Abstract
The labeling of cells with nanomaterials for tumor detection is a very important part of various biomedical applications. In this study, multilayer nanocomposites were synthesized to achieve the multiple functions of fluorescence, magnetism, and bioaffinity. Firstly, superparamagnetic Fe3O4 nanoparticles were prepared as a magnetic core. Then, fluorescein isothiocyanate (FITC) was covalently linked to the surface of the silica-coated Fe3O4 core (designated FMNPs). Finally, bovine serum albumin (BSA) was conjugated onto the FMNPs (designated FMNPs-BSA). We also evaluated the feasibility and efficiency of labeling the human liver cancer cell line SMMC-7721 (SMMC-7721) with nanocomposites. SEM, hysteresis loop, EDS, FTIR, fluorescence spectra, and fluorescence microscopy were used to determine the physicochemical properties of nanocomposites. Fluorescence microscopy, SEM-EDS, and TEM were used to determine fluorescence labeling, absorption, and uptake respectively. The results showed that the nanocomposites obtained exhibited fine superparamagnetism, strong fluorescence, and good biological affinity. We succeeded in using the new multilayer nanocomposites to label cells, which had properties of magnetic targeting and fluorescent tracing.
Introduction
As one of the serious diseases endangering people's health, liver cancer has increased in incidence. Therefore, finding a valid approach to identify, track, and kill liver cancer cells has become our major concern.
In previous works, magnetic nanoparticles (MNPs) were modified and endowed with novel and necessary properties and were used in medical therapy (CitationDilnawaz et al. 2012, CitationGarcia-Bennett et al. 2010, CitationLundqvist et al. 2008, CitationIwasaki et al. 2010, Citationde Dios and Díaz-García 2010, CitationXu et al. 2005, CitationLu et al. 2004). The combined effects of the small size and superparamagnetism led to their use in labeling and sorting out cells (CitationLundqvist et al. 2004, CitationPamme and Wilhelm 2006, CitationYoon et al. 2006, CitationPerrin-Cocon et al. 1999, CitationIto et al. 2004), magnetic resonance imaging (CitationThiel et al. 1998, CitationSahu et al. 2012, CitationKohler et al. 2006, CitationBulte and Kraitchman 2004), targeted drug delivery (CitationRiviere et al. 2005, CitationChomoucka et al. 2010, CitationVeiseh et al. 2010, CitationYang et al. 2009), and hyperthermia in cancer therapy (CitationIto et al. 2005, CitationSharma and Chen 2009, CitationHergt et al. 2006). However, it is worth mentioning that the combination of controllability and labeling, which are two important and basic supporting points in the study of tumor cells, is of great significance. A challenge for future applications of nanomaterials is to find methods to integrate the functionalized nanomaterials into cells (CitationWidder et al. 1983, CitationWilhelm et al. 2002 and 2003). As we all know, some essential small molecules (such as amino acids, sugars, and ions) can traverse the plasma membrane with the action of integral membrane protein pumps or channels. However, macromolecules must be carried into the cell, in a process termed endocytosis. Endocytosis occurs by multiple mechanisms that fall into two broad categories, ‘phagocytosis’ and ‘pinocytosis’ (CitationConner and Schmid 2003). Recently, researchers expounded the method of interaction of nanocomposites with the cell membrane (CitationGu et al. 2010, CitationLuk et al. 1980). Synthetic multifunctional nanocomposites, which can be integrated into cells and subcellular structures, have been widely used to monitor cell position and function in vivo (CitationDilnawaz et al. 2012, CitationFeng et al. 2011, CitationKohler et al. 2005, CitationPisslaru et al. 2006, CitationArbab et al. 2004, CitationSarup et al. 1991). Moreover, cell therapy necessitates an efficient and non-invasive technique to monitor the cells’ behaviors. In this aspect, the additional fluorescence properties of nanocomposites offer a potential method for imaging in vitro and in vivo (CitationSun et al. 2010, CitationMi et al. 2010, CitationCorr et al. 2006, CitationSchafer et al. 2007, CitationFleige et al. 2002).
The objective of this study was to bio-label liver cancer cells with multifunctional nanocomposites. The MNPs of Fe3O4 (magnetic core) were first coated with silica shells by the hydrolysis of TEOS; FITC-APTES were then covalently linked with the silica-coated Fe3O4 to prepare bifunctional nanoparticles. As FMNPs were functionalized with amino groups, BSA could be easily immobilized on the FMNPs-NH2 by EDC and NHS. It was proven that the multilayer nanocomposites had stable properties of fluorescence, superparamagnetism, and biological affinity. We also conducted cell experiments to study interactions between particles and cells in vitro. As a result, the multilayer nanocomposites could be used as fluorescent probes to successfully label and image SMMC-7721 by absorption or uptake. Our research indicated that the multilayer nanocomposites might be applied for cancer treatment, such as magnetic drug targeting and cell imaging.
Materials
All chemicals were of analytical grade or of the highest purity available, and were used directly without any further purification. Iron (III) chloride hexahydrate (FeCl3·6H2O, 99.0%), iron (II) sulfate heptahydrate (FeSO4·7H2O, 99.0%), and trisodium citrate dehydrate (C6H5Na3O7·2H2O, 99.0%) were purchased from KeLong Chemical Co., Ltd. N-Hydroxysuccinimide (NHS, 98%), tetraethoxysilane (TEOS, 98%), and 3-amino-propyltriethoxysilane (APTES, 98%) were purchased from Alfa Aesar. Fluorescein isothiocyanate (FITC, 90%) was purchased from Chengdu Biochemical Reagent Co., Ltd. 1-(3-Dimethylamino- propyl)-3-ethylcarbodiimide hydrochloride (EDC, 98%) was obtained from Tokyo Kasei Kogyo Co., Ltd. RPMI-1640 was purchased from Hyclone. Standard fetal bovine serum (FBS) was purchased from Tianjin Hanyang Biologicals Technology Co., Ltd. SMMC-7721 was obtained from West China Hospital (Sichuan University). Other organic solvents used were of analytical reagent grades.
Experiments
Synthesis of Fe3O4 MNPs
The superparamagnetic nanoparticles were synthesized by the method of chemical co-precipitation. Typically, the solution of a mixture of FeCl3·6H2O (0.01 M), FeSO4·7H2O (0.006 M) and de-ionized water (50 mL) was treated using the Ultra Turrax for 5 min. The solution was heated to 50°C in an inert N2 atmosphere. Then, ammonia aqueous solution (1.5 M) was dropped into a three-necked flask with vigorous stirring until a pH of 10 was reached. Trisodium citrate dihydrate (0.5 g) was then added into the solution. After the reaction, the product obtained was separated by a magnet and further washed several times with deionized water and ethanol. The resulting MNPs were stored in anhydrous ethanol at room temperature.
Synthesis of FITC-APTES
FITC (9.2 mg) and APTES (50 uL) were added into deionized water (5 mL), and then the solution was stirred in the dark in an inert N2 atmosphere, overnight. The resulting FITC-APTES was stored in a dark place for later use.
Synthesis of fluorescent magnetic nanoparticles (FMNPs)
MNPs (13 mg, 1 mL) were dispersed in an ethanol-water mixture (100 mL, ethanol/water = 4/1, v/v). The mixture was homogenized by Ultra Turrax (30 min) and by ultrasonic cell crusher noise isolating chamber (30 min). Then, ammonia solution (0.8 mL, 25 wt%) was added as a catalyst, and the reacted mixture was vigorously stirred at room temperature. TEOS (0.14 mL) was added 30 min later, and the reaction proceeded for 4 h. FITC-APTES (0.3 mL) was then added into the mixture and stirred for another 1 h. TEOS (0.80 mL) was added dropwise for 2 h, and then stirred vigorously overnight. After the reaction, the product obtained was separated by a magnet and further washed several times with deionized water and ethanol. The resulting FMNPs were stored in anhydrous ethanol at 4°C in a dark place.
Synthesis of fluorescent magnetic nanocomposites grafted with BSA (FMNPs-BSA)
The amine group was immobilized on FMNPs by using APTES. FMNPs (0.3 g) were dispersed in a mixture of ethanol and water (105 mL, ethanol/water = 20/1, v/v). APTES (0.5 mL) was added dropwise into the solution in 1 h with vigorous stirring. The mixture was vigorously stirred at 50°C for 3 h. After that, FMNPs-NH2 were separated by a magnet and washed several times with deionized water and ethanol. The resulting FMNPs-NH2 were stored in anhydrous ethanol in a dark place for later use.
Next, the BSA was immobilized on the FMNPs-NH2 by EDC/NHS. Firstly, BSA (1.6 mg) was dispersed in phosphate-buffered solution (PBS, pH = 4.8, 2 mL) under an ice-salt bath. EDC (0.64 mg) and NHS (0.96 mg) were added into the PBS solution for 40 min with gentle shaking. Then FMNPs-NH2 (2 mg) was added into the above solution at room temperature for 4 h. After the reaction, the product obtained was separated by a magnet and further washed several times with ethanol and PBS. The resulting FMNPs-BSA were stored in PBS solution at 4°C in a dark place.
Incubation of the liver cancer cell with the multifunctional nanocomposites
MNPs, FMNPs, and FMNPs-BSA were sterilized using a filter membrane (0.22 μm). SMMC-7721 were cultured with the above nanocomposites (about 0.5 mg mL− 1) in RPMI-1640 medium with 10% FBS for 72 h under conditions of 100% humidity, 5% CO2, and 37°C. After that, the cells were extensively washed with PBS (pH = 7.4), fixed with glutaraldehyde (2.5 wt%), and processed for analysis.
Results and discussion
Structural design of the multilayer nanocomposites
A schematic illustration of the multilayer nanocomposites is shown in . The Fe3O4 superparamagnetic nanoparticles were synthesized as a magnetic core. In order to avoid fluorescence quenching of FITC and aggregation of nanoparticles, the Fe3O4 nanoparticles were coated with a silicon oxide layer, and the silicon oxide layer was formed with TEOS via a hydrolysis reaction. In the experiments, we found that if free FITC was encapsulated in the silica shell, FITC would leak into the solution because of its water solubility. To resolve this issue, FITC was covalently linked with APTES by the reaction of addition of a carbon-nitrogen double bond, where APTES was a coupling agent. Hence, we synthesized FITC-APTES before the synthesis of FMNPs with good fluorescence stability. Silanization took place on the surfaces bearing hydroxyl groups of the silicon oxide layer in the organic solvent, where APTES was a coupling agent. In order to avoid fluorescence leakage, the FMNPs were also coated with the silicon oxide layer. APTES was then added and hydrolyzed onto the surface to provide active NH2 groups. BSA was immobilized with the amino group of APTES by EDC/NHS.
The prepared multilayer nanocomposites had three main properties: magnetism, fluorescence, and biocompatibility. Nanocomposites with a magnetic core could be moved to the desired area under the influence of an external magnetic field, with FITC, which could be detected by fluorescence microscope, and BSA, which could enhance their biocompatibility.
Characterization of multilayer nanocomposites
In order to obtain more direct information on size and morphology of the nanoparticles, the magnetite particles were analyzed by SEM (JSM-7500F, JEOL). From the SEM images of MNPs, FMNPs, and FMNPs-BSA (), it could be seen that they were all quasi-spherical. The average diameter of MNPs was about 30 nm, and 80% of them were 20–40 nm (). As indicated in , the average size of FMNPs was about 100 nm, and 80% of them were 75–125 nm. The average diameter of FMNPs-BSA was about 210, nm and 80% of them were 180–260 nm.
The elements of nanocomposites were analyzed by SEM-EDS (JEOL JEM-7500F scanning electron microscopy). shows the obvious peaks of some significant elements. The peaks of Fe and O were observed in the curve of MNPs, which corresponded to the elements of Fe3O4. The peaks of N and Si were observed in the curve of FMNPs and FMNPs-BSA. The two sources of the Si element were TEOS and APTES, for FMNPs and FMNPs-BSA. The sources of the N element were FITC and APTES for FMNPs. Other than that, BSA was also one of the N sources for FMNPs-BSA. The Fe weight percentage (wt%) of MNPs was 74.8, while the Fe wt% of FMNPs was 11.8, and the Fe wt% of FMNPs-BSA was 4.09. The N wt% of FMNPs was 1.46 and the N wt% of FMNPs-BSA was 3.01. The Fe wt% showed a gradual decrease while N wt% showed a gradual increase, which corresponded to the change of structure. The Fe3O4 superparamagnetic nanoparticles were synthesized as a magnetic core, so the Fe wt% was very high. The surface of the particles was covered by SiO2, APTES, and BSA, in that order. EDS was an analytical method of detecting elements on the surface of materials. The changes in the elements detected were consistent with the changes of the surface structure of nanocomposites. With the addition of sources of the N element, the N wt% became higher. For an interpretation of the structural changes, please see the section on structural design of multilayer nanocomposites.
Magnetic characterization of MNPs, FMNPs, and FMNPs-BSA was carried out using the MPMS XL-7 superconducting quantum interference device (SQUID) magnetometer at 300 K. shows that the hysteresis loops of MNPs, FMNPs, and FMNPs-BSA had no hysteresis, and were completely reversible. The magnetization increased rapidly and reached saturation at a low magnetic field, which fully proved the existence of the ferromagnetic characteristic. However, the saturation magnetic moments of FMNPs and FMNPs-BSA reached 6.0 emu g−1 and 2.9 emu g−1 respectively. These saturation magnetization values (saturation magnetization, Ms) were far lower than that of the Fe3O4 (70 emu g−1) used. The proportion of nonmagnetic materials of unit mass had greatly increased because of the addition of the nonmagnetic substances (TEOS, APTES, FITC and BSA) onto the surface of the MNPs. As can be seen from , all of these nanoparticles exhibited a superparamagnetic property.
Infrared spectrograms of MNPs, FMNPs, and FMNPs-BSA were obtained using a Nicolet-6700 FT-IR spectrometer (Thermo Scientific, U.S.). In , it can be seen that the significant peaks of MNPs were at around 636 and 545 cm−1. These bands were a result of the split v1 band at 570 cm−1, which corresponded to the Fe–O bond of bulk magnetite (CitationChenevier et al. 2000, CitationKreisel et al. 1998). Furthermore, an adsorption band was observed at around 399 cm−1, which corresponded to the shifting of the v2 band of the Fe–O bond of bulk magnetite (at 375 cm−1) to a higher wavenumber. The absorption bands at 3430 cm−1 in resulted from the − OH vibration on the surface of magnetite (CitationMa et al. 2003). The significant peaks of FMNPs at 3405 cm−1 and 1618 cm−1 were due to the N–H stretching vibration and the NH2 bending mode of the free NH2 group, respectively (). In , the peak at 1082 cm−1was assigned to the Si-O-H and Si–O–Si groups. The absorption bands at 964 cm−1 and 797 cm−1 were related to the vibrations of Si–O–H and − OH. Comparing the FTIR spectra of FMNPs with FMNPs-BSA (), the appearance of new absorption bands at 3405 cm− 1, 1654 cm−1, and 1582 cm−1 could be ascribed to N–H and C = O stretching vibrations, which could prove the successful grafting of BSA on the surface using the linking agent (EDC/NHS). The presence of the anchored methylene group was confirmed by C–H stretching vibrations that appeared at 2976 cm−1, as seen in (CitationYamaura et al. 2004).
The fluorescence spectra of the nanoparticles were obtained by using the Cary Eclipse fluorescence spectrophotometer (Varian, U.S.). illustrates the spectra of FITC, FMNPs, and FMNPs-BSA. Free FITC had broad emission spectra, from 510 nm to 600 nm, and the peak of the emission bands occurred at 520 nm. When the emission wavelength was 520 nm, the excitation spectra occurred at 420–520 nm and the peak of the excitation band appeared at 495 nm, as seen in . These characteristic data were similar to those of the previous studies (CitationLundqvist et al. 2004). In , the emission peak was 521 nm and the excitation peak was 498 nm. In , these two bands shifted to 520 and 500 nm respectively. Compared with FITC, the small shift of the bands for FMNPs and FMNPs-BSA were due to the influence of the amino groups and amide groups respectively. Hence, it was concluded that the surface modification and grafting did not affect the fluorescence characteristics significantly.
Labeling of SMMC-7721 in vitro
The magnetic response and fluorescence photographs of nanocomposites were obtained using a ShunYu XD30-RFL instrument, under an external magnetic field. From , it is evident that the movement of bright green FMNPs and FMNPs-BSA could easily be observed. They moved fast to the right magnet in a short time. These experimental results revealed that nanocomposites have good magnetic response.
Figure 7. Fluorescence microscopy images of (a) FMNPs, and (b) FMNPs-BSA moving due to the application of the external magnetic field; the yellow arrow indicated the direction of the external magnetic field.
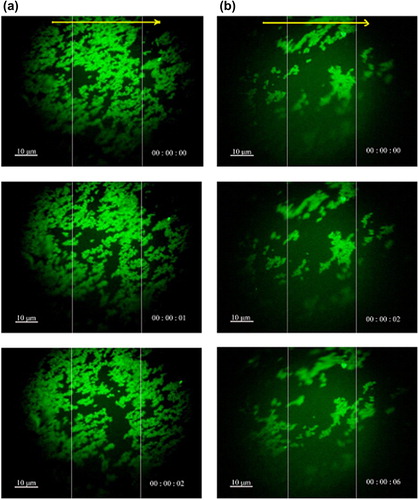
The prepared MNPs, FMNPs and FMNPs-BSA were sterilized by membrane filtration (0.22 μm). Cells of the human liver cancer cell line SMMC-7721 (SMMC-7721) )were cultured for 72 h with MNPs, FMNPs, and FMNPs-BSA, respectively. The cells were washed immediately to remove the unbound particles. shows the optical microscopy image of the untreated SMMC-7721 as control group, and the shape of SMMC-7721 can be seen to be irregular. and show the optical microscopy images of the SMMC-7721 after incubation with MNPs. The MNPs existed in two main forms: individual particles and aggregate particles. As seen in , almost all the MNPs gained entry into SMMC-7721 (the red narrow heads). The cells became round in shape after incubation with MNPs. The absorption and uptake by SMMC-7721 could be observed clearly, as shown in . Hence, some MNPs were swallowed into cells (the red arrowheads), and some MNPs were absorbed on the surface of cells (the yellow arrowheads).
Figure 8. (a) Microscopy image of the untreated SMMC-7721; Microscopy images of SMMC-7721 after incubation with (b-1 and b-2) MNPs.
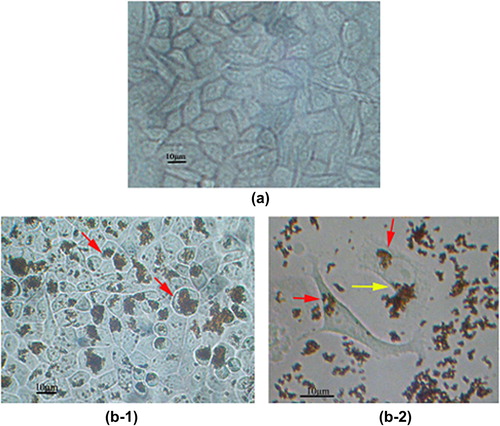
In , the left column (a) shows the microscopy images of the SMMC-7721 after incubation with FMNPs and the right column (b) shows those with FMNPs-BSA. The first two different images showed, respectively, images of the SMMC-7721 and particles that were analyzed using the optical microscope; and the fluorescence microscope. The last image presents an overlap of the two channels. (I) shows that the shape of SMMC-7721 was irregular. In (II), some bright green particle clusters were observed. In (III), we see that the bright green clusters corresponding to particles in (I) were mostly localized in the same position. It also shows that some particles were swallowed into cells or absorbed on the surface of cells. So the cells were successfully labeled with sites of enhanced fluorescence, presumably due to clusters of FMNPs and FMNPs-BSA respectively.
Cellular absorption or uptake
The untreated SMMC-7721 was spindle shaped and had a smooth surface, as presented in . Compared with the untreated cells, the surfaces of cancer cells after incubation with MNPs, FMNPs and FMNPs-BSA were tougher, and their shapes rounder. The phenomenon, mainly due to the expansion of the endoplasmic reticulum, swelling and collapse of most of the mitochondria, expansion of nuclear space, and shrinkage of nuclear envelope breeds (CitationHu et al. 2005).
The elements of the cell surface were analyzed by SEM-EDS (JEOL JEM-7500F scanning electron microscopy). Compared with the characteristic peaks of the Fe element (), showed that the Fe element was also detected and the Fe wt% gradually decreased. The percentage of Fe was zero in the untreated cells (). The percentage of Fe was 5.75% after incubation with MNPs (), 1.51% after incubation with FMNPs (), and 1.30% after incubation with FMNPs-BSA (). The Fe element that came from the nanocomposites was detected, which indicated that SMMC-7721 could absorb MNPs, FMNPs and FMNPs-BSA.
Figure 10. SEM-EDS image of the untreated (a) SMMC-7721; SEM-EDS images of SMMC-7721 after incubation with (b) MNPs, (c) FMNPs, and (d) FMNPs-BSA.
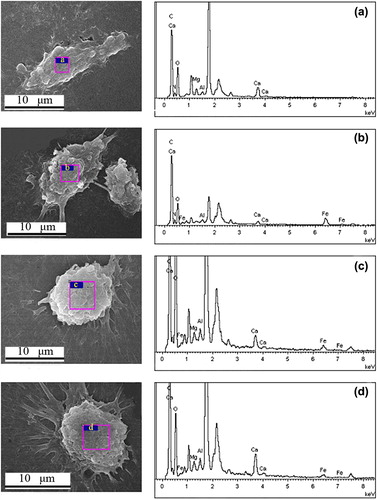
shows the TEM images of SMMC-7721 after incubation with nanocomposites, obtained using a Tecnai G2F20 S-TWIN (FEI, U.S.).
Figure 11. TEM image of the untreated (a) SMMC-7721; TEM images of SMMC-7721 after incubation with (b) MNPs, (c) FMNPs, and (d) FMNPs-BSA.
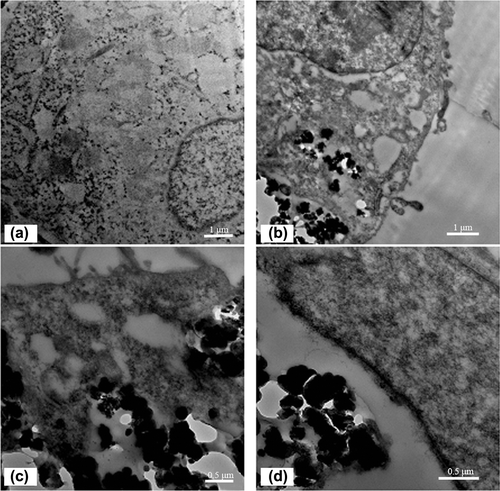
In the control group, several scattered vacuoles were observed in the untreated SMMC-7721 cells, which might be the result of expansion of the endoplasmic reticulum (). In the experimental group, MNPs, FMNPs, and FMNPs-BSA were observed in the cells. Nanocomposites were not observed in the cell nucleus. The MNPs, FMNPs, and FMNPs-BSA aggregated into irregular clusters respectively. Compared with the untreated cells (), nanocomposites appeared in the cytoplasm (, , and ), which indicated that the SMMC-7721 could ingest MNPs, FMNPs and FMNPs-BSA.
Conclusions
Our study showed that the prepared MNPs, FMNPs and FMNPs-BSA had been absorbed or swallowed by SMMC-7721. MNPs, FMNPs and FMNPs-BSA, which had a size in the nanometer range and good magnetic response, were able to reach the specific location in the presence of an external magnetic field. FMNPs and FMNPs-BSA, which have been described in this study, were employed for cell tracking.
The results reported here are expected to pave the way for further tailoring of multifunctional nanocomposites used in medical therapy. What is worth mentioning is that the prepared FMNPs were useful carrier models for drug delivery and cell imaging. The preparation of FMNPs-BSA provides a method for grafting other proteins or drugs onto FMNPs. Moreover, the BSA can also be combined with drugs. Therefore, such kinds of multilayer nanocomposites may play an important role in metabolism and drug delivery.
Acknowledgements
This work is supported by National Natural Science Foundation of China (NO. 20975072). The authors thank the Instrumental Analysis Center of Sichuan University for the characterization of products. They would also like to thank the Biochemistry and Molecular Biology Departments of Sichuan University for the incubation of cells, and Prof. Hui Li for the use of the FT-IR spectrometer and fluorescence spectrophotometer.
Declaration of interest
The authors report no declarations of interest. The authors alone are responsible for the content and writing of the paper.
References
- Arbab AS, Jordan EK, Wilson LB, Yocum GT, Lewis BK, Frank JA. 2004. In vivo trafficking and targeted delivery of magnetically labeled stem cells. Hum Gene Ther. 15:351–360.
- Bulte JW, Kraitchman DL. 2004. Iron oxide MR contrasts agents for molecular and cellular imaging. NMR Biomed. 17:484–499.
- Chenevier P, Veyret B, Roux D, Henry-Toulme N. 2000. Interaction of cationic colloids at the surface of J774 cells: a kinetic analysis. Biophys J. 79:1298–1309.
- Chomoucka J, Drbohlavova J, Huska D, Adam V, Kizek R, Hubalek J. 2010. Magnetic nanoparticles and targeted drug delivering. Pharmacol Res. 62:144–149.
- Conner SD, Schmid SL. 2003. Regulated portals of entry into the cell. Nature. 422:37–44.
- Corr SA, Byrne AO’, Gun'ko YK, Ghosh S, Brougham DF, Mitchell S, et al. 2006. Magnetic-fluorescent nanocomposites for biomedical multitasking. Chem Commun.4474–4476.
- Dilnawaz F, Singh A, Mewar S, Sharma U, Jagannathan NR, Sahoo SK. 2012. The transport of non-surfactant based paclitaxel loaded magnetic nanoparticals across the blood brain barrier in a rat model. Biomaterials. 33:2936–2951.
- de Dios AS, Díaz-García ME. 2010. Multifunctional nanoparticles: analytical prospects. Anal Chim Acta. 666:1–22.
- Feng Y, Jin X, Dai G, Liu J, Chen J, Yang L. 2011. In vitro targeted magnetic delivery and tracking of superparamagnetic iron oxide particles labeled stem cells for articular cartilage defect repair. J Huazhong Univ Sci Technol. 31:204–209.
- Fleige G, Seeberger F, Laux D, Kresse M, Taupitz M, Pilgrimm H. 2002. In vitro characterization of two different ultrasmall iron oxide particles formagnetic resonance cell tracking. Invest Radiol. 37: 482–488.
- Garcia-Bennett A, Nees M, Fadeel B. 2010. In search of the Holy Grail: Folate-targeted nanoparticles for cancer therapy. Biochem Pharmacol. 81:976–984.
- Gu N, Li Y. 2010. Interaction of nanoparticles on cell membranes. Acta Bioch Bioph Sin. 26:623–637.
- Hergt R, Dutz S, R Müller, Zeisberger M. 2006. Magnetic particle hyperthermia: nanoparticle magnetism and materials development for cancer therapy. J Phys: Condens Matter. 19:2919–2934.
- Hu S, Li S, Yan Y, Wang Y, Cao X. 2005. Change of hepatoma cells in ultrastructure after inorganic crystal nanoparticles uptaken. J Wuhan Univ Technol. 27:21–23.
- Ito A, Hibino E, Kobayashi C, Terasaki H, Kagami H, Ueda M. 2005. Construction and delivery of tissue-engineered human retinal pigment epithelial cell sheets, using magnetite nanoparticles and magneticforce. Tissue Eng. 11: 489–496.
- Ito A, Takizawa Y, Honda H, Hata K, Kagami H, Ueda M. 2004. Tissue engineering using magnetite nanoparticles and magnetic force: heterotypiclayers of cocultured hepatocytes and endothelial cells. Tissue Eng. 10:833–840.
- Iwasaki T, Kosaka K, Watano S, Yanagida T, Kawai T. 2010. Novel environmentally friendly synthesis of superparamagnetic magnetite nanoparticles using mechanochemical effect. Mater Res Bull. 45:481–485.
- Kohler N, Sun C, Fichtenholtz A, Gunn J, Fang C, Zhang M. 2006. Methotrexate-immobilized poly (ethylene glycol) magnetic nanoparticles for MR imaging and drug delivery. Small. 2:785–792.
- Kohler N, Sun C, Wang J, Zhang M. 2005. Methotrexate-modified superparamagnetic nanoparticles and their intracellular uptake into human cancer cells. Langmuir. 21:8858–8864.
- Kreisel J, Lucazeau G, Vincent H. 1998. Raman spectra and vibrational analysis of BaFe12O19 hexagonal ferrite. J Solid State Chem. 137: 127–137.
- Lu HC, Yi GS, Zhao SY, Chen DP, Guo LH, Chend J. 2004. Synthesis and characterization of multi-functional nanoparticles possessing magnetic, up-conversion fluorescence and bio-affinity properties. J Mater Chem. 14:1336–1341.
- Luk KH, Hulse RM, Phillips LT. 1980. Hyperthermia in cancer therapy. West J Med. 132:179–185.
- Lundqvist M, Sethson I, Jonsson BH. 2004. Protein adsorption onto silica nanoparticles: conformational changes depend on the particles'curvature and the protein stability. Langmuir. 20: 10639–10647.
- Lundqvist M, Stigler J, Elia G, Lynch I, Cedervall T, Dawson KA. 2008. Nanoparticle size and surface properties determine the protein corona with possible implications for biological impacts. Proc Natl Acad Sci USA. 105:14265–14270.
- Ma M, Zhang Y, Yu W, Shen HY, Zhang HQ, Gu N. 2003. Preparation and characterization of magnetite nanoparticles coated by aminosilane. Coll Surf A Physio Chem Eng Aspects. 212:219–226.
- Mi C, Zhang J, Gao H, Wu X, Wang M, Wu Y, et al. 2010. Multifunctional nanocomposites of superparamagnetic (Fe3O4) and NIR-responsive rare earch–doped up-conversion fluorescent (NaYF4: Yb, Er) nanoparticles and their applications in biolabeling and fluorescent imaging of cancer cells. Nanoscale. 2:1141–1148.
- Pamme N, Wilhelm C. 2006. Continuous sorting of magnetic cells via on-chip free-flow magnetophoresis. Lab Chip. 6:974–980.
- Perrin-Cocon LA, Marche PN, Villiers CL. 1999. Purification of intracellular compartments involved in antigen processing: a new method based on magnetic sorting. Biochem J. 338:123–130.
- Pisslaru SV, Harbuzariu A, Gulati R, Witt T, Sandhu NP, Simari RD. 2006. Magnetically targeted endothelial cell localization in stented vessels. J Am Coll Cardiol. 48:1839–1845.
- Riviere C, Boudghene FP, Gazeau F, Roger J, Pons JN, Laissy JP, et al. 2005. Iron oxide nanopartice-labeled rat smooth muscle cells: cardiac MR imaging for cell graft monitoring and quantitation. Radiology. 235:959–967.
- Sahu SK, Maiti S, Pramanik A, Ghosh SK, Pramanik P. 2012. Controlling the thickness of polymeric shell on magnetic nanoparticles loaded with doxorubicin for targeted delivery and MRI contrast agent. Carbohyd Polym. 87:2593–2604.
- Sarup JC, Johnson RM, King KL, Fendly BM, Lipari MT, Napier MA, et al. 1991. Characterization of an anti-p185HER2 monoclonal antibody that stimulates receptor function and inhibits tumor cell growth. Growth Regul. 1:72–82.
- Schafer R, Kehlbach R, Wiskirchen J, Bantleon R, Pintaske J, Brehm BR. 2007. Transferrin receptor upregulation: in vitro labeling of rat mesenchymal stem cells with superparamagnetic iron oxide. Radiology. 244: 514–523.
- Sharma R, Chen CJ. 2009. Newer nanoparticles in hyperthermia treatment and thermometry. J Nanopart Res. 11:671–689.
- Sun P, Zhang H, Liu C, Fang J, Wang M, Chen J, et al. 2010. Preparation and characterization of Fe3O4/CdTe magnetic/fluorescent nanocomposites and their applications in immuno- labeling and fluorescent imaging of cancer cells. Langmuir. 26: 1278–1284.
- Thiel A, Scheffold A, Radbruch A. 1998. Immunomagnetic cell sorting–pushing the limits. Immunotechnology. 4:89–96.
- Veiseh O, Gunn JW, Zhang M. 2010. Design and fabrication of magnetic nanoparticles for targeted drug delivery and imaging. Adv Drug Deliv Rev. 62:284–304.
- Widder KJ, Morris RM, Poore GA, Howard DP, Senyei AE. 1983. Selective targeting of magnetic albumin microspheres containing low-dose doxorubicin: total remission in Yoshida sarcoma-bearing rats. Eur J Cancer Clin Oncol. 19: 135–139.
- Wilhelm C, Billotey C, Roger J, Pons JN, Bacri JC, Gazeau F. 2003. Intracellular uptake of anionic superparamagnetic nanoparticles as a function of their surface coating. Biomaterials. 24:1001–1011.
- Wilhelm C, Gazeau F, Roger J, Pons JN, Bacri JC. 2002. Interaction of anionic superparamagnetic nanoparticles with cells: kinetic analyses of membrane adsorption and subsequent internalization. Langmuir. 18:8148–8155.
- Xu XQ, Shen H, Xu JR, Xu J, Li XJ, Xiong XM. 2005. Core-shell structure and magnetic properties of magnetite magnetic fluids stabilized with dextram. Appl Surf Sci. 252:494–500.
- Yamaura M, Camilo RL, Sampaio LC, Macêdoc MA, Nakamurad M, Tomad HE. 2004. Preparation and characterization of (3-aminopropyl) triethoxysilane-coated magnetite nanoparticles. J Magn Magn Mater. 279:210–217.
- Yang P, Quan Z, Hou Z, Li C, Kang X, Cheng Z, Lin J. 2009. A magnetic, luminescent and mesoporous core-shell structured composite material as drug carrier. Biomaterials. 30:4786–4795.
- Yoon TJ, Yu KN, Kim E, Kim JS, Kim BG, Yun SH, et al. 2006. Specific targeting, cell sorting, and bioimaging with smart magnetic silica core-shell nanomaterials. Small. 2:209–215.