Abstract
To study the effects of Wharton's jelly cells (WJCs) on acute spinal cord injury (SCI), 81 rats were divided into a sham surgery group, a model group, and a WJC transplantation group (n = 27). Motor functions of the model and WJC transplantation groups were partially recovered, and the recovery was better in the latter group. The WJC transplantation group had integral spinal cord tissues. Compared with the model group, the WJC transplantation group expressed significantly less interleukin-1β (IL-1β) and more nerve growth factor (NGF) (P < 0.05). WJC transplantation changed the microenvironment of the SCI site, inhibited IL-1β expression, increased NGF expression, promoted the recovery of neurological function, and relieved secondary SCI.
Introduction
Generally, spinal cord injury (SCI), as devastating damage to the central nervous system, temporarily injures the blood-brain barrier, induces neutrophils and monocytes/macrophages to enter the central nervous system, releases inflammatory factors, triggers a local severe immune response, and leads to secondary SCI (CitationOyinbo 2011). Stem cells, which are capable of self-renewal and multi-directional differentiation, offer promise for SCI therapy. Expressions of inflammatory factors are increased post-SCI. Interleukin-1β (IL-1β) plays an important role, as a cytokine, in systemic or local responses to infection and injury. IL-1β also mainly mediates chronic or acute inflammatory response. IL-1β expression is upregulated at the site of injury, thus aggravating SCI (CitationWang et al. 1997). Nerve growth factor (NGF) mainly facilitates the differentiation and maturation of sympathetic and sensory nerve cells during development, maintains normal neuronal function, and induces the growth of neurites towards nerve fibers. Therefore, it is of great significance to control local inflammatory response and to increase NGF expression after SCI, for better recovery. Although mesenchymal stem cells and Wharton's jelly cells (WJCs) of the human umbilical cord are conducive to SCI recovery (CitationRyu et al. 2012, CitationOliveri et al. 2014), the detailed mechanism remains unclear. In this study, we detected the changes in IL-1β and NGF expression in the spinal cord tissues of SCI rats after transplantation of WJCs, aiming to explore the possible mechanism of WJC transplantation therapy.
Experimental
Materials
Eighty-one healthy adult female SD rats (190–210 g) were provided by the experimental animal center of our hospital and bred under sterile conditions. Umbilical cord tissues, which showed negative results in the detection of infection-related indices, were donated by female volunteers who underwent normal Cesarean section. This study has been approved by the ethics committee of our hospital. The reverse transcription kit, real-time fluorescent quantitative kit, and Trizol reagent were purchased from TaKaRa (Japan). Primers were designed by Sangon Biotech (Shanghai) Co., Ltd. Western blot-related kits were bought from Life Technologies (USA). Rabbit anti-rat IL-1β and NGF antibodies were obtained from Santa Cruz (USA).
Isolation, culture, and identification of WJCs
Fresh umbilical cord, from which arteries, veins and surface film were removed, was rinsed with normal saline. Wharton's jelly tissues were scissored into 1.0–1.5 mm3 blocks, transferred into a 75 mL cell culture flask, and cultured in 6 mL of DMEM/F12 culture medium containing 10% (v/v) fetal bovine serum and 100 U/mL penicillin and streptomycin (double antibody) at 37°C in a 5% CO2 incubator. The culture medium was refreshed 3 d later, and then once every 2–3 d. When 80–90% of the cells grew to adherence, they were passaged after trypsin digestion, and the culture medium was refreshed once every 2–3 d. Surface antigens of the 3rd or 4th generation cells were detected with flow cytometry.
Establishment of SCI rat model
Rats were anesthetized by intraperitoneal injection with 10% chloral hydrate (v/v) (4 mL/kg), shaved to expose the surgical site, fixed on a stereotaxic apparatus, sterilized, and covered with surgical drape. With the first spinous process as the center, the skin of the back was incised (about 2 cm) in the middle, and subcutaneous mucosa was separated by blunt dissection. Subsequently, the tendon of the paraspinal muscle was cut off along the midline to completely expose the left first spinous process, vertebral plate, and vertebral arch. After the spinous process was removed with a pair of small curved forceps under a microscope, the vertebral plate until the central vertebral body was removed along the broken end of the spinous process, and the left vertebral arch until the pedicle was removed vertically, forming an L-shaped bone window (scar width: 1 mm). Finally, the posterior midline cleft of the L3 vertebra was exposed with an ophthalmic scalpel and subjected to left semi-transection. After hemostasis with gelatin sponge, the incised muscle and skin without bleeding were sutured layer-by-layer. After surgery, 200000 U of penicillin was subcutaneously or intramuscularly injected to observe the respiratory changes.
Animal grouping
The rats were divided into a sham surgery group (preparation of L-shaped bone window and then layer-by-layer suturing), a model group (preparation of L-shaped bone window and then semi-transection), and a WJC transplantation (transplantation of 1 × 106 WJCs through the femoral vein after semi-transection) group.
Recovery of motor function
Six rats were randomly selected from each group, the motor functions of which were evaluated with the Basso, Beattie, Bresnahan (BBB) scale (CitationBasso et al. 1995) on postoperative days 1, 3, 7, 14, 21 and 28, in a double-blind manner.
Observation of spinal ultrastructural changes
The above rats were sacrificed under anesthesia 28 d after surgery, and their spinal cord tissues were prepared into ultrastructure sections, stained, and observed by the pathological department of our hospital. Meanwhile, expression of NGF mRNA and protein in the fresh tissues were also detected.
Detection of IL-1β and NGF mRNA expression in spinal cord tissues
Three of the remaining rats in each group were selected for detection of IL-1β mRNA expression at each time point (0, 3, 6, 12, 24, 72 and 168 h). After being anesthetized, the rats were fixed on the operating table, and their spinal cord tissues were separated under sterile conditions. With the injury site as the center, 3 cm of the vertebral body was disconnected, washed with pre-cooled normal saline, and stored in liquid nitrogen prior to use. Total mRNA was extracted by using the Trizol-based method and reverse-transcribed into cDNA with GAPDH as the internal reference. According to the kit's instructions, real-time fluorescent quantitative PCR was performed with the Fast 7500 Real Time System at 95°C for 30 s, 95°C for 3 s, and 60°C for 30 s, 40 cycles in total. Each experiment was conducted in triplicate. Amplification and melting curves were plotted after PCR. Relative mRNA expression level = 2−ΔΔCT, with the mean value recorded. NGF mRNA expressions were detected 28 d after surgery with the same method. Primer sequences: Upstream primer of IL-1β: 5’-CACCTTCTTTTCCTTCATCTTTG-3’, downstream primer: 5’-GTC CTTGCTTGTCTCTCCTTGTA-3’; upstream primer of NGF: 5’-CTGCTGAACCAATAGCTGCCCG-3’, downstream primer: 5’-CGCCTTGACAAAGGTGTGAGT CG-3’; upstream primer of GAPDH: 5’-CCTCAAGATTGTCAGCAAT-3’, downstream primer: 5’-CCATCCACAGTCTTCTGAGT-3’.
Detection of IL-1β and NGF protein expression in spinal cord tissues
Spinal cord tissues were lysed with RIPA buffer and centrifuged at 12000 r/min and 4°C for 10 min, from which the supernatant was collected and stored at − 20°C. Protein concentrations were detected by the BCA methods, and the proteins were subjected to polyacrylamide gel electrophoresis. The product was then transferred onto a membrane that was blocked in 50 g/L skimmed milk 1.5 h later at room temperature for 1 h, incubated overnight after additions of primary antibodies for IL-1β and NGF (1:1000 dilution), and washed three times with TBST. Afterwards, the membrane was incubated with horseradish peroxidase-labeled goat anti-rat secondary antibody at room temperature for 2 h, washed three times with TBST, and developed with ECL reagent. Finally, gray values of the protein bands were measured with ImageJ software.
Statistical analysis
All data were analyzed by SPSS 19.0. The BBB scores were compared by repeated measures of ANOVA. The differences between IL-1β and the expression of NGF mRNA and protein were compared at different time points by one-way ANOVA. Inter-group comparisons were performed by the SNK-q test. A value of P < 0.05 was considered statistically significant.
Results
Motor function recovery of left hind limbs
No rats died in this study. The sham surgery group showed slightly sluggish postoperative response, with the motor function recovered to normal after they awoke from anesthesia. The left hind limbs of the model and WJC transplantation groups suddenly twitched upon semi-transection, suggesting that the model had been successfully established. All groups suffered from flaccid paralysis in the left lower limbs after they awoke from anesthesia. Although they began to eat more food and became more vigorous 3 d after surgery, the left lower limbs could only be dragged, and muscle tension in the back increased despite well-recovered skin. The WJC transplantation group managed to bend three joints of the left hind limbs and to bear weight while standing on postoperative day 14, and walked using the left hind limbs on postoperative day 28. The BBB scores at different time points are summarized in .
Table I. BBB scores at different time points.
Local ultrastructure of the injury site
On postoperative day 28, transmission electron microscopy (TEM) showed that the sham surgery group had integral spinal cord structure, Schwann cells, a small amount of unmyelinated nerve fibers, numerous medullated nerve fibers, and clear synapse structure (). In the model group, however, nerve fibers were scattered, the connective tissues and synapse had become vague or had even disappeared, and Schwann cells had shrunk (). There were no neuron-like cells around the injury site in the WJC transplantation group, and Schwann cells were less prone to proliferation in the injury center. Meanwhile, the myelin sheath had thickened, the myelin lamellae had become regular and dense without vacuolar degeneration, and there were large amounts of neurofilaments and microtubules in the axoplasm ().
IL-1β mRNA and protein expression in spinal cord tissues
Real-time fluorescent quantitative PCR showed that compared with the sham surgery group, the model and WJC transplantation groups began to express more IL-1β mRNA 3 h after surgery, and the levels peaked 3 h later and then recovered to normal 168 h after SCI. The IL-1β mRNA expression level of the WJC transplantation group was lower than that of the model group 6 h after SCI, which became most obvious 6 h and 12 h after surgery. Western blot exhibited that compared with the sham surgery group, the model and WJC transplantation groups began to express more IL-1β protein 3 h after surgery, and the levels peaked at 72 h and 168 h respectively. However, the expression level of the IL-1β protein in the WJC transplantation group was always lower than that in the model group at each time point tested (, , ).
Figure 2. IL-1β protein expressions in spinal cord tissues (1: sham surgery group; 2: model group; 3: WJC transplantation group).
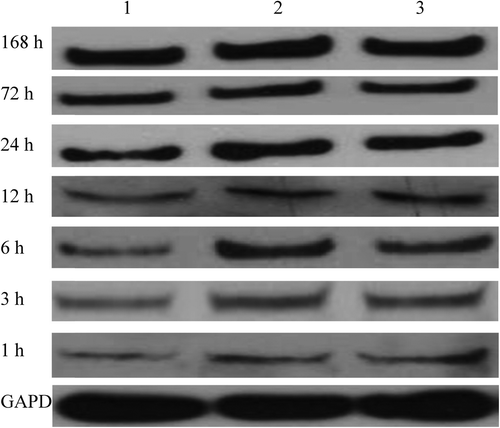
Table II. IL-1β mRNA expression in spinal cord tissues at different time points.
Table III. IL-1β protein expression in spinal cord tissues at different time points.
NGF mRNA and protein expression in spinal cord tissues
As suggested by real-time fluorescent quantitative PCR and Western blot, the levels of expression of NGF mRNA and protein in the WJC transplantation group were higher than those in the sham surgery and model groups (, ).
Figure 3. NGF protein expressions in spinal cord tissues 28 d after SCI (1: sham surgery group; 2: model group; 3: WJC transplantation group).
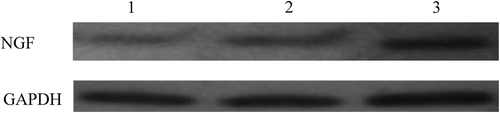
Table IV. NGF mRNA and protein expression in spinal cord tissues 28 d after SCI.
Discussion
Recently, SCI has been well treated by using the self-renewal and multi-directional differentiation of stem cells (CitationLi and Lepski 2013). WJCs aggregate toward the injury site in the host, survive, and recover the neurological function of SCI rats (CitationDai et al. 2013). However, the mechanism followed is unknown. WJC transplantation treats SCI probably by replacing necrotic tissue cells and injured neurons (CitationDai et al. 2013), and by inhibiting local inflammatory response (CitationBethea and Dietrich 2002). The effects of WJCs on inflammatory factors are manifested as: a disturbance of the balance between chemokines and their receptors, thus alleviating secondary SCI; the regulation of inflammatory factor expression during early SCI (upregulation of anti-inflammatory factors and downregulation of pro-inflammatory factors) and interaction with corresponding effector cells; and the protection of neurons by regulating the inflammatory cascade.
In this study, the BBB scale and TEM showed that WJC transplantation obviously recovered the motor function, and considerably increased neurofilaments and microtubules at the injury site, which partially fused with spinal cord tissues. Hence, WJC transplantation managed to recover the injured tissues.
SCI is a severe trauma of the central nervous system, and acute SCI is immediately followed by secondary inflammatory response and pathological changes at the site of injury and the adjacent spinal cord tissues. In the meantime, nerve function is deprived owing to the disorder in microcirculation, bleeding, edema, necrosis, and liquefaction (CitationTator and Fehlings 1991). On the other hand, IL-1β accumulates leukocytes and stimulates both the leukocytes and endothelial cells to express intercellular adhesion molecules, mediates infiltration of neutrophils, aggravates local inflammatory response, induces release of inflammatory cytokines such as TNF-α and IL-6, and enhances the synthesis and release of NO and EAA. CitationNesic et al. (2001) reported that IL-1β mRNA expression was significantly upregulated in the injury site after SCI. CitationGourin and Shackford (1997) found that overexpression of IL-1β after SCI was involved in the onset of secondary SCI. In this study, the WJC transplantation group, which expressed more IL-1β mRNA and protein than the sham surgery group did after SCI, had lower levels of expression than those in the model group, indicating that WJCs mitigated inflammatory response by reducing IL-1β expression in the spinal cord.
First found and studied most thoroughly, NGF, as a neurotrophic factor, promotes the differentiation and maturation of sympathetic and sensory nerve cells, maintains normal neuronal function, and specifically facilitates the growth of neurites into nerve fibers. Moreover, NGF can effectively prolong target cell injury-induced sudden death of neurons, maintain the survival of neurons after SCI, contribute to the reconstruction of neural circuits by cooperating with the cell adhesion molecules, cell-mediated elongation factor, and mechanical environment, and finally, rebuild physiological functions (CitationVaron and Conner 1994). CitationTuszynski et al. (1997) reported that after SCI, NGF induced the extension of sensory neuronal and noradrenergic neuronal fibers in adult rats, as well as local sprouting of motor neurons. By locally injecting NGF into rats with transecting SCI, CitationFernandez et al. (1993) found that the density of the axons in the corticospinal tract in the experimental group significantly exceeded that of the control group. SCI significantly increased NGF expression. In this study, the model group and the WJC transplantation group expressed more NGF than the sham surgery group did 28 d after SCI, and the level of was highest in the transplantation group. Accordingly, WJC transplantation enhanced NGF expression in SCI tissues, thereby relieving the injury to neurons and benefiting the repair and regeneration of nerve injury.
In summary, WJC transplantation improved the microenvironment at the site of SCI, inhibited IL-1β expression in spinal cord tissues, increased NGF expression, and nutritionally supported the recovery of rat neurological function. It is probable that WJCs exerted these effects by regulating inflammatory response and by decreasing secondary SCI. The findings provide valuable evidence for unveiling the mechanism by which stem cell transplantation treats SCI.
Declaration of interest
The authors report no declarations of interest. The authors alone are responsible for the content and writing of the paper.
References
- Basso DM, Beattie MS, Bresnahan JC. 1995. A sensitive and reliable locomotor rating scale for open field testing in rats. J Neurotrauma. 12:1–21.
- Bethea JR, Dietrich WD. 2002. Targeting the host inflammatory response in traumatic spinal cord injury. Curr Opin Neurol. 15: 355–360.
- Dai G, Liu X, Zhang Z, Yang Z, Dai Y, Xu R. 2013. Transplantation of autologous bone marrow mesenchymal stem cells in the treatment of complete and chronic cervical spinal cord injury. Brain Res. 1533:73–79.
- Fernandez E, Pallini R, Lauretti L, Mercanti D, Serra A, Calissano P. 1993. Spinal cord transection in adult rats: effects of local infusion of nerve growth factor on the corticospinal tract axons. Neurosurgery. 33:889–893.
- Gourin CG, Shackford SR. 1997. Production of tumor necrosis factor-alpha and interleukin-1beta by human cerebral microvascular endothelium after percussive trauma. J Trauma. 42:1101–1107.
- Li J, Lepski G. 2013. Cell transplantation for spinal cord injury: a systematic review. Biomed Res Int. 2013:786475.
- Nesic O, Xu GY, McAdoo D, High KW, Hulsebosch C, Perez-Pol R. 2001. IL-1 receptor antagonist prevents apoptosis and caspase-3 activation after spinal cord injury. J Neurotrauma. 18:947–956.
- Oliveri RS, Bello S, Biering-Sørensen F. 2014. Mesenchymal stem cells improve locomotor recovery in traumatic spinal cord injury: systematic review with meta-analyses of rat models. Neurobiol Dis. 62:338–353.
- Oyinbo CA. 2011. Secondary injury mechanisms in traumatic spinal cord injury: a nugget of this multiply cascade. Acta Neurobiol Exp (Wars). 71:281–299.
- Ryu HH, Kang BJ, Park SS, Kim Y, Sung GJ, Woo HM, et al. 2012. Comparison of mesenchymal stem cells derived from fat, bone marrow, Wharton's jelly, and umbilical cord blood for treating spinal cord injuries in dogs. J Vet Med Sci. 74:1617–1630.
- Tator CH, Fehlings MG. 1991. Review of the secondary injury theory of acute spinal cord trauma with emphasis on vascular mechanisms. J Neurosurg. 75:15–26.
- Tuszynski MH, Murai K, Blesch A, Grill R, Miller I. 1997. Functional characterization of NGF-secreting cell grafts to the acutely injured spinal cord. Cell Transplant. 6:361–368.
- Varon S, Conner JM. 1994. Nerve growth factor in CNS repair. J Neurotrauma. 11:473–486.
- Wang CX, Olschowka JA, Wrathall JR. 1997. Increase of interleukin- 1beta mRNA and protein in the spinal cord following experimental traumatic injury in the rat. Brain Res. 759:190–196.