Abstract
Context: Recently, umbilical cord blood (UCB) has been recognized as a suitable potential source of hematopoietic stem/progenitor cells (HSPCs) for transplantation. Lengthy thrombocytopenia after UCB transplantation is a major problem because of insufficient megakaryocyte (Mk) progenitors, which results in delayed platelet recovery. Frequent allogenic platelet transfusion leads to resistance to platelet units and higher risk of transmission of pathogenic agent. Objective: Ex vivo expansion of HSPCs and their differentiation to Mk progenitors on aminated PES nanofiber could lead to faster platelet recovery after UCB transplantation. Materials and methods: CD34 cells were positively enriched using the MidiMACS system. CD34+ cells were seeded onto conventional culture and aminated PES scaffold. The proliferation of CD34+ cells, and also their differentiation into Mk progenitors, were evaluated. We used the flow cytometric method for analyzing CD41 and CD61 markers and real-time PCR for the expression level of transcription factors, as NF-E2 and GATA-1. Results: This study indicated increased CD34+ cell population in aminated PES compared to the conventional system. After differentiation, the amount of CD41/CD61-expressing cells and the quantity of NF-E2 expression level increased in the aminated PES versus the 2-D system. The quantity of GATA-1 expression level was reduced on CD41/CD61+ cells compared to CD34+ cells, with no difference between the aminated PES and the conventional system. Discussion: Aminated PES nanofiber could have more effect on the proliferation of CD34+ cells and Mk differentiation than the conventional culture. Conclusion: Injection of the expanded cells and differentiated Mk progenitors, along with the transplantation of UCB stem cells might accelerate recovery of platelets and decrease the period of thrombocytopenia after transplantation.
Introduction
The first transplantation of hematopoietic stem cells (HSCs) from umbilical cord blood (UCB) was successfully performed in a child with severe Fanconi anemia in 1988 (CitationGluckman et al. 1989). UCB is a suitable alternative source of hematopoietic stem/progenitor cells (HSPCs) for HSC transplantation (CitationArmitage 1994). Regardless of the abundant advantages of using UCB for transplantation compared to other cell sources, the low number of HSPCs contained in a single UCB unit, and the consequently delayed platelet recovery, has limited successful transplantation (CitationLaughlin 2001, CitationKoh et al. 2007). Platelet recovery in cord blood (CB) transplantation takes a longer period compared to that in bone marrow (BM) or mobilized peripheral blood (MPB)cell transplantation (CitationChen et al. 2009), as the average of number of days for platelet engraftment in UCB and MPB is nearly 70 and 20 days, respectively (CitationIgnatz et al. 2007). Thus, ex vivo expansion of megakaryocytes (Mk) derived from UCB along with HSCs might accelerate Mk differentiation and platelet recovery after transplantation. It has been shown that the proliferative rate of CD34+ cells of CB, in response to interleukin (IL -3), stem cell factor (SCF), and thrombopoietin (TPO), is higher than those in BM cells. Nevertheless, the megakaryocytic differentiation of CB CD34+ progenitors in the same in vitro culture system is less than that in BM (CitationMiyazaki et al. 2000).
In general, an efficient promising strategy for improving delayed platelet recovery after CB transplantation is an ex vivo expansion of Mk from HSCs (CitationChen et al. 2009). The conventional culture plates with two-dimensional (2-D) devices could not prepare the microarchitecture required for interaction between the cell and substrate, in addition to the restricted ability for exciting self-renewal of early progenitors. The interaction between HSPCs and their extracellular matrix (ECM) components plays an important role in the survival, homing, fate choice, proliferation, and differentiation of the cells. It was observed that surface chemistry and topographical cues could impress on the rate of HSPC proliferation, CD34+ cell expansion, and cell-cell and cell-matrix interactions. Therefore, the three-dimensional (3-D) culture medium played an important role in promoting hematopoiesis (CitationLi et al. 2001, CitationZhang et al. 2003, CitationEhring et al. 2003, CitationChua et al. 2006).
LaIuppa et al. screened a variety of polymer, metal, and glass substrates with supporting expansion of hematopoietic cells (CitationLaIuppa et al. 1997). Experiments have shown that nanofiber scaffolds fabricated by the electrospinning technique significantly increase cell capture percentage. The scaffolds biomimic the microenvironmental niche of BM for facilitating the proliferation or differentiation functions of HSCs (CitationMa et al. 2008). Moreover, aligned nanofibers result in increasing ECM production of human ligament fibroblasts (HLF) (CitationLee et al. 2005).
Among unmodified, hydroxylated, carboxylated, and aminated nanofibers and films experimented with in ex vivo expansion of UCB-derived CD34+ cells, aminated nanofiber mesh and film were found to be most efficient in developing the expansion of the CD34+ and CD45+ cells, as compared to polystyrene culture media. Furthermore, the aminated nanofiber mesh could augment the HSPC–substrate adhesion (CitationChua et al. 2006).
In a similar study, Hatami et al. used a two-stage protocol in which expansion of CD34+ cells, and then Mk differentiation, were evaluated. The human BM mesenchymal stem cell (MSC) feeder layer was utilized in a pre-established protocol for cell expansion, and the Mk differentiation stage was carried out by using TPO and IL-3 (CitationHatami et al. 2014).
We hypothesized that aminated polyethersulfone (PES) nanofibers could prompt the proliferation of CD34+ cells and differentiation of HSPCS into megakaryocytes, ex vivo.
Materials and methods
Collection of umbilical cord blood
UCB samples were obtained from healthy full-term neonates after obtaining approval from the university's ethical committee in the Alzahra hospital of Tabriz, transferred to the laboratory at 4°C, and processed within 4 h.
Isolation of CD34+ cells from umbilical cord blood
CB samples were diluted with phosphate buffer saline (PBS) containing 2mMEDTA and 0.5% bovine serum albumin (BSA: Stem Cell Technologies, Vancouver, BC, Canada). Mononuclear cells (MNC) were separated by centrifugation at 1500 RPM (400 × g) for 35 min at room temperature over Ficoll Hypaque (1.077 g/cm3, GE Healthcare), collected from the interface layer and washed twice with PBS. CD34+ cells were enriched by positive selection using the CD34 micro bead kit and a Miltenyi MidiMACS system, according to the manufacturer’s protocol (Miltenyi Biotec, Bergisch Gladbach, Germany).
Preparation of aminated PES nanofiber
Aminated PES nanofibers (molecular weight: 35,000 daltons, fabricated by electrospinning) were purchased from the Bonyakhte Company, in ready form. The nanofibers were sterilized in 70% ethanol, and then loaded into 24-well culture plates (Nunc).
Culture of CD34+ cells in the conventional culture (2-D) and PES nanofiber (3-D) conditions
CD34+ cells were cultured in both systems with serum-free StemSpan medium (Stem Cell Technologies, Vancouver, BC, Canada), at a density of 4 × 104 cells/ml. To assess the expansion, the CD34+ cells were cultured in StemSpan media supplemented with FLT3 (100 ng/ml), SCF (100 ng/ml), and TPO (100 ng/ml), at 37°C and 5% CO2 (CitationNikougoftar Zarif et al. 2011) for 7 days. All cytokines were obtained from Stem Cell Technologies, Vancouver, BC, Canada.
For the analysis of differentiation, the cell culture was performed in two stages. In the first stage, CD34+ cells were cultured onto only the conventional system with FLT3 (100 ng/ml), SCF (100 ng/ml), and TPO (100 ng/ml), and incubated for 3 days at 37°C with 5% CO2. Cells were harvested after 3 days of expansion. by 2-D and 3-D), with the same density, and stimulated only by TPO (100 ng/ml) (CitationNikougoftar Zarif et al. 2011). The cells were harvested on day-7 of the differentiation process (second stage) from both the systems. However, for isolating cells from the PES scaffolds, we washed the nanofibers twice with PBS (2x) supplemented with 2mMEDTA.
Analysis of CD34, CD38, CD41, and CD61 expression by flow cytometry
The cells were taken for the assessment of CD34, CD38, CD41, and CD61 expression level on the cultured cells. The following antibodies were used: CD34-PE (BD Biosciences, Becton Dickinson, USA), CD38-FITC, CD41-FITC, and CD61-FITC (DakoCytomation, Denmark). The CD38 marker was used to confirm the negativity of human hematopoietic stem cell maturation. The cell suspension was prepared with the final volume of 50 μl in 0.01 M PBS, and then 10 μl of antibody was added. The cell samples were incubated in the dark at 4°C for 20 min. After the incubation time, the cells were washed twice with PBS containing BSA (2%), and resuspended in paraformaldehyde (1%). Flow cytometric analysis was performed by the FACSCalibur analyzer (BD Biosciences, USA).
Real-time PCR
The CD34 + cells selected by the Miltenyi MidiMACS system, and the differentiated CD41/61 + cells, were harvested, and total RNA was extracted with QIAzol (QIAGEN, USA), and cDNA was synthesized using the RevertAid™ First Strand cDNA Synthesis Kit and random hexamers (Fermentas, Canada). The Mk gene-specific primer sequences were as follows: for the GAPDH gene (as intrinsic control), TCTCTGCTCCTCCTGTTC (forward) and GTTGACTCCGACCTTCAC (reverse), for the NF-E2 gene, GGATGACAATG GCAACAAG (forward) and TAGACAAGGTGGAGGCTAA (reverse), and for the GATA-1 gene, TCCTCCACACCAGAATCA (forward) and TCAGC GTC CCTGTAGTAG (reverse). Data was analyzed using the 2^ (− delta delta Ct) method, as previously described (CitationLivak and Schmittgen 2001). Relative gene expression from differentiated cells was calculated relative to the expression of the same gene non-differentiated.
Statistical analysis
The results were evaluated by SPSS Ver. 17.0 and analyzed by the paired sample T test. The values of p < 0.05 were considered significant.
Results
Isolation of CD34+ cells and ex vivo expansion
The effect of aminated PES nanofiber in the development of expansion of CD34+ cells was evaluated in a 7-day culture. The purity of CD34+ cells was determined as 88% by flow cytometry (), and the viability was 86% (approximated by Trypan blue).
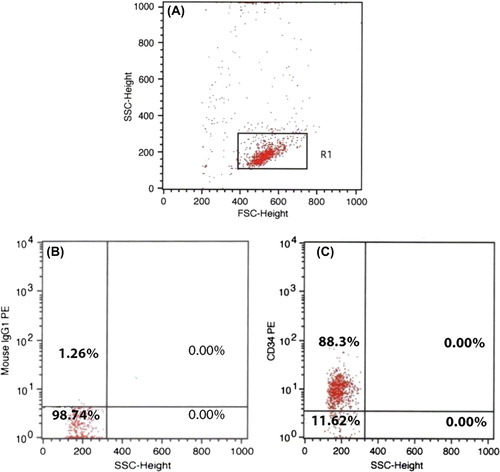
shows the HSPC fold expansion in 2-D and 3-D media after 7 days. The total expansion of the CD34+ cells on the aminated PES nanofiber and the conventional culture was 17.69-fold and 9.8-fold, respectively. There was a significant increase (1.8-fold) in the number of PES-cultured cells compared to the control (p < 0.05).
Differentiation of the expanded CD34+ cells to MK lineage
To assess the effect of aminated PES nanofiber on the differentiation of CB-derived CD34+ cells to Mk lineage, the expression of CD41/61 markers was surveyed. TPO- stimulated cells were collected from both the conditions on different days of differentiation. The phenotype of CD34+ cells was steady on the first day of differentiation, and CD34 (87%) and CD38 (% 0.03) were evaluated.
When CD34+ cells were stimulated by TPO, the expression of CD41 and CD61 were increased gradually, but this level was higher in the cells grown on aminated PES than those on the 2-D medium. The average of CD41 and CD61 expression on different days of differentiation has been shown in . As shown by the data presented, the expression of Mk markers was significantly higher in PES-cultured cells compared to that in the control culture, on all days. The result of flow cytometric analysis of CD41 and CD61 markers on day-7 has been shown () (p < 0.05).
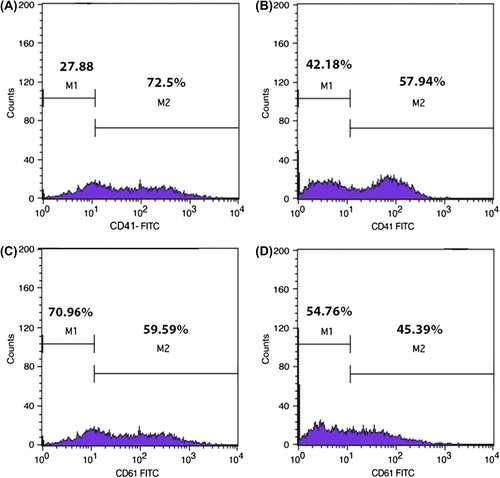
Table I. The percentage of CD41 and CD61- expressing cells in differentiation stage on conventional culture and aminated PES scaffold at different days.
Effects of aminated PES nanofibers on the expression of platelet-specific genes (GATA-1 and NF-E2)
To evaluate the development of the CD41/61+ cell population from hematopoietic cells, the mRNA expression of GATA-1 and NF-E2, along the megakaryopoietic pathway on two different days, and also on CD34+ cells as control, were calculated. Gene expression was measured by the real-time PCR method after normalization, according to the internal control gene expression (GAPDH).
The level of GATA-1 expression was diminished in the differentiated cells compared to CD34+ progenitors in both culture systems, without any significant difference between them (). In this study, we induced HSPC stimulation into Mk progenitors to the early differentiation stage. Consequently, the GATA-1 gene was found to express on the CD34+ cells remarkably, but it down-regulated through a differentiation/maturation trend and became undetectable. However, it was found that the GATA-1 gene promoter does not become silent.
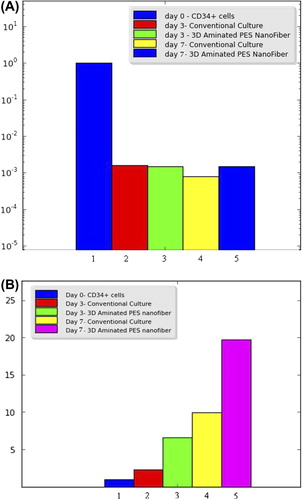
In parallel, the expression of NF-E2 mRNA was measured in differentiating megakaryocytes. The level of NF-E2 mRNA expression was gradually upregulated in the stage of megakaryocytic maturation (). All quantities were compared with the CD34+ cells (day 0). The expression of NF-E2 was found to be significantly higher in the cells cultured on aminated PES nanofiber than those grown in the conventional culture conditions during the early differentiation stages of Mk progenitors. As the results illustrate, the average level of NF-E2 gene expression was more than 2-fold in the cells that were differentiated in aminated PES media, compared to those that were cultured in the conventional condition (p < 0.05).
Discussion
Thrombocytopenia is one of the major draw backs after CB transplantation. The usage of UCB is limited in adults because of the low content of HSCs and Mk progenitors, even in one or two units (CitationLi et al. 2001), resulting in delayed platelet recovery in grafts of CB cells (CitationGluckman et al. 1989, CitationHofmeister et al. 2007). To overcome this limitation, 3-D culture environments have been developed, similar to the in vivo environmental niche. However, in order to create successful ex vivo expansion, HSCs needed be enriched, and the isolated CD34+ cells were expanded (CitationShivdasani 2001). Two-dimensional cultures were found to have restricted efficiency for the growth of hematopoietic progenitors. By contrast, 3-D cultures could function like the in vivo hematopoietic microenvironment by regulating cell fate (CitationLi et al. 2001). In this research, the effect of aminated PES nanofiber on the proliferation and differentiation of CD34+ progenitors toward Mk progenitors was investigated. We utilized the aminated PES nanofiber as the 3-D culture, accompanied by a serum-free StemSpan and cytokine cocktail. All processes were accomplished in parallel with the conventional system, which was carried out in the 2-D culture.
The number of expanded CD34+ cells was 1.8 times higher on the aminated PES nanofiber than those cultured on the conventional system in a 7-day expansion. HSPCs were found to proliferate more rapidly on the PES scaffold compared with 2-D culture. In similar research, the expansion of CD34+ cells was reported to be 3.5-fold higher on the aminated PES substrate than that on the commercial tissue culture polystyrene surface (TCPS) in a 10-day expansion. One feasible mechanism may be the trapping and enriching of certain protein ingredients of the medium by aminated nanofibers (CitationChua et al. 2006), and these components could affect the cell expansion (CitationKeselowsky et al. 2004, CitationWilson et al. 2005). Aminated PES nanofibers could possibly catch cytokines in the culture medium, fix to the supplemented growth factors (CitationChua et al. 2006), and act as a niche for hematopoietic stem cells (CitationCalvi et al. 2003, CitationZhang et al. 2003). The expression of CD34+ is diminished during the differentiation of HSPCs. The CD34 antigen is a highly sialylated and negatively charged glycophosphoprotein (CitationLanza et al. 2001). Another possible mechanism suggests that amine groups with a positive charge conjugated to the surface might adhere to the negatively charged CD34 molecule of the HSPC surface and lead to the activation of the downstream signaling pathways, enhancing cell proliferation (CitationChua et al. 2006).
The main goal of our assessment was to study the influence of aminated PES nanofibers in the additional induction of CD34+ cells into Mk progenitors. CD41, CD61 and + CD42 are distinctive markers for investigating cells committed to the Mk lineage, and the expression of CD41 (integrin αIIb) and CD61 (integrin β3) can describe Mk differentiation (CitationVainchenker Kieffer 1988, CitationDebili et al. 2001, CitationMathur et al. 2004). Glycoproteins IIb/IIIa (CD41/CD61), are detectable as receptors on the surface of all Mk lineage cells, and their expression increases during the maturation of cells (CitationRabellino et al. 1981, CitationLevene et al. 1985). Because the expression of CD42 is accrued after CD41 (CitationDebili et al. 1992), we evaluated the level of expression of CD41 and CD61. It was observed that the nanofiber scaffold could biomimic the microenvironmental niche of BM and develop the proliferation or differentiation of HSCs (CitationMa et al. 2008). Aminated nanofibers have been known to be most efficient in developing the expansion of the CD34+ and CD45+ cells and augmenting the HSPC-substrate adhesion, as compared to polystyrene culture media (CitationChua et al. 2006).
The number of CD41/61 expressing cells in nanofiber-coated plates was higher compared to the same population of cells in conventional plates at the differentiating stage. The substrate adhesion ability of CD34 + cells might be an explanation for the faster differentiation of these cells (CitationWright et al. 2001). Therefore, ex vivo 3-D systems were developed to mimic the in vivo hematopoietic niche. HSCs could not stay alive without appropriate growth factors in the medium. So, aminated PES surfaces might entrap and localize the differentiation-specific cytokines of platelet TPO and develop Mk progenitors from CD34+ cells. The amino groups bound to PES surface could probably affect the proliferation and differentiation of hematopoietic stem cells and play a supporting/synergistic role for the supplemented cytokines in the media (CitationChua et al. 2006).
GATA-1 and NF-E2, two of the important Mk transcriptional regulators, were required for Mk maturation/ differentiation (CitationShivdasani 2001). These factors, along with TPO, the principal growth factor of thrombopoiesis, participate in the completion of Mk maturation and platelet production (CitationShivdasani et al. 1995, CitationKostyak and Naik 2007). Thrombocytopenia could be the result of the absence or impairment of GATA-1 function, due to which Mk differentiation is arrested. The downregulation of GATA-1 in CD41/61+ cells compared to primary progenitors (CD34+ cells) prompted us to hypothesize that GATA-1 might have a light role in the differentiation of early Mk progenitors and overexpresses in the mid and/or terminal stage of Mk maturation. Our data did not exhibit any significant difference in the expression of GATA-1 gene in both the culture systems. However, our findings indicated that the NF-E2 mRNA expression level was increased during the maturation of Mk progenitors, suggesting a strong correlation between the NF-E2 mRNA and cell differentiation. We observed a higher level of NF-E2 gene expression in PES nanofiber-harvested cells compared with those harvested from the conventional conditions. NF-E2, one of the critical transcriptional factors regulating the expression of one or more target genes, involves Mk maturation and platelet generation (CitationShivdasani et al. 1995). Consequently, the aminated PES nanofiber itself is not sufficient to induce the proliferation and/or differentiation of HSCs, but provides a microenvironment like the BM niche, and supports and enriches cytokines, affecting the expansion or differentiation of the cells.
Conclusion
Aminated PES nanofibers could have a greater effect on the proliferation of CD34+ cells and their differentiation to Mk progenitors, than conventional culture. Therefore, injection of the expanded cells and differentiated Mk progenitors along with stem cell transplantation (from UCB) might accelerate the recovery of platelets and decrease the duration of thrombocytopenia after transplantation. However, this hypothesis needs further studies under in vivo conditions.
Acknowledgments
The authors thank Tabriz Blood Transfusion Headquarter for providing labratory facilities..
Declaration of interest
None of the authors have any conflicts of interest to declare.
This study was supported by the Blood Transfusion Research Center, High Institute for Research and Education in Transfusion Medicine, Tehran, Iran, and the Hematology Oncology Research Center, Tabriz University of Medical Sciences, Tabriz, Iran.
References
- Armitage JO. 1994. Bone marrow transplantation. N Engl J Med. 330:827–838.
- Calvi LM, Adams GB, Weibrecht KW, Weber JM, Olson DP, Knight MC, et al. 2003. Osteoblastic cells regulate the haematopoietic stem cell niche. Nature. 425:841–846.
- Chen TW, Yao CL, Chu IM, Chuang TL, Hsieh TB, Hwang SM. 2009. Large generation of megakaryocytes from serum-free expanded human CD34+ cells. Biochem Biophys Res Commun. 378: 112–117.
- Chua KN, Chai C, Lee PC, Tang YN, Ramakrishna S, Leong KW, Mao HQ. 2006. Surface-aminated electrospun nanofibers enhance adhesion and expansion of human umbilical cord blood hematopoietic stem/progenitor cells. Biomaterials. 27:6043–6051.
- Debili N, Issaad C, Massé JM, Guichard J, Katz A, Breton-Gorius J, Vainchenker W. 1992. Expression of CD34 and platelet glycoproteins during human megakaryocytic differentiation. Blood. 80: 3022–3035.
- Debili N, Robin C, Schiavon V, Letestu R, Pflumio F, Mitjavila-Garcia MT, et al. 2001. Different expression of CD41 on human lymphoid and myeloid progenitors from adults and neonates. Blood. 97: 2023–2030.
- Ehring B, Biber K, Upton TM, Plosky D, Pykett M, Rosenzweig M. 2003. Expansion of HPCs from cord blood in a novel 3D matrix. Cytotherapy. 5:490–499.
- Gluckman E, Broxmeyer HE, Auerbach AD, Friedman HS, Douglas GW, Devergie A, et al. 1989. Hematopoietic reconstitution in a patient with Fanconi's anemia by means of umbilical-cord blood from an HLA-identical sibling. N Engl J Med. 321:1174–1178.
- Hatami J, Andrade Pe Z, Bacalhau D, Cirurgião F, Ferreira CF, Cabral J, Silva C. 2014. Proliferation extent of CD34+ cells as a key parameter to maximize megakaryocytic differentiation of umbilical cord blood-derived hematopoietic stem/progenitor cells in a two-stage culture protocol. Biotechnology Reports. 4:50–55.
- Hofmeister CC, Zhang J, Knight KL, Le P, Stiff PJ. 2007. Ex vivo expansion of umbilical cord blood stem cells for transplantation: growing knowledge from the hematopoietic niche. Bone Marrow Transplant. 39:11–23.
- Ignatz M, Sola-Visner M, Rimsza LM, Fuchs D, Shuster JJ, Li XM, et al. 2007. Umbilical cord blood produces small megakaryocytes after transplantation. Biol Blood Marrow Transplant. 13:145–150.
- Keselowsky BG, Collard DM, García AJ. 2004. Surface chemistry modulates focal adhesion composition and signaling through changes in integrin binding. Biomaterials. 25:5947–5954.
- Koh LP, Rizzieri DA, Chao NJ. 2007. Allogeneic Hematopoietic Stem Cell Transplant Using Mismatched/Haploidentical Donors. Biol Blood Marrow Transplant. 13:1249–1267.
- Kostyak JC, Naik UP. 2007. Megakaryopoiesis: Transcriptional Insights into Megakaryocyte Maturation. Front Biosci. 12:2050–2062.
- LaIuppa JA, McAdams TA, Papoutsakis ET, Miller WM. 1997. Culture materials affect ex vivo expansion of hematopoietic progenitor cells. J Biomed Mater Res. 36:347–359.
- Lanza F, Healy L, Sutherland DR. 2001. Structural and functional features of the CD34 antigen: an update. J Biol Regul Homeost Agents. 15:1–13.
- Laughlin MJ. 2001. Umbilical cord blood for allogeneic transplantation in children and adults. Bone Marrow Transplant. 27:1–6.
- Lee CH, Shin HJ, Cho IH, Kang YM, Kim IA, Park KD, Shin JW. 2005. Nanofiber alignment and direction of mechanical strain affect the ECM production of human ACL fibroblast. Biomaterials. 26: 1261–1270.
- Levene RB, Lamaziere JM, Broxmeyer HE, Lu L, Rabellino EM. 1985. Human megakaryocytes. V. Changes in the phenotypic profile of differentiating megakaryocytes. J Exp Med. 161:457–474.
- Li Y, Ma T, Kniss DA, Yang ST, Lasky LC. 2001. Human cord cell hematopoiesis in three-dimensional nonwoven fibrous matrices: in vitro simulation of the marrow microenvironment. J Hematother Stem Cell Res. 10:355–368.
- Livak KJ, Schmittgen TD. 2001. Analysis of relative gene expression data using real-time quantitative PCR and the 2 (-Delta Delta C (T) Method. Methods. 25:402–408.
- Ma K, Chan CK, Liao S, Hwang W, Feng Q, Ramakrishna S. 2008. Electrospun nanofiber scaffolds for rapid and rich capture of bone marrow-derived hematopoietic stem cells. Biomaterials. 29:2096–2103.
- Mathur A, Hong Y, Wang G, Erusalimsky JD. 2004. Assays of megakaryocyte development: surface antigen expression,ploidy, and size. Methods Mol Biol. 272:309–322.
- Miyazaki R, Ogata H, Iguchi T, Sogo S, Kushida T, Ito T, et al. 2000. Comparative analyses of megakaryocytes derived from cord blood and bone marrow. Br J Haematol. 108:602–609.
- Nikougoftar Zarif M, Soleimani M, Abolghasemi H, Amirizade N, Abroun S, Kaviani S. 2011. The high yield expansion and megakaryocytic differentiation of human umbilical cord blood CD133+ cells. Cell Journal (Yakhteh). 13:173–178.
- Rabellino EM, Levene RB, Leung LL, Nachman RL. 1981. Human megakaryocytes. II. Expression of platelet proteins in early marrow megakaryocytes. J Exp Med. 154:88–100.
- Shivdasani RA, Rosenblatt MF, Zucker-Franklin D, Jackson CW, Hunt P, Saris CJ, Orkin SH. 1995. Transcription factor NF-E2 is requaired for platelet formation independent of the actions of TPO/MGDF in megakaryocyte development. Cell. 81:695–704.
- Shivdasani RA. 2001. Molecular and transcriptional regulation of megakaryocyte Differentiation. Stem cells. 19:397–407.
- Vainchenker W, Kieffer N. 1988. Human megakaryocytopoiesis: in vitro regulation and characterization of megakaryocytic precursor cells by differentiation markers. Blood Rev. 2:102–107.
- Wilson CJ, Clegg RE, Leavesley DI, Pearcy MJ. 2005. Mediation of biomaterial-cell interactions by adsorbed proteins: a review. Tissue Eng. 11:1–18.
- Wright DE, Wagers AJ, Gulati AP, Johnson FL, Weissman IL. 2001. Physiological migration of hematopoietic stem and progenitor cells. Science. 294:1933–1936.
- Zhang J, Niu C, Ye L, Huang H, He X, Tong WG, et al. 2003. Identification of the haematopoietic stem cell niche and control of the niche size. Nature. 425:836–841.