Abstract
Background: The combination of hyperthermia and controlled drug delivery is a very promising recent effort in cancer therapy. The aim of this study is to synthesize and characterize a dual pH/thermal-responsive composite nanoparticle that acts as a cisplatin carrier, and to evaluate its release profile at different pH and temperature conditions relevant to the physiological and cancerous environment during hyperthermia. Methods: Poly (n-isopropyl acrylamide-methacrylic acid-hydroxy ethyl methacrylate) (P (NIPAAM-MAA-HEM)) was synthesized by emulsion polymerization. Fe3O4 magnetic nanoparticles (MNPs) and cisplatin were loaded onto the nanogel, by the swelling method and the conjugation of cisplatin with the −COOH group of MAA, respectively. The chemical and morphological properties of the drug-loaded composite nanoparticle and its profile of drug release at pH levels of 7.4 (physiological pH), 6.8 (tumor extracellular pH), and 5.3 (endosomal pH), and at temperatures of 37°C (physiological) and 43°C (hyperthermia), were studied. Results: The result shows that the synthesized nanogel and nanocomposite were almost pure and were of an appropriate size and stability. Magnetic saturation was at a position appropriate for the production of enough heat during hyperthermia. A high level of drug release under conditions of low pH and high temperature was observed. Conclusion: This result suggests that the dual pH/temperature-responsive P (NIPAAM-MAA-HEM) magnetic nanocomposite can be very effective in hyperthermia and controlled cisplatin delivery.
Introduction
Chemotherapy and thermotherapy are conventional methods for treating cancer. Unfortunately, both of them have major side effects, and unnecessarily kill normal cells. In recent years, much research has been devoted to intelligent drug delivery (CitationSutradhar and Amin 2014). Progress in nano medicine has aided this effort. Cancer therapy is the most challenging issue because of the complexity of this disease and the overwhelming side effects of the anticancer drugs (CitationDobbelstein and Moll 2014). Many materials, from natural to synthetic and from different processes, have been used for targeted drug delivery (CitationGoldberg et al. 2007). Designing carrier nanoparticles that can discriminate between healthy and cancerous cells and release the drug primarily in the cancerous medium, is a very important task, and much work has gone into it (CitationDavaran et al. 2013). Physiological differences between healthy and cancerous cells, like pH, can be used in this matter (CitationHensley et al. 2013). The synthetic polymer is one such material that has been recently used in this regard, because of its biocompatibility and biodegradability (CitationAkbarzadeh et al. 2012). Some of these polymers have received FDA approval. A hydrogel is a cross-linked polymer that can absorb water in a watery environment (swelling), and spill it in response to a triggering stimulus (CitationDavaran et al. 2001). A temperature-sensitive hydrogel can adsorb a hydrophilic drug in a watery environment and release it in a temperature at which it undergoes shrinkage, called the lower critical solution temperature (LCST) (CitationAkbarzadeh et al. 2012). When the temperature is raised to the LCST, the hydrophilic/hydrophobic balance of the hydrogel changes, resulting the shrinkage of the hydrogel and the release of drug. Thus, these hydrogels can be used in hyperthermia therapy to release the drug in the desired location. MNPs have recently been used in noninvasive thermotherapy because they can convert magnetic energy to heat (CitationAkbarzadeh et al. 2012). A magnetic thermosensitive hydrogel nanocomposite (MTHN) is an encapsulated MNP with a thermosensitive hydrogel that shrinks and releases the drug in an oscillating magnetic manner because of the heat produced by MNPs (CitationDavaran et al. 2012). A dual pH/temperature-sensitive hydrogel is synthesized with pH and temperature-sensitive monomers. Any change in pH can change its LCST, and therefore its shrinkage and releasing temperature (CitationSingh and Lee 2014). A nano-scale hydrogel is called a nanogel. Poly (n-isopropyl acrylamide co- methacrylic acid co- hydroxyethylmethacrylate) (P (NIPAAM co-MAA co-HEM)) nanogel is a dual pH/temperature-sensitive hydrogel that swells at temperatures lower than LCST (CitationKarnoosh-Yamchi et al. 2014). NIPAAM is temperature-sensitive, and MAA and HEM are pH-sensitive as well (CitationConstantin et al. 2014). Raising the temperature or decreasing the pH can diminish the hydrogen bonding between water and the hydrogel molecule, and increase the hydrophobicity of the gel, thereby causing shrinkage and decreasing size of the nanogel (CitationMolina et al. 2013). In the present work, the dual pH/temperature-sensitive nanocomposite was synthesized, and (II) (cisplatin) was loaded onto it by the swelling method. The physicochemical properties of the nanogel and the release of drug at different temperature and pH levels were studied to reveal the relation between pH/temperature and drug release, which is very important in designing a smart drug delivery system.
Materials and methods
Material and instruments
N-isopropyl acrylamide (NIPAAM) was purchased from Acros Organics (NJ, USA), and recrystallized in n-hexane-toluene (90/10, v/v) before use. Hydroxy ethyl methacrylate (HEM), Methacrylic acid (MAA), N-N methylene bis acrylamide (BIS), and Potassium per sulfate (KPS) were purchased from Merck (Germany). Sodium dodecyl sulfate (SDS) was received from Sigma and was used as supplied. MNPs were purchased from US Research Nanomaterials Inc. (TX, USA). Cis-diamine-dichloroplatinum (II) (CDDP) was obtained from Merck (Germany). Sodium mono hydrogen phosphate and dihydrogen phosphate were purchased from Sigma-Aldrich Co. (Steinheim, Germany). NaOH was obtained from (Merck Chemical Co. (Germany)).
Following are the instruments used, and their manufacturers: Freeze dryer (Telstar, Spain) Magnetic stirrer (Heidolph, Germany), Shaker incubator (WiseCube, Germany), Dialysis membrane (Cellu SepH1) 0.5 μm membrane with a MWCO of 2000 (Millex AP, Millipore), UV-Visible Spectrophotometer (Cecil CE, Germany), Sonicator (Hielscher, Germany), Heater (Wise Cube, Germany), Centrifuge (Labnet, USA), Nuclear Magnetic resonance (NMR) spectroscope (Brucker DRX, MA, USA), Scanning Electron Microscope (SEM) (TESCAN, Czech Republic), Vibrating sample magnetometer (VSM) (Meghnatis Daghigh Kavir Co, Iran), and Dynamic laser scattering (DLS) (Malvern, England).
Methods
Preparation of P (NIPAAM - MAA – HEM) nanogel
The free radical emulsion polymerization method was used to prepare the terpolymer nanogel. NIPAAM, MAA, and HEM were dispersed in water in a molar ratio of 70/20/10 respectively. BIS was used as a cross-linker with different weight ratios (ratios of 1%, 3% and 5% of monomer weight). SDS was used as a surfactant agent at a ratio of 3% of monomer weight. To initiate the polymerization process, potassium per sulfate (KPS) was used as the initiator (at a ratio of 3.5% weight of monomers). After 30 min of degassing the solution mixture with N2, the rising temperature of the mixture started to reach 70°C, and the initiator was then allowed to drop onto the solution slowly. The polymerization process was continued for 4 h at this temperature. After that, the polymer solution (latex) was cooled. Purification of the synthesized polymer was done using a dialysis bag membrane against distilled water for 5 days. Some of the synthesized polymer was freeze-dried and stored for further experiments. The remainder was kept as a solution for the SEM experiment (CitationAhmadi et al. 2014, CitationDavaran et al. 2013,CitationGhasemali et al. 2013, CitationSadat Tabatabaei Mirakabad et al. 2014,CitationDavaran et al. 2014, CitationKouhi et al. 2014).
Preparation of cisplatin-loaded magnetic hydrogel nanocomposite (CPMHN)
The cisplatin-loaded magnetic hydrogel nano composite (CPMHN) was prepared by dissolving the hydrogel in NaOH solution in the presence of cisplatin and MNPs. Typically, 100 mg of MNPs and 50 mg of cisplatin were dispersed in 40 ml of NaOH (25 mM) solution and then sonicated for 15 min at a 0.5 cycle and 50% amplitude. The temperature of the solution rose to 50°C. Then, 400 mg of freeze-dried nanogel (with different cross-linker ratios) was dispersed in the solution and then sonicated again for 5 min at the above-mentioned conditions. The solution was left for 48 h in a shaker incubator at 5°C, to allow the swelling of nanogel and the encapsulation of cisplatin and MNPs in the nanogel. After that, the solution was centrifuged, the supernatant was removed and precipitated. The nanocomposite was washed with NaOH and then dialyzed against deionized water for 3 days, to remove NaOH and free cisplatin. The dialyzed solution was centrifuged and then freeze-dried and stored for further application (CitationKouhi et al. 2014, CitationAbbasi et al. 2014, CitationPourhassan-Moghaddam et al. 2014, CitationAbbasi et al. 2014, CitationEatemadi et al. 2014, CitationHosseininasab et al. 2014, CitationDavoudi et al. 2014).
Measurement of drug-loading and encapsulation efficiency
The content of cisplatin in the separated supernatant and NaOH obtained in the washing stage were measured using UV spectrophotometry at 225 nm, to obtain drug-loading (DL) and encapsulation efficiency (EE). If we consider:
The following formula was used to obtain them:
The nanogel with the best DL capacity was chosen for further experiments.
Furthermore, the content of cisplatin in the best-loaded nanocomposite was measured with Energy Dispersive X-ray Spectroscopy (EDX), which was done with SEM.
Measurement of swelling behavior
The nanogel with the best loading was chosen for swelling measurements by the gravimetric method. 10 mg of synthesized hydrogel was dissolved in 2 ml of water at different levels of pH (7.4, 6.8 and 5.3) and at different temperatures (25°C, 37°C and 43°C), and left to equilibrate for 24 h. After removing excess water by filter paper, the nanogel was weighed. If WS = Weight of gel in swollen state and Wd = Weight of gel in dried state, the swelling ratio (Q) can be measured using the following equation:
LCST measurement of both nanogel and CPMHN
To determine the LCST of aqueous nanogel and nanocomposite at three pH values (5.3, 6.8 and 7.4), the optical light transmittance method was used, at 500 nm with the UV-visible spectrophotometer (Cecil CE, Germany). For this, 0.5 mg/ml of nanogel and nanocomposite were dissolved in PBS at each pH and left for 24 h at 25°C, to allow complete swelling of the nanogel and nanocomposite. After that, each sample was placed in a spectrophotometer and transmittance was measured at the heating rate of 1°C/min. The LCST of the nanogel and nanocomposite was defined as the temperature showing 50% of transmittance.
Chemical characterization of hydrogel and CPMHN
Characterization of the hydrogel and drug-loaded nanocomposite was carried out by Fourier Transform Infrared (FTIR) spectroscopy using the KBr disk. The nanogel was dispersed in dimethyl sulfoxide (DMSO) and characterized with NMR spectroscopy.
Particle size analysis
Particle size of the synthesized CPMHN was studied with MALVERN DLS at a concentration of 0.5 mg ml− 1 and 25°C, for a duration of 10 s.
Morphological study of hydrogel and CPMHN
The morphological properties of the synthesized nanogel and nanocomposite were observed with the SEM (VEGA, TESCAN, Czech Republic). One drop of nanogel and nanocomposite were placed on aluminum foil and left to dry. After coating, the sample was observed under 10 kV with SEM.
Study of the magnetic properties of CPMHN
The VSM was used to evaluate the magnetic properties of the nanocomposite and compare it to the magnetic properties of the Fe3O4 MNP. The sample was placed in an applied field of 0–10kOe.
Study of in vitro drug release of CPMHN
First, 20 mg of drug-loaded magnetic nanocomposite was dispersed in PBS, in test tubes with different pH values (7.4 represents the physiological pH, 6.8 is the pH of extracellular tumor, and 5.3 is tumor endosomal pH). A dispersion of the composite nanoparticle was placed in a shaker incubator with different temperatures (25°C for testing its stability, 37°C representing physiological body temperature, and 43°C representing hyperthermia temperature). At specific time intervals, the test tubes were gently shaken and then centrifuged at 5000 rpm for 5 min. Then 1 mg of the solution, free of nanoparticles, withdrawn and replaced with fresh PBS. The withdrawn solution was stored in micro tubes for UV spectroscopy (CitationTaheri Anganeh et al. 2014, CitationAlimirzalu et al. 2014, CitationEatemadi et al. 2014, CitationKarnoosh-Yamchi et al. 2014).
Result and discussion
Chemical structure of P (NIPAAM-MAA-HEM)
shows the chemical structure of the synthesized hydrogel. After synthesis of the nanogel, the reaction yields calculated were 84%, 85%, and 79%, for1%, 3%, and 5% cross-linker hydrogel, respectively. The synthesized hydrogel has five main parts: the NIPAAM group, MAA group, HEM group, cross-linker segment (BIS), and the backbone. The carboxylic group of MAA is responsible for conjugation with cisplatin. MNPs and some of the cisplatin incorporate in the network of the gel. Physically incorporated cisplatin seems be released faster than cisplatin conjugated with the carboxylic group of MAA.
shows the FTIR spectrum of the synthesized nanogel. The peaks at 1650 cm− 1 and 1690 cm− 1 belong to stretching carbonyl group of NIPAAM. The peak at 1730 cm− 1 belongs to the C = O stretch of the carbonyl group of the ester bond between the backbone and HEM. The backbone C–C stretching appears at 800–1000 cm− 1. The absorbance bands for HEM are: 3400 cm− 1 for OH, 2990 cm− 1 for CH3 and CH2, and 1074 cm− 1 for O–C of alcohol. The peak at 1717 cm− 1 belongs to the carboxylic group of MAA.
Figure 2. FTIR spectrum of a) P (NIPAAM-MAA-HEM) nanogel, and b) P (NIPAAM-MAA-HEM) drug-loaded magnetic hydrogel nanocomposite.
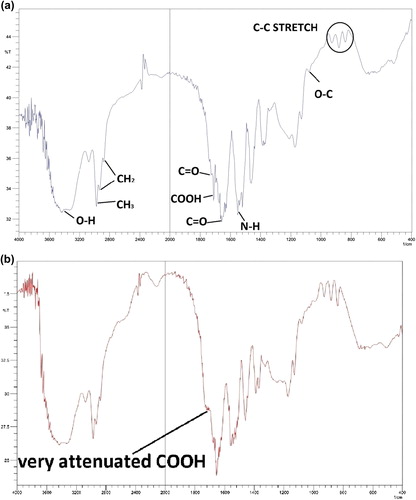
shows the FTIR spectrum of the synthesized cisplatin-loaded nanocomposite. The peaks are almost matched, except for the disappearance of a peak that was representative of the carboxylic group of MAA. This event shows the conjugation between OH of the carboxylic group of MAA and the Pt of cisplatin.
shows the NMR spectrum of the nanogel. The terminal isopropyl methyl protons display a high peak at 1.1 ppm. The peak at 3.8 ppm belongs to isopropyl methylene protons. The carboxylic group of MAA protons appears in a weak peak at 12 ppm. The methylene protons of the nanogel backbone appeared at 1.3–2.2 ppm. The amide N–H Group has its peak at 8.1–8.2 ppm. This structure that NMR and FTIR show is consistent with the expected chemical structure. The high purity of the final product is revealed from the NMR peak.
Swelling behavior
shows the swelling behavior of the nanogel at corresponding pH and temperature levels. As seen, the swelling behavior of nanogel is directly related to pH and inversely related to temperature. That is because the carboxylic group of MAA and the OH group of HEM become deprotonated in high pH, and electrostatic repulsion between these groups causes more swelling of the nanogel. Furthermore, at high temperatures, the interaction between the hydrophobic parts of NIPAAM in the nanogel increase, resulting in the decrease of hydrogen bonding between nanogel and water, and hence its collapse (CitationTomar et al. 2014).
Table I. Swelling behavior of the nanogel at different values of pH and temperature.
LCST measurement of nanogel and nanocomposite
and show the LCST diagram of the nanogel and nanocomposite respectively. For the nanogel, the LCST is 53°C, 44°C and 39°C, for pH of 7.4, 6.8 and 5.3, respectively. Likewise, the parameters for the nanocomposite are 48°C, 41°C, and 38°C, for pH values of 7.4, 6.8, and 5.3 respectively. The LCST of both nanogel and nanocomposite increase with increasing pH. That is because of the ionization of –COOH and –OH of MAA and HEM that produce electrostatic repulsion between the gel matrix and result in the high LCST. The LSCT of the nanocomposite is lower than that of the nanogel, which is the result of hindrance by the Fe3O4 MNP on swelling and motion of the polymer (CitationKhan 2008).
Drug-loading and encapsulation efficiency
The values for DL and EE of the nanogel with different cross-linkers are shown in . With increasing cross-linker density, the loading capacity is decreased. Predictably, more cross-linkers make the hydrogel more compact, preventing diffusion of NaOH and cisplatin, thereby allowing effective conjugation. Drug-loading capacity is increased at low temperature and high pH. That can be interpreted as being due to the deprotonation of the carboxylic group of MAA and the expansion of the nanogel at high pH, which facilitate the catalytic action of NaOH in the reaction between the –COOH group of MAA and the Pt of cisplatin. Furthermore, as mentioned in the section on swelling behavior, at low temperature below LCST, hydrogen bonding between the polymer and the environment is high, resulting in high incorporation of drug in the nano void of the hydrogel. shows the EDX spectrum of the drug-loaded nanocomposite. The Pt content of the nanocomposite is 4.3% of weight, which shows the drug loading of 6.7% .The measure of cisplatin content obtained in EDX is very close to that measured using spectroscopy (CitationCheng et al. 2013).
Table II. Relation of drug-loading and cross-linker density.
Particle size analysis
shows the size of the drug-loaded nanocomposite particle in deionized water. The average hydrodynamic size of the particle was 294 nm. The size of the nanoparticle is very important in formulations that are prepared for injection. Large particles can be eliminated by the reticulo endothelial system in a very short time, consequently decreasing its effects. The size of the nanoparticle obtained seems be appropriate for injection (CitationKulkarni and Feng 2013).
Morphological study
shows the SEM micrograph of the nanogel and the drug-loaded nanocomposite. As shown, the size of the nanocomposite is a little greater than that of the nanogel. That could be explained by the fact that some of the MNPs are absorbed on the surface of the nanogel and the interaction between their charged surfaces acts as a cue for the clustering of the nanocomposite and its growth in size. The micrograph of the nanocomposite shows that the particles are almost mono dispersed. This property is necessary for the application of MNPs in hyperthermia, to produce more heat corresponding to the applied magnetic field (CitationKim et al. 2013).
Magnetic properties
shows the magnetic curve of the Fe3O4 MNP and the cisplatin-loaded magnetic nanocomposite. The magnetic saturation for cisplatin-loaded nanocomposite is about 18 emu/g, compared to that of the Fe3O4 MNP, which was found to be 80 emu/g. That shows that the MNP is highly encapsulated in hydrogel matrices. The high saturation magnetization for the cisplatin composite nanoparticle can produce appropriate heat in an alternative magnetic field (AMF) that is necessary for hyperthermia therapy. The decrease in coercivity in the cisplatin composite nanoparticle is due the preventive effect of the polymer shell on the aggregation of the Fe3 O4 MNPs (CitationGillich et al. 2012).
Stability of the cisplatin-loaded composite nanoparticle
shows the cisplatin release from the composite nanoparticle during a 14-day interval, at a temperature of 25°C. The cumulative cisplatin release from the complex at the end of the experiment was only 31%, which shows the high stability of the loaded cisplatin for storage at room temperature.
Table III. Cumulative release of cisplatin at a temperature of 25°C and pH of 7.4.
In vitro drug release from the cisplatin-loaded composite nanoparticles
shows drug release from the composite nanoparticle, over a time interval of 336 h at a temperature of 37°C and 43°C, for each corresponding pH. The drug release was much faster at low pH and high temperature. At low pH, the conjugation between Pt and COO− was attacked by H+ and caused the release of cisplatin into the PBS medium. Moreover, at high temperature, the collapse of the NIPAAM segment makes it more compact and decreases the size of the nano void in the hydrogel matrix. Thus, the cisplatin that was already released by the H+ attack, and the cisplatin that was stored in the hydrogel matrix, release faster. It is also shown that the release of drug at higher pH (6.8 and 7.4) does not become significantly faster at high temperature, comparing to the release at a pH of 5.3. This shows that the decreasing pH has more effect on the speed of drug release than increasing temperature. From the last statement, we can conclude that the most cisplatin is conjugated to the –COOH group of the hydrogel, which can release it only in an acidic environment (CitationMa et al. 2012).
Conclusion
The synthesis, characterization, and drug release evolution of the P (NIPAAM-MAA-HEM) composite nanoparticle was studied. The nanogel synthesized with the emulsion polymerization method produces gel particles of an almost nanometer scale. Fe3O4 MNPs and cisplatin were encapsulated in dual-responsive gel nanoparticles with the methods of swelling and chemical conjugation between cisplatin and the –COOH group of MAA, respectively. The magnetic saturation of the drug-loaded composite nanoparticles was at an appropriate level, which means that it can produce enough heat for application in hyperthermia. The complex has good stability in retaining the loaded drug and maintaining size. Composite nanoparticles show high drug release at low pH and high temperature, which are conditions relevant to the cancer medium during hyperthermia. The dual pH/temperature-responsive nanocomposite shows its efficiency for the combined application in hyperthermia and controlled-drug delivery.
Authors’ contributions
SD conceived of the study and participated in its design and coordination. AA participated in the sequence alignment and drafted the manuscript. All authors read and approved the final manuscript.
Acknowledgments
The authors thank the Department of Medical Nanotechnology of the Faculty of Advanced Medicine and Drug Applied Research Center of Tabriz University of Medical Sciences, for all support provided.
Declaration of interest
The authors have no declaration of interest. The authors alone are responsible for the content and writing of the paper.
References
- Abbasi, Aval, Akbarzadeh, Milani, Nasrabadi, JooAbbasi E, Aval SF, Akbarzadeh A, Milani M, Nasrabadi HT, Joo SW. 2014. Dendrimers: synthesis, applications, and properties. Nanoscale Res Lett. 9:247
- Abbasi E, Milani M, Fekri Aval S, Kouhi M, Akbarzadeh A, Tayefi Nasrabadi H, et al. 2014. Silver nanoparticles: synthesis, properties, bio-applications and limitations. Crit Rev Microbiol. 1–8.
- Ahmadi A, Shirazi H, Pourbagher N, Akbarzadeh A, Omidfar K. 2014. An electrochemical immunosensor for digoxin using core-shell gold coated magnetic nanoparticles as labels. Mol Biol Rep. 41:1659–1668.
- Akbarzadeh A, Mikaeili H, Zarghami N, Mohammad R, Barkhordari A, Davaran S. 2012. Preparation and in vitro evaluation of doxorubicin-loaded Fe3O4 magnetic nanoparticles modified with biocompatible copolymers. Int J Nanomedicine. 7:511–526.
- Akbarzadeh A, Samiei M, Joo SW, Anzaby M, Hanifehpour Y, Nasrabadi HT. 2012. Synthesis, characterization and in vitro studies of doxorubicin-loaded magnetic nanoparticles grafted to smart copolymers on A549 lung cancer cell line. J Nanobiotechnology. 10:46.
- Akbarzadeh A, Zarghami N, Mikaeili H, Asgari D, Goganian AM, Khiabani HK, et al. 2012. Synthesis, characterization, and in vitro evaluation of novel polymer-coated magnetic nanoparticles for controlled delivery of doxorubicin. Nanotechnol Sci Appl. 5:13–25.
- Alimirzalu S, Akbarzadeh A, Abbasian M, Alimohammadi S, Davaran S, Hanifehpour Y, et al. 2014. Synthesis and study of physicochemical characteristics of Fe3O4 magnetic nanocomposites based on poly(Nisopropylacrylamide)for anti-cancer drugs delivery. Asian Pac J Cancer Prev. 15:49–54.
- Cheng R, Meng F, Deng C, Klok HA, Zhong Z. 2013. Dual and multi-stimuli responsive polymeric nanoparticles for programmed site-specific drug delivery. Biomaterials. 34:3647–3657.
- Constantin M, Bucatariu S, Ascenzi P, Simionescu BC, Gheorghe F, et al. 2014. Poly(NIPAAm-co-β-cyclodextrin) microgels with drug hosting and temperature-dependent delivery properties. React. Funct. Polym. 84:1–9.
- Davaran S, Akbarzadeh A, Nejati-Koshki K, Alimohammadi S, Ghamari MF, Soghrati MM. et al. 2013. In vitro studies of NIPAAM-MAA-VP copolymer-coated magnetic nanoparticles for controlled anticancer drug release. J Encapsul Adsorpt Sci. 3:108–115.
- Davaran S, Mamaghani PY, Kaffashi B. 2012. Synthesis and physicochemical characterization of ph-and thermosensitive nanopolymers based on N-isopropylacrylamide containing doxorubicin anti-cancer agent. Int J Green Nanotechnol. 4:389–393.
- Davaran S, Rashidi MR, Hashemi M. 2001.Synthesis and characterization of methacrylic derivatives of 5-amino salicylic acid with pH-sensitive swelling properties. AAPS PharmSciTech. 2:80–85.
- Davaran S, Rezaei A, Alimohammadi S, Khandaghi AA, Nejati-Koshki K, Tayefi Nasrabadi H, et al. 2014. Synthesis and physicochemical characterization of biodegradable star-shaped poly lactide-co-glycolide– β-cyclodextrin copolymer nanoparticles containing albumin. Adv Nanopart. 3:14–22.
- Davoudi Z, Akbarzadeh A, Rahmatiyamchi M, Movassaghpour AA, Alipour M, Nejati-Koshki K, et al. 2014. Molecular target therapy of akt and nf-kb signaling pathways and multidrug resistance by specific cell penetrating inhibitor peptides in hl-60 cells. Asian Pac J Cancer Prev. 15:4353–4358.
- Dobbelstein M, Moll U. 2014. Targeting tumour-supportive cellular machineries in anticancer drug development. Nat Rev Drug Discov. 13:179–196.
- Eatemadi A, Daraee H, Karimkhanloo H, Kouhi M, Zarghami N, Akbarzadeh A, et al. 2014. Carbon nanotubes: properties, synthesis, purification, and medical applications. Nanoscale Res Lett. 9:1–13.
- Eatemadi A, Daraee H, Zarghami N, Yar HM, Akbarzadeh A, Hanifehpour Y. 2014. Nanofiber: synthesis and biomedical applications. Artif Cells Nanomed Biotechnol. 1–11.
- Ghasemali S, Nejati-Koshki K, Akbarzadeh A, Tafsiri E, Zarghami N, Rahmati-Yamchi M, et al. 2013. study of inhibitory effect of β-cyclodextrin-helenalin complex on HTERT gene expression in T47D breast cancer cell line by real time quantitative PCR (q-PCR). Asian Pac J Cancer Prev. 14:6949–6953.
- Gillich T, Acikgöz C, Isa L, Schlüter AD, Spencer ND, Textor M. 2012. PEG-stabilized core–shell nanoparticles: impact of linear versus dendritic polymer shell architecture on colloidal properties and the reversibility of temperature-induced aggregation. ACS nano. 7:316–329.
- Goldberg M, Langer R, Jia X. 2007. Nanostructured materials for applications in drug delivery and tissue engineering. J Biomater Sci Polym Ed. 18:241–268.
- Hensley CT, Wasti AT, DeBerardinis RJ. 2013. Glutamine and cancer: cell biology, physiology, and clinical opportunities. J Clin Invest. 123:3678–3684.
- Hosseininasab S, Pashaei‐Asl R, Khandaghi AA, Tayefi Nasrabadi H, Nejati-Koshki K, Akbarzadeh A, et al. 2014. Synthesis, characterization, and in vitro studies of PLGA‐PEG nanoparticles for oral Insulin delivery. Chem Biol Drug Des. 84:307–315.
- Karnoosh-Yamchi J, Mobasseri M, Akbarzadeh A, Davaran S, Ostad-Rahimi AR, Hamishehkar H, et al. 2014. Preparation of pH sensitive insulin-loaded Nano hydrogels and evaluation of insulin releasing in different pH conditions. Mol Biol Rep. 41:6705–6712.
- Khan A. 2008. Preparation and characterization of magnetic nanoparticles embedded in microgels. Mater Lett. 62:898–902.
- Kim E, Lee K, Huh YM, Haam S. 2013. Magnetic nanocomplexes and the physiological challenges associated with their use for cancer imaging and therapy. J Mater Chem B. 1:729–739.
- Kouhi M, Vahedi A, Akbarzadeh A, Hanifehpour Y, Joo SW. 2014. Investigation of quadratic electro-optic effects and electro absorption process in GaN/AlGaN spherical quantum dot. Nanoscale Res Lett. 9:131–136
- Kulkarni SA, Feng SS. 2013. Effects of particle size and surface modification on cellular uptake and biodistribution of polymeric nanoparticles for drug delivery. Pharm Res. 30:2512–2522.
- Ma WF, Wu KY, Tang J, Li D, Chuan Wei, Jia Guo. 2012. Magnetic drug carrier with a smart pH-responsive polymer network shell for controlled delivery of doxorubicin. J. Mater. Chem. 22:15206–15214.
- Molina R, Ligero C, Jovančić P, Bertran E. 2013. In situ polymerization of aqueous solutions of NIPAAm initiated by atmospheric plasma treatment. Plasma Processes Polym. 10:506–516.
- Pourhassan-Moghaddam M, Zarghami N, Mohsenifar A, Rahmati Yamchi M, Gholizadeh D, Akbarzadeh A, et al. 2014. Watercress-based gold nanoparticles: biosynthesis, mechanism of formation and study of their biocompatibility in vitro. IET Digital Library. 5:345–350.
- Sadat Tabatabaei Mirakabad F, Nejati-Koshki K, Akbarzadeh A, Yamchi MR, Milani M, Zarghami N. 2014. PLGA-based nanoparticles as cancer drug delivery systems. Asian Pac J Cancer Prev. 15:517–535.
- Singh NK, Lee DS. 2014. In situ gelling pH- and temperature-sensitive biodegradable block copolymer hydrogels for drug delivery. J. Controlled Release. 193:214–227.
- Sutradhar KB, Amin ML. 2014. Nanotechnology in cancer drug delivery and selective targeting. ISRN Nanotechnol. 2014:12.
- Taheri Anganeh M, Tabatabaei Mirakabad FS, Izadi M, Zeighamian V, Badrzadeh F, Salehi R, et al. 2014. The comparison between effects of free curcumin and curcumin loaded PLGA-PEG on telomerase and TRF1 expressions in calu-6 lung cancer cell line. Int J Biosci. 4:134–145.
- Tomar LK, Tyagi C, Choonara YE, Kumar P, Pillay V. 2014. Rheological and swelling behavior of pH sensitive hydrogel particles. APCBEE Procedia. 9:192–196.