Abstract
The aim of the present study was to formulate methylprednisolone acetate -Eudragit® RS100 nanofibers and nanobeads by the electrospinning method. The physicochemical characteristics of the prepared electrospuns were assessed as well. The particle size and morphology were evaluated using scanning electron microscopy. The crystallinity of the drug in the nanofibers and nanobeads obtained was also studied by X-ray crystallography and differential scanning calorimetry (DSC) thermograms. In addition, FT-IR spectroscopy was applied to investigate any possible chemical interaction between the drug and carrier during the preparation process. The drug release kinetics were considered, to predict the release mechanism. Increasing the concentration of the injected solution resulted in the production of more nanofibers and less nanobeads, with the particle size ranging from 100 to 500 nm. The drug crystallinity was decreased during the electrospinning process; however, no interaction between drug and polymer was observed. The electrospuns showed faster drug release pattern compared to the pure drug. The release data were best fitted to the Weibull model, in which the corresponding shape factor values of the model were less than 0.75 indicating the diffusion mechanism of drug release. In conclusion, electrospinning could be considered as a simple and cost effective method for fabricating the drug: polymer nanofibers and nanobeads.
Introduction
Novel drug delivery systems have attracted the interest of many researchers due to their advantages such as the ability to lead the drug into the target site, less toxicity, patient compliance and convenience as well as improvement of the drug's physicochemical properties and pharmacokinetics (CitationReddy 2000, CitationPrisant et al. 1992). In recent years, nanoformulations have become a dominant concept of modern drug delivery owing to their increased surface area (CitationAkhgari et al. 2013, CitationSnopczynski et al. 2009) and improved dissolution rate of the poorly water-soluble drugs (CitationKocbek et al. 2006). Nanoparticles could be prepared by different techniques such as emulsification solvent evaporation, self-assembly, nanoprecipitation, drawing template synthesis (CitationKrishna Sailaja et al. 2011), aerosol flow reactor (CitationEerikäinen et al. 2003) and electrospinning methods (CitationSode et al. 2013). At the end of the twentieth century, electrostatic spinning, namely electrospinning, attracted the interest of the researchers due to its capability to produce ultrafine fibers (CitationMartins et al. 2008). This process can be mostly applied in a large scale, owing to its simplicity and cost effectiveness (CitationBhardwaj and Kundu 2010). Electrospinning has three important components including a high voltage supplier, syringe and collector (CitationPham et al. 2006). This technique uses a high voltage electrical field to form solid fibers from a liquid drug: polymer solution so that the drug particles are encapsulated in the dry ultrathin layer nanofibers or nanobeads (CitationDoustgani et al. 2011). Nanobeads could be used for oral administration as seen in many research studies for different drugs, for example, nanoparticles of paclitaxel (CitationZabaleta et al. 2012), insulin (CitationDamgé et al. 1988) and azithromycin (CitationMohammadi et al. 2010) or for ocular delivery as reported for methylprednisolone acetate (CitationAdibkia et al. 2007a). Moreover, nanofibers could be incorporated in the implants due to better integration with the extra-cellular matrix (CitationSargeant et al. 2008, CitationLiu and Webster 2007) or wound healing dressings for topical drug delivery (CitationKim et al. 2004). Different parameters such as distance between the needle and collector, voltage, the drug: polymer ratio and solution concentration could affect the nanofiber's characteristics (CitationChowdhury and Stylios 2010). Electrospun nanoscale preparations have been used in nanocatalysis (CitationChinnappan and Kim 2012), tissue engineering scaffolds (CitationLuu et al. 2003), protective clothing (CitationSubbiah et al. 2005), filtration (CitationRajesh and Natarajan 2009), biomedicine, optical electronics (CitationWang et al. 2002), biotechnology and environmental engineering (CitationThavasi et al. 2008). This process has also been applied in pharmaceutical science to improve the physicochemical characteristics of naproxen (CitationCanbolat et al. 2013), ibuprofen (CitationPires et al. 2013, CitationShi et al. 2013), doxycycline (CitationChaturvedi et al. 2013), mebeverine (CitationIllangakoon et al. 2014), sumatriptan (CitationVrbata et al. 2013), indomethacin (CitationAkhgari et al. 2013), and β-estradiol (CitationOh and Lee 2013).
Eudragit® RS100 is a cationic copolymer of poly(ethyl acrylate, methyl–methacrylate and chlorotrimethyl– ammonioethyl methacrylate. Furthermore, the hydrophilic character of the Eudragit® RS100 causes the polymer to get somewhat swelled up at the physiologic pH. The polycationic nature of Eudragit® RS100, resulting from the presence of quaternary ammonium groups, makes it a suitable carrier to encapsulate the anionic drugs and also attach to the negatively charged cells (CitationAdibkia et al. 2007a, CitationLoveymi et al. 2012).
Methylprednisolone acetate (MPA) is a synthetic water-insoluble glucocorticoid with a strong and prolonged anti-inflammatory, immunosuppressive and anti-allergic activity. MPA nanoparticles have been prepared using some techniques such as quasi-emulsion solvent diffusion (CitationAdibkia et al. 2007a) and self-assembly with cyclodextrin (CitationHwang et al. 2008). However, to the best of our knowledge, MPA-Eudragit® RS100 electrospuns have not been prepared and studied so far. In the present work, MPA-Eudragit® RS100 nanofibers and nanobeads were formulated using the electrospinning technique and the physicochemical characteristics of the preparations were assessed.
Materials and methods
Materials
Eudragit® RS100, from Deguss, was a kind gift from the Akbarieh Co. (Tehran, Iran). Methylprednisolone acetate was purchased from Iran-Hormoon Co. (Tehran, Iran). Ethanol and potassium monobasic phosphate were obtained from Merck Company (Germany). All other chemicals were of the highest available purity.
Preparation of the electrospuns
MPA and Eudragit® RS100 with the drug: polymer ratios of 1:5 and 1:10 were dissolved in the ethanol with magnetic stirring. The concentrations of the drug: polymer solutions were adjusted to be 10, 15 and 20% (w/v). The prepared solutions were dropped with a constant flow rate (5 ml/h) via a syringe to the charge field. The distance between the needle of the syringe and collector was 10 cm and the DC voltage was controlled at 25 KV. Solid nanofibers and nanobeads were obtained on the collector and stored in microtubes for further analysis.
Scanning electron microscopy
Scanning electron microscopy (SEM) was used to scrutinize the surface morphology and shape of the particles. The samples were mounted on aluminum stubs and coated with gold under an argon gas environment. Micrographs were achieved using a Scanning Electron Microscope (LEO 440i, Leo Electron Microscopy Ltd., Cambridge, UK) operated at 20 KV.
Differential scanning calorimetry
Differential scanning calorimetry (DSC) was used to evaluate the drug's thermal behavior in the formulations. The accurately weighed samples (5 mg) were placed and sealed into aluminum pans. The thermograms were recorded over the temperature range of 40°C–240°C at the rate of 20°C/min. Aluminum oxide and indium powders were employed as reference and standard, respectively. Thermograms were recorded and analyzed using the TA60 software.
X-ray powder crystallography
X-ray powder diffraction patterns were investigated using a diffractometer (Siemens, Germany) with Cu Kα radiation of wavelength 1.5405A°. The step width was 0.02°. Samples were run between 2θ = 5–40° with the scan rate of 2 degrees per minute. The samples were ground using a mortar and pestle prior to examination and placed in an aluminum holder.
Fourier transformed infrared spectroscopy
The fourier transformed infrared spectroscopy (FT-IR) analysis was conducted to verify the possibility of chemical interaction between the drug and polymer. The analysis was performed with FT-IR spectrophotometer (Shimadzu 43000, Shimadzu) by the KBr disk method. The scanning range was 400–4000 cm− 1 and the resolution was 2 cm− 1. The spectra were obtained by averaging 32 scans.
Dissolution study and drug release kinetics
The release rates of MPA from the physical mixtures with the drug: polymer ratios of 1:5 and 1:10, the pure MPA and the nanoformulations were determined using USP apparatus II with the paddle stirrer. The dissolution medium was 300 ml phosphate buffer (pH = 6.8) and the temperature was controlled at 37°C with continuous orbital mixing (50 rpm). All the investigated formulations contained an equivalent of 20 mg of MPA. At the specified time intervals, 3 ml of media was removed by syringe and filtrated using a cellulose acetate membrane (Whatman, UK) with the pore diameter of 20 nm. The volume removed was replaced with fresh media and the concentration of MPA was assayed at 247.2 nm using a UV spectrophotometer (Shimadzu, Kyoto, Japan). The cumulative amount of the released MPA was calculated for 8 h and plotted versus time.
Data obtained from the in vitro release studies were fitted into nine common kinetic models including: zero order, first order, Higuchi, power law, Hixson-Crowell, Square root of mass, Three seconds root of mass, Weibull and Linear probability (CitationBarzegar-Jalali 2008, CitationWagner 1969, CitationCosta and Sousa Lobo 2001) to find out the mechanism of drug release from the prepared formulations . Squared correlation coefficient (RSQ) and percent error (PE) were calculated for each model as well.
Results and discussion
Morphology of the electrospuns
SEM images of the drug-loaded nanostructures are shown in . The results indicated that the particle size and the diameter of the fibers ranged from 100 to 500 nm, and that enhancing the solution concentration resulted in the increase of fiber diameter. The concentration of the injected solution was the main factor affecting the morphology of the formulated electrospuns. Injecting the solution with low concentration (10%) resulted in discrete beads while the enhanced solution concentration (15%) resulted in the increased amount of nanofibers and decreased fraction of nanobeads. Smooth nanofibers without the presence of any nanobeads were obtained employing the solution with the concentration of 20% (). Concavities on the surface of the beads may have resulted from evaporation of the solvent.
Figure 1. SEM images for MPA-Eudragit® RS100 electrospuns. F1–F3: samples with the drug: polymer ratio of 1:5 and solution concentrations of 10% (F1), 15% (F2) and 20% (F3). F4–F6: samples with the drug: polymer ratio of 1:10 and solution concentrations of 10% (F4), 15% (F5) and 20% (F6).
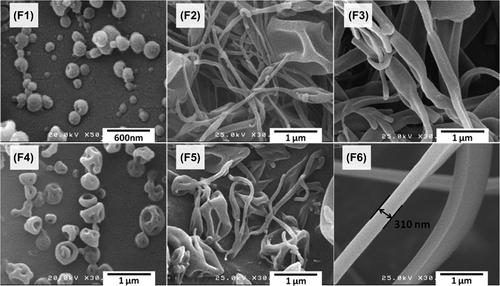
The electric charge on the surface of the liquid resulted from the high voltage electric field which induces electrostatic repulsion forces which in turn create a liquid jet. Formation of the bead like structures in the low solution concentrations of the liquid is due to the instability of the jet with a low surface tension, which breaks the jet into separate droplets. Conversely, the viscoelasticity of the solution- when sufficient enough- overcomes the surface tension and prevents the jet break, giving rise to the formation of the beads-on-string or uniform nanofibers (CitationYu et al. 2006).
Physicochemical characterization
DSC thermograms and X-ray powder diffractograms were applied to study the drug crystallinity alterations during the electrospinning process. DSC thermograms of the MPA, Eudragit® RS100, physical mixtures and the prepared nanoformulations are presented in . The Eudragit® RS100 thermogram suggested that the glass transition temperature was 58.44°C. DSC analysis of MPA showed a single endothermic peak at 212.77°C, related to the drug's melting point. The characteristic endothermic peak of the drug disappeared in the physical mixture because of the MPA solvation in the amorphous carrier during the heating process and/or the interaction between drug and polymer increased by heating.
Figure 2. DSC thermograms of MPA; Eudragit® RS100; PM 1:5 (physical mixture with the drug: polymer ratio of 1:5) and formulations with the drug: polymer ratio of 1:5 prepared using 10% (ME 1:5 10%) and 20% (ME 1:5 20%) solution concentrations.
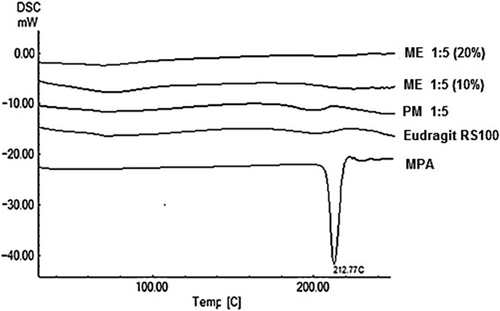
No clear peak presented in the thermograms of the prepared nanofibers and nanobeads due to any possible decrease in the drug crystallinity and/or solvation of the drug in the melted carrier and/or heat-induced interaction between drug and polymer (CitationAdibkia et al. 2007b, CitationJavadzadeh et al. 2010). Such findings have been reported for naproxen-Eudragit® RS100 nanoparticles prepared by the quasi-emulsion solvent diffusion technique (CitationAdibkia et al. 2011). Similarly, this kind of interaction was previously reported for the physical mixture of piroxicam/PVP k-90 (CitationAdibkia et al. 2007b, CitationTantishaiyakul et al. 1999).
X-ray diffractograms of the pure MPA, polymer, physical mixture of the drug and polymer and the obtained nanoformulations are shown in . An X-ray examination of the pure MPA exhibited dominant peaks at 2θ = 13.2, 14, 14.5, 15.1, 17.2 and 19◦, attributed to the crystalline state of MPA, whereas the amorphous Eudragit® RS100 diffractogram contained no major peak. The decreased height of the peaks in the physical mixture diffractogram was due to the dilution effect caused by the polymer network.
Figure 3. X-Ray diffractograms of MPA; Eudragit® RS100; PM 1:5 (physical mixture with the drug: polymer ratio of 1:5) and nanoparticles with the drug: polymer ratio of 1:5 prepared using 10% (NP 1:5 10%) and 20% (NP 1:5 20%) solution concentrations.
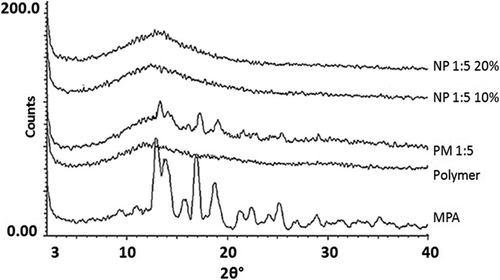
The prepared electrospuns showed no distinct diffraction peaks, possibly due to the drug amorphization (CitationAdibkia et al. 2007c, CitationPignatello et al. 2002). The X-ray powder crystallography (XRPD) findings were in consistence with the DSC results.
Possible interactions between the drug and polymer were assessed by FT-IR analysis. FT-IR spectra of the MPA, physical mixture and the nanoformulations are demonstrated in . MPA showed the characteristic peaks at 1735 and 1248 cm− 1 (the range of C = O and C-O stretching), 1600 cm− 1(-C = C- aromatic), and 1650 cm− 1(CO bounded to the pentagonal ring). Comparing the FT-IR spectrum of the physical mixture and the electrospuns revealed no chemical interaction between the MPA and Eudragit® RS100. Similar results have been stated for the nanoparticles of MPA-Eudragit® RS100 prepared with the quasi-emulsion solvent diffusion technique (CitationAdibkia et al. 2007a).
Dissolution study and drug release kinetics
Drug release profiles of the pure MPA, physical mixtures, as well as the prepared nanofibers and nanobeads are presented in . There was no meaningful difference between the release pattern of the pure MPA and its physical mixtures with the polymer. All the electrospuns revealed faster drug release rate in comparison with the pure drug and physical mixtures. The initial burst release within the first 2 h followed by the subsequent plateau release was recognized in the release patterns of all resulting electrospuns. The burst effect could be due to the high surface area of the nanostructures as well as the tendency of the drug molecules to accumulate on the surface. Accordingly, the initial phase is initiated by dissolution and diffusion of the drug from the superficial layers while the drug release from the core of the nanofibers contributes to the plateau phase (CitationYu et al. 2009, CitationMohammadi et al. 2010, CitationMidhun et al. 2011).
Figure 5. Drug release profiles of the pure MPA; physical mixtures and the prepared electrospuns. Each point is the average of three replications and the vertical bars represent standard deviations.
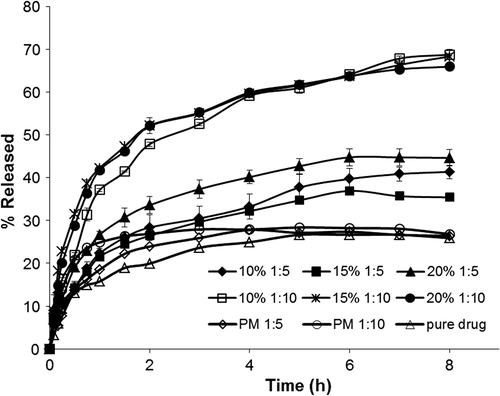
The release patterns were not considerably affected by the concentration of the injected solution; however, enhancing the polymer amount resulted in an increase of the drug release rate. This could be owing to the fact that enhancing the polymer amount may possibly cause a more homogeneous dispersion of the drug molecules within the polymer matrices (CitationAdibkia et al. 2007a, CitationPignatello et al. 2000, Citation2001). Besides, increasing the polymer amount may initiate a more permeable matrix of the drug: polymer nanostructures, which in turn allows for a better penetration of the dissolution medium through the matrix. Therefore, the drug: polymer composition is possibly the main factor for the release rate. However, it seems that a complex phenomenon between the drug and polymer molecules may occur, including drug entrapment within polymer molecules and the adsorption of the drug molecules on the surface of polymeric matrix because of the electrostatic adhesions (CitationAdibkia et al. 2007a, CitationDouglas et al. 1986).
Kinetic study of the drug release is every so often valuable in obtaining one or two physically meaningful parameters which are beneficial for comparative purposes and for relating the release parameters with important factors such as bioavailability. Additionally, a kinetic parameter can be used to evaluate the influence of formulation factors on the drug release (CitationBarzegar-Jalali et al. 2008). Comparing the RSQ and PE values, the Weibull model was found to be the best fitting model for all the electrospuns. The value of the exponent β is an important indicator to predict the drug release mechanism through the polymer matrix. The estimated values of β ≤ 0.75 specify Fickian diffusion while β values in the range of 0.75–1 indicate a combined mechanism of Fickian diffusion and swelling-controlled release (CitationAdibkia et al. 2007b). As seen in the , the resulting β values were less than 0.75, representing that diffusion was the dominant mechanism of drug release from the electrospuns.
Table I. Parameters of the different kinetic models; squared correlation coefficients (RSQ); and percent error (PE) for the formulations F1–F6.
Conclusion
The SEM results revealed that MPA-Eudragit® RS100 nanobeads and nanofibers could be prepared using the electrospinning method while the size and morphology of the resulting particles mainly depended on the concentration of the applied solution. The DSC and XRPD analyses indicated that the drug crystallinity was decreased during the process. The FT-IR spectra displayed no chemical interaction between MPA and Eudragit® RS100 during the electrospinning procedure. All the prepared nanostructures showed faster drug release rate in comparison with the pure drug and the physical mixtures. The adjustable formulation parameters together with the simplicity and cost effectiveness of this method make it a promising technique for industrial production.
Acknowledgement
The authors would like to thank Research Vice-Chancellor of Tabriz University of Medical Sciences, Tabriz, Iran. This article is based on a thesis submitted for Pharm D degree in the Faculty of Pharmacy, Tabriz University of Medical Sciences, Tabriz, Iran.
Declaration of interest
The authors report no declarations of interest. The authors alone are responsible for the content and writing of the paper.
References
- Adibkia K, Javadzadeh Y, Dastmalchi S, Mohammadi G, Niri FK, Alaei-Beirami M. 2011. Naproxen-eudragit RS100 nanoparticles: preparation and physicochemical characterization. Colloids Surf B Biointerfaces. 83:155–159.
- Adibkia K, Omidi Y, Siahi MR, Javadzadeh AR, Barzegar-Jalali M, Barar J, et al. 2007a. Inhibition of endotoxin-induced uveitis by methylprednisolone acetate nanosuspension in rabbits. J Ocul Pharm Ther. 23:421–432.
- Adibkia K, Shadbad MRS, Nokhodchi A, Javadzedeh A, Barzegar-Jalali M, Barar J, et al. 2007b. Piroxicam nanoparticles for ocular delivery: physicochemical characterization and implementation in endotoxin-induced uveitis. J Drug Target. 15:407–416.
- Adibkia K, Siahi Shadbad MR, Nokhodchi A, Javadzedeh A, Barzegar-Jalali M, Barar J, et al. 2007c. Piroxicam nanoparticles for ocular delivery: physicochemical characterization and implementation in endotoxin-induced uveitis. J Drug Target. 15:407–416.
- Akhgari A, Heshmati Z, Sharif Makhmalzadeh B. 2013. Indomethacin electrospun nanofibers for colonic drug delivery: preparation and characterization. Adv Pharm Bull. 3:85–90.
- Barzegar-Jalali M. 2008. Kinetic analysis of drug release from nanoparticles. J Pharm Pharm Sci. 11:167–177.
- Barzegar-Jalali M, Adibkia K, Valizadeh H, Shadbad MR, Nokhodchi A, Omidi Y, et al. 2008. Kinetic analysis of drug release from nanoparticles. J Pharm Pharm Sci. 11:167–177.
- Bhardwaj N, Kundu S. 2010. Electrospinning: A fascinating fiber fabrication technique. Biotechnol Adv. 28:325–347.
- Canbolat MF, Celebioglu A, Uyar T. 2013. Drug delivery system based on cyclodextrin-naproxen inclusion complex incorporated in electrospun polycaprolactone nanofibers. Colloids Surf B Biointerfaces. 115C:15–21.
- Chaturvedi TP, Srivastava R, Srivastava AK, Gupta V, Verma PK. 2013. Doxycycline poly e-caprolactone nanofibers in patients with chronic periodontitis - a clinical evaluation. J Clin Diagn Res. 7:2339–2342.
- Chinnappan A, Kim H. 2012. Nanocatalyst: Electrospun nanofibers of PVDF – Dicationic tetrachloronickelate (II) anion and their effect on hydrogen generation from the hydrolysis of sodium borohydride. Int J Hydrogen Energy. 37:18851–18859.
- Chowdhury M, Stylios G. 2010. Effect of experimental parameters on the morphology of electrospun nylon 6 fibres. Int J Basic Appl Sci. 10:70–78.
- Costa P, Sousa Lobo JM. 2001. Modeling and comparison of dissolution profiles. Eur J Pharm Sci. 13:123–133.
- Damgé C, Michel C, Aprahamian M, Couvreur P. 1988. New approach for oral administration of insulin with polyalkylcyanoacrylate nanocapsules as drug carrier. Diabetes. 37:246–251.
- Douglas S, Davis S, Illum L. 1986. Nanoparticles in drug delivery. Crit Rev Ther Drug Carrier Syst. 3:233–261.
- Doustgani A, Vasheghani-Farahani E, Soleimani M, Hashemi- Najafabadi S. 2011. Preparation and characterization of aligned and random nanofibrous nanocomposite scaffolds of poly (vinyl alcohol), poly (e-Caprolactone) and nanohydroxyapatite. Int J Nanosci Nanotechnol. 7:127–132.
- Eerikäinen H, Watanabe W, Kauppinen E, Petri Ahonen P. 2003. Aerosol flow reactor method for synthesis of drug nanoparticles. Eur J Pharm Biopharm. 55:357–360.
- Hwang J, Rodgers K, Oliver JC, Schluep T. 2008. α-Methylprednisolone conjugated cyclodextrin polymer-based nanoparticles for rheumatoid arthritis therapy . Int J Nanomed. 3:359.
- Illangakoon UE, Nazir T, Williams GR, Chatterton NP. 2014. Mebeverine-loaded electrospun nanofibers: physicochemical characterization and dissolution studies. J Pharm Sci. 103:283–292.
- Javadzadeh Y, Ahadi F, Davaran S, Mohammadi G, Sabzevari A, Adibkia K. 2010. Preparation and physicochemical characterization of naproxen–PLGA nanoparticles. Colloids Surf B. 81:498–502.
- Kim K, Luu YK, Chang C, Fang D, Hsiao BS, Chu B, Hadjiargyrou M. 2004. Incorporation and controlled release of a hydrophilic antibiotic using poly (lactide-co-glycolide)-based electrospun nanofibrous scaffolds. J Control Release. 98:47–56.
- Kocbek P, Baumgartner S, Kristl J. 2006. Preparation and evaluation of nanosuspensions for enhancing the dissolution of poorly soluble drugs. Int J Pharm. 312:179–186.
- Krishna Sailaja A, Amareshwar P, Chakravarty P. 2011. Different techniques used for the preparation of nanoparticles using natural polymers and their application. Int J Pharm Pharm Sci. 3.
- Liu H, Webster TJ. 2007. Nanomedicine for implants: A review of studies and necessary experimental tools. Biomaterials. 28:354–369.
- Loveymi BD, Jelvehgari M, Zakeri-Milani P, Valizadeh H. 2012. Design of vancomycin RS-100 nanoparticles in order to increase the intestinal permeability. Adv Pharm Bull. 2:43.
- Luu YK, Kim K, Hsiao BS, Chu B, Hadjiargyrou M. 2003. Development of a nanostructured DNA delivery scaffold via electrospinning of PLGA and PLA-PEG block copolymers. J Control Release. 89:341–353.
- Martins A, Reis RL, Neves NM. 2008. Electrospinning: processing technique for tissue engineering scaffolding. Int Mater Rev. 53:257–274.
- Midhun B, Shalumon K, Manzoor K, Jayakumar R, Nair S, Deepthy M. 2011. Preparation of budesonide-loaded polycaprolactone nanobeads by electrospraying for controlled drug release. J Biomater Sci Polym Ed. 22:2431–2444.
- Mohammadi G, Valizadeh H, Barzegar-Jalali M, Lotfipour F, Adibkia K, Milani M, et al. 2010. Development of azithromycin– PLGA nanoparticles: Physicochemical characterization and antibacterial effect against Salmonella typhi. Colloids Surf B Biointerfaces. 80:34–39.
- Oh B, Lee CH. 2013. Advanced cardiovascular stent coated with nanofiber. Mol Pharm. 10:4432–4442.
- Pham Q, Sharma U, Mikos A. 2006. Electrospinning of polymeric nanofibers for tissue engineering applications: a review. Tissue Eng. 12:1197–1211.
- Pignatello R, Amico D, Chiechio S, Spadaro C, Puglisi G, Giunchedi P. 2001. Preparation and analgesic activity of Eudragit RS100® microparticles containing Diflunisal. Drug Deliv. 8:35–45.
- Pignatello R, Bucolo C, Ferrara P, Maltese A, Puleo A, Puglisi G. 2002. Eudragit RS100 nanosuspensions for the ophthalmic controlled delivery of ibuprofen. Eur J Pharm Sci. 16:53–61.
- Pignatello R, Consoli P, Puglisi G. 2000. In vitro release kinetics of Tolmetin from tabletted Eudragit microparticles. J Microencapsul. 17:373–383.
- Pires LR, Guarino V, Oliveira MJ, Ribeiro CC, Barbosa MA, Ambrosio L, Pego AP. 2013. Ibuprofen-loaded poly(trimethylene carbonate-co-epsilon-caprolactone) electrospun fibres for nerve regeneration. J Tissue Eng Regen Med. (Epub ahead of print).
- Prisant LM, Bottini B, DiPiro JT, Carr AA. 1992. Novel drug-delivery systems for hypertension. Am J Med. 93:45S–55S.
- Rajesh KP, Natarajan TS. 2009. Electrospun polymer nanofibrous membrane for filtration. J Nanosci Nanotechnol. 9:5402–5405.
- Reddy KR. 2000. Controlled-release, pegylation, liposomal formulations: new mechanisms in the delivery of injectable drugs. Ann Pharmacother. 34:915–923.
- Sargeant TD, Guler MO, Oppenheimer SM, Mata A, Satcher RL, Dunand DC, Stupp SI. 2008. Hybrid bone implants: self-assembly of peptide amphiphile nanofibers within porous titanium. Biomaterials. 29:161–171.
- Shi Y, Wei Z, Zhao H, Liu T, Dong A, Zhang J. 2013. Electrospinning of ibuprofen-loaded composite nanofibers for improving the performances of transdermal patches. J Nanosci Nanotechnol. 13:3855–3863.
- Snopczynski T, Goralczyk K, Czaja K, Strucinski P, Hernik A, Korcz W, Ludwicki JK. 2009. [Nanotechnology–possibilities and hazards]. Rocz Panstw Zakl Hig. 60:101–111.
- Sode K, Tanaka M, Suzuki Y, Kawakami H. 2013. Nickel nanoparticle chains inside carbonized polymer nanofibers: preparation by electrospinning and ion-beam irradiation. Nanoscale. 5: 8235–8241.
- Subbiah T, Bhat GS, Tock RW, Parameswaran S, Ramkumar SS. 2005. Electrospinning of nanofibers. J Appl Polym Sci. 96:557–569.
- Tantishaiyakul V, Kaewnopparat N, Ingkatawornwong S. 1999. Properties of solid dispersions of piroxicam in polyvinylpyrrolidone. Int J Pharm. 181:143–151.
- Thavasi V, Singh G, Ramakrishna S. 2008. Electrospun nanofibers in energy and environmental applications. Energy Environ Sci. 1:205–221.
- Vrbata P, Berka P, Stranska D, Dolezal P, Musilova M, Cizinska L. 2013. Electrospun drug loaded membranes for sublingual administration of sumatriptan and naproxen. Int J Pharm. 457:168–176.
- Wagner JG. 1969. Interpretation of percent dissolved‐time plots derived from in vitro testing of conventional tablets and capsules. J Pharm Sci. 58:1253–1257.
- Wang X, Drew C, Lee SH, Senecal KJ, Kumar J, Samuelson LA. 2002. Electrospun nanofibrous membranes for highly sensitive optical sensors. Nano Lett. 2:1273–1275.
- Yu D-G, Zhu L-M, White K, Branford-White C. 2009. Electrospun nanofiber-based drug delivery systems. Health. 1:67–75.
- Yu JH, Fridrikh SV, Rutledge GC. 2006. The role of elasticity in the formation of electrospun fibers. Polymer. 47:4789–4797.
- Zabaleta V, Ponchel G, Salman H, Agüeros M, Vauthier C, Irache JM. 2012. Oral administration of paclitaxel with pegylated poly (anhydride) nanoparticles: permeability and pharmacokinetic study. Eur J Pharm Biopharm. 81:514–523.