensor.
Effect of temperature
The study of the effect of temperature is also important to determine the optimum performance parameters for a biosensor. To determine temperature effect on the biosensor, the experiments were carried out between 20°C and 45°C under the optimum working conditions obtained from the optimization studies of the biosensor. Then, the slope values of the biosensor were determined by plotting calibration graphs for each temperature ().
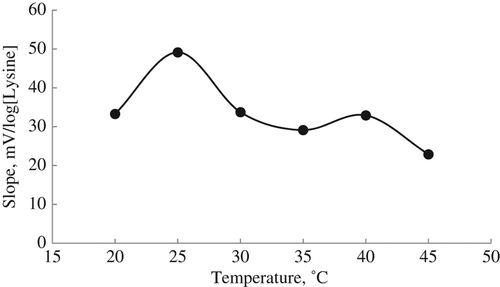
According to , the slope of the biosensor increased until 25°C. The decrease in the slope of the biosensor after 25°C shows that LOx activity immobilized on the biosensor decreased. The studies related to lysine biosensor can be carried out between 30°C and 40°C. It is a known fact that temperature has a significant effect on enzyme activity. Although it is expected that immobilized enzymes are less affected by temperature, LOx immobilized on the biosensor was affected by the temperature increases because the dissociation constants are increased and the ammonium ion concentration of the working solution are decreased at higher temperatures (CitationKelly et al. 2000, CitationAkyilmaz et al. 2007, CitationSaurina et al. 1998).
Selectivity on ammonium-selective lysine biosensor
The interference effects of ascorbic acid and some amino acids - glycine, alanine, valine, methionine, serine, cysteine, arginine, histidine, glutamic acid, glutamine, phenylalanine, leucine, proline, tyrosine, the stock solutions of lysine, 15 different amino acids and ascorbic acid of 10 − 2M were prepared.
After the solutions were mixed with an equal volume of ascorbic acid and amino acid, solutions with lysine were prepared separately at the same concentration and optimum conditions (10 − 3M, pH 7.5), and the mV measurements were carried out for each solution. In addition, measurements were made in lysine solution (10 − 3M, pH: 7.5) without any amino acid and ascorbic acid being added. In this way, the interference effects of amino acids were determined as a percentage while the response of lysine was accepted as 100%. The effect of interference of each amino acid and ascorbic acid is given . Although tyrosine showed a more significant interference effect than other amino acids, the others did not show any negative effect on biosensor response. In addition, the interference effect of ascorbic acid that can present in some real samples was studied and this substance did not show any interference effect on the biosensor.
Table II. Selectivity of lysine biosensor towards ascorbic acid and some amino acids.
Saurina et al., studied the response of the potentiometric lysine biosensor with some amino acids in the same solution and determined the interference effects of ornithine, tyrosine, arginine, phenylalanine, aspartic acid and glutamic acid as 42%, 34%, 13.1%, 14.7%, 8.6%, 4.13%, and 10.1%, respectively. They also stated that the other amino acids showed a negligible signal at the concentration of 10 − 4M lysine (CitationSaurina et al. 1998).
Effect of stirring rate
To study the effect of stirring rate on the responses of the lysine biosensor, the measurements were done in 10 mM TRIS buffer (pH 7.5) at three different stirring rates (100, 250, and 750 rpm) after reaching stable potential. When stirring was done at 100 and 750 rpm levels, the time to reach stable potential was longer than that at the 250 rpm level. A decrease of stirring rate (100 rpm) did not result in any influence on the magnitude of the analytical signal, but caused an increase in the response time of sensors. The explanation for this situation is that enzymatic reaction is slow at a low stirring rate. The rate of enzymatic reactions decreases at a high stirring rate (750 rpm) also, because of possible changes in the enzyme conformation. Therefore, the magnitude of analytical signal is found to be lower. For this reason, 250 rpm is determined as the optimal stirring rate.
Response time
An ideal biosensor should respond in a short time. The response time of the lysine biosensor was determined by recording the time elapsed to reach a stable potential value after the biosensor and the reference electrode were immersed in calibration solutions of lysine, and the biosensor showed response between 0.5 and 1 min.
Storage stability of the lysine biosensors
To determine the lifetime of the lysine biosensor stored in the refrigerator when not in use for a period of 45 days, potential measurements were made during the 45 days and the linear working range and slopes were determined by drawing calibration graphs each day. Activity losses of 10% and 25% of the biosensor were shown after 10 and 45 days, respectively ().
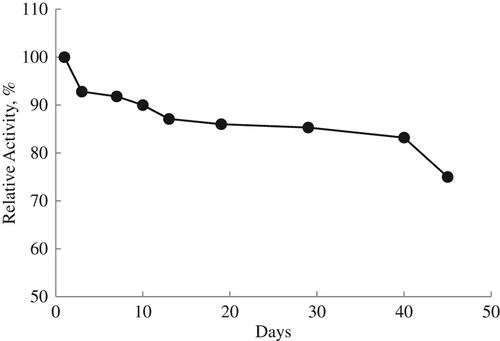
It can be said that our prepared lysine biosensor based on LOx and PVC membrane containing palmitic acid can be easily used in bioanalytical studies because of its long lifetime and wide linear working range. Glutaraldehyde crosslinking also increased the biosensor response.
Reproducibility of the lysine biosensors
To determine the reproducibility of the biosensor prepared, mV measurements were made in 15 serial lysine calibration solutions (pH 7.5) on the same day, and the slopes of the calibration graphs were determined after each measurement (). Numerically, the largest slope value was assumed to be 100% and the other slope values were calculated according to it. It was determined that the lowest slope percent was 85%. The results obtained show that the reproducibility of our lysine biosensor is very good.
Lysine determination in commercial lysine tablets and capsules
We investigated whether our prepared lysine biosensor based on the ammonium-selective PVC membrane containing palmitic acid could be used in lysine tablets and capsules for lysine determination. For this purpose, a lysine assay in these samples were carried out by using the standard addition method. The results obtained with the biosensor and the reported values are shown in .
Table III. The results of lysine content obtained from the proposed lysine biosensor in commercial lysine tablet and capsule.
Conclusion
After the potentiometric ammonium-ion sensor produced by using PVC, palmitic acid and nonactin as an ammonium ionophore was prepared, LOx enzyme was immobilized on the membrane of this sensor. The prepared biosensor allows detection of ammonium produced by the enzymatic oxidation of l-lysine at optimum working conditions. The linear working range and optimum pH were determined as 1 × 10− 1–1 × 10− 5M and 7.5, respectively. No significant interference effect of the amino acids and ascorbic acid were shown on the lysine assay with the biosensor, with the exception of tyrosine. The l-lysine content of pharmaceutical tablets and capsules (and hence protein quality) can be measured rapidly and cheaply and is free from interferents by using our prepared lysine biosensor.
Declaration of interest
The authors report no declarations of interest. The authors alone are responsible for the content and writing of the paper.
We gratefully acknowledge the financial support of Yildiz Technical University Science Research Project Foundation (Project No: 28-01-02-15).
References
- Akyilmaz E, Erdogan A, Öztürk R, Yaşa I. 2007. Sensitive determination of L-lysine with a new amperometric microbial biosensor based on Saccharomyces cerevisiae yeast cells. Biosens Bioelectron. 22:1055.
- Carpenter KJ, Booth VH. 1973. Damage to lysine in food processing: its measurement and its significance. Nutr Abstr Rev. 43:423.
- Chen RLC, Lee MH, Matsumoto K. 1996. Selective biosensing of L-lysine by a low-temperature flow-injection technique using an immobilized lysine oxidase reactor. Anal Sci. 12:87.
- Fernández-Trapiella AC. 1990. Quantitative analysis methionine, cysteine and lysine in feeds by reverse-phase liquid chromatography using precolumn derivatization with 9-fluorenylmethyl chloroformate: preliminary study. J Assoc Off Anal Chem. 73:935.
- Dempsey E, Wang J, Wollenberger U, Ozsoz MR, Smyth MR. 1992. A lysine dehydrogenase-based electrode for biosensing of L-lysine. Biosens Bioelectron. 7:323.
- Guerrieri A, Cataldi TRI, Ciriello R. 2007. The kinetic and analytical behaviours of an l-lysine amperometric biosensor based on lysine oxidase immobilised onto a platinum electrode by co-crosslinking. Sens Actuators B Chem. 126:424.
- Jones BN, Gilligan JP. 1983. O-Phthaldialdehyde precolumn derivatization and reversed-phase high-performance liquid chromatography of polypeptide hydrolysates and physiological fluids. J Chromatogr. 266:471.
- Karakuş E, Pekyardımcı Ş , ve Kılıç E. 2005. Urea biosensors based on PVC membrane containing palmitic acid. Artificial Cells Blood Substitutes and Biotechnology. 33:329–341.
- Kelly SC, O’Connel PJ, O’Sullivan CK, Guilbault GG. 2000. Development of an interferent free amperometric biosensor for determination of L-lysine in food. Anal Chim Acta. 412:111.
- Lavagnini MG, Moscone D, Palleschi G, Compagnone D, Cremisini C. 1993. Amperometric lysine bioprobes analysis in feeds. Talanta. 40:1301.
- Li H, He H, Wolfbeis OS. 1992. An optical biosensor for lysine based on the use of lysine decarboxylase and a cadaverine-sensitive membrane. Biosens Bioelectron. 7:725.
- Marconi E, Panfili G, Messia MC, Cubadda R, Comaagnone D, Palleschi G. 1996. Fast analysis of lysine in food using protein microwave hydrolysis and electrochemical biosensor. Anal Lett. 29:1125.
- Romette JL, Yang JS, Kusakabe H. 1983. Enzyme electrode for specific determination of L-lysine. Biotechnol Bioeng. 25:2557.
- Saurina J, Hernández-Cassou S, Fabregas E, Alegret S. 1998. Potentiomet-ric biosensor for lysine analysis based on a chemically immobilizedlysine oxidase membrane. Anal Chim Acta. 371:49.
- Siegler K, Weber B, Weber E, Tonder K, Klingner E, Riechert F. 1994. A lysine sensor for process control. J Chem Technol Biotechnol. 59:279.
- Simonian AL, Badalian IE, Berezov TT, Smirnova IP, Khaduev SH. 1994. Flow-injection amperometric biosensor on immobilized L-lysine oxidase for L-lysine determination. Anal Lett. 27:2849.
- Thio NP, Tompkins DH. 1989. Regulatory approach to determination of lysine in feedstuffs by liquid chromatography with fluorescence detection via pre-column dansylation. J Assoc Off Anal Chem. 72:69.
- Vrbová E, Marek M, Ralys E. 1992. Biosensor for the determination of L-lysine. Anal Chimica Acta. 270:131.
- White WC, Guilbault GC. 1978. Lysine specific enzyme electrode for determination of lysine in grains and foodstuffs. Anal Chem. 50:1481.