Abstract
We prepared and evaluated a multifunctional envelope-type nano device (MEND) as a liver-targeting and long-circulation carrier for SiRNA. The polymer GA-PEG-Pp-DOPE was synthesized by modifying polyethylene glycol (PEG) with glycyrrhetinic acid (GA), peptide (Pp), and dioleoyl phosphoethanolamine (DOPE). The Pp is a substrate of matrix metalloproteinase 2. MEND was prepared with GA-PEG-Pp-DOPE and cationic phospholipids by the filming-rehydration method, and the orthogonal test was applied to optimize the prescription. The results of the biological evaluation results suggest that MEND is a promising delivery system for SiRNA.
Introduction
RNA interference (RNAi), a feasible strategy of gene therapy for tumor treatment, is the technique of delivering small interfering RNA (SiRNA) into target tumor cells by transfection to depress or even block the expression of specific genes. RNAi is implemented through the action of the RNA-induced silencing complex (RISC) (CitationGreory et al. 2005). RISC combines with mRNA in cytoplasm and hinders reverse transcription.
One of the key challenges for successful transfection of SiRNA into target cells is the development of safe and efficient vectors, because naked SiRNA is easily subjected to degradation and is hardly concentrated in target tissues. Vectors for SiRNA are subdivided into viral and non-viral vectors. Viral vectors have high transfection efficiency. However, their clinical application is restrained by concerns of high immunogenicity, carcinogenicity, and other risks of mutation. A multitude of non-viral vectors have been developed, to overcome the drawbacks of their viral counterparts. Generally speaking, non-viral vectors have low immunogenicity and low carcinogenicity, and their large-scale production is relatively easy (CitationDong et al. 2012). One of the promising non-viral vectors, multifunctional envelope-type nano device (MEND), is designed based on the study of programmed packaging (CitationKogure et al. 2004, CitationHatakeyama et al. 2007). The MEND is advantageous to implement the notion of programmed packaging, and it is a modified liposome which can transfer SiRNA to the correct place at the appropriate time. The MEND can enable the SiRNA to achieve a high expression in vitro, owing to the control of intracellular trafficking (CitationHatakeyama et al. 2007).
For successful development of a systematic gene delivery system, organ-targeting property and in vivo stability are principal considerations. According to Harashima's method(CitationHatakeyama et al. 2007), we designed and prepared liver-targeting and long-circulation MEND with GA-PEG-Pp-DOPE, a polymer synthesized earlier. Binding to the glycyrrhetinic acid (GA) receptors of hepatic parenchymal cells, GA engenders an active liver-targeting efficiency (CitationTian et al. 2009). Polyethylene glycol (PEG) extends the blood circulation time of MEND by improving the hydrophilicity of MEND particles. However, the existence of PEG could obstruct the endocytosis of MEND by causing steric hindrance. Recognized by matrix metalloproteinase 2 (MMP-2) which is overexpressed on the tumor cell surface, the Pp will be degraded and the PEG will be cut from the MEND, facilitating the endocytosis of the MEND particles. Therefore, the peptide (Pp) produces additional liver-targeting ability. The enhanced permeability and retention (EPR) effect of tumor cells needs a diameter of less than 200 nm, and we ensured that our MEND particles averaged 165 nm in size.
Materials and instruments
GA (98%), Zelang Medical Technology Co., Ltd.; NH2-PEG-COOH 2000 (90%), Seebio Biotech, Inc.; Pp (98%), Sangon Biotech (Shanghai) Co., Ltd.; Dioleoyl Phosphoethanolamine (DOPE) and DOTAP Chloride (DOTAP), Shanghai Advanced Vehicle Technology Pharmaceutical, Ltd.; Cy3-SiRNA, Guangzhou RiboBio Co., Ltd.; Poly-L-lysine (PLL, mw 30,000–70,000), Sigma-Aldrich Co. LLC.; NC-SiRNA (with sequence of ) and K-Ras-SiRNA (with sequence of
), Shanghai GenePharma Co., Ltd.; N,N’-Dicyclohexylcarbodiimide (DCC, 90%) and N-Hydroxysuccinimide (NHS, 90%), J&K Scientific Ltd.; Dimethyl sulfoxide and Dichloromethane, China Sinopharm International (Shanghai) Co., Ltd.; BEL-7402 cell line, Boster Biological Technology Co., Ltd; Fetal Bovine Serum, Zhejiang Tianhang Biological Technology Co., Ltd.; DMEM/High Glucose (1×) and 0.25% trypsin solution, Thermo Fisher Scientific Inc.; Nuclear Magnetic Resonance (NMR) Spectrometer (JNM- ECP600), JEOL; Zetasizer Nano instrument (Zetasizer Nano-ZS90), Malvern Instruments Ltd.; Transmission electron microscope (JEM2010), JEOL. Other chemicals were all of analytical or chromatographic grade.
Methods and results
Synthesis of GA-PEG-Pp-DOPE
Synthesis of GA-PEG
GA and PEG were connected via the amidation reaction (). GA (235.5 mg, 0.5 mmol) and DCC (206.0 mg, 1.0 mmol) were dissolved in 10 ml of THF, and NHS (115.0 mg, 1.0 mmol) was added into the solution. The solution was stirred for 18 h at room temperature, the resulting mixture was filtrated, and the filtrate was washed with diethyl ether to obtain GA-NHS (227.2 mg, 80%). NH2-PEG-COOH (200 mg, 0.1 mmol) and GA-NHS (85.2 mg, 0.15 mmol) were dissolved in 10 ml of DCM, and stirred at 35°C for 54 h; the resulting mixture was filtrated, and the filtrate was washed with diethyl ether to obtain GA-PEG-COOH (186.1 mg, 76%). The 1H NMR spectra of GA, NH2-PEG-COOH and GA-PEG-COOH were obtained using a NMR spectrometer at 600 MHz. The 1H NMR of GA-PEG-COOH showed 7 new methyl ions attributed to GA and a new sharp signal at d = 3.64 ppm, which is attributed to the –CH2– of NH2-PEG-COOH, suggesting that GA has already coupled to NH2-PEG-COOH ().
Synthesis of GA-PEG-Pp
The Pp with a sequence of PLGLAG (CitationJiang et al. 2004, CitationLevi et al. 2010) was connected to GA-PEG-COOH via the amidation reaction (). GA-PEG-COOH (186.1 mg, 0.08 mmol) and DCC (32.9 mg, 0.16 mmol) were dissolved in 20 ml of DMSO, followed by the addition of NHS (18.4 mg, 0.16 mmol), and stirred for 30 min. Pp (42.1 mg, 0.08 mmol) was then added into the mixture and agitated for more than 4 h at room temperature, the reaction mixture was filtered, and the filtrate was placed into a dialysis bag with a MWCO of 1000, and dialyzed against ultrapure water. The water was refreshed at 1, 3, 12, and 24 h after the start of dialysis; the aqueous solution in the dialysis bag was then lyophilized to obtain the solid GA-PEG-Pp floccule (134.5 mg, 57%). MS assay indicated conspicuous peaks near 3000.
Synthesis of GA-PEG-Pp-DOPE
GA-PEG-Pp and DOPE were combined through the amidation reaction (). GA-PEG-Pp (118.4 mg, 0.04 mmol), DCC (16.5 mg, 0.08 mmol), and NHS (9.2 mg, 0.08 mmol) were added into 10 ml of chloroform, and stirred at room temperature for 1 h. DOPE (29.8 mg, 0.04 mmol) was dissolved in 1 ml of chloroform added into the solution in the previous step, and stirred for 18 h. The resulting mixture was filtered and the filtrate was dried in vacuum. After purification by size exclusion chromatography and lyophilization, GA-PEG-Pp-DOPE (38.9 mg, 26.4%) was obtained. MS assay showed numerous peaks around 3800, which represented the molecular weight of GA-PEG-Pp-DOPE.
Preparation of SiRNA-MEND
SiRNA condensing
Poly-L-lysine (PLL) was chosen to condense SiRNA to enable a more stable structure. PLL-SiRNA complexes were obtained by adding PLL into SiRNA dissolved in RNase-free water. To find the optimal mass ratio of PLL to SiRNA, agarose gel electrophoresis (AGE) of different mass ratios of PLL/SiRNA complexes was employed to evaluate the SiRNA condensing capacity. Each well was loaded with 10 μl of PLL/SiRNA complex solution. All instruments and solvents were RNase-free. depicts the migration of SiRNA complexed with PLL within the agarose gel. According to , the SiRNA condensing capacity of PLL rises along with the increase of the mass ratio of PLL to SiRNA. We chose 20:1 as the optimum mass ratio.
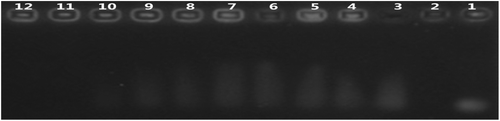
Preparation of the MEND
We prepared the liposome membrane with the filming-rehydration method. GA-PEG-Pp-DOPE (2 mg) and DOTAP (9 mg) were completely dissolved in chloroform, and the PLL/SiRNA complex was diluted with 5 ml of PBS and added into a 150 ml round-bottom flask to hydrate the liposomes. The resulting mixture was treated with a probe-type ultrasonicator, and a translucent natty blue SiRNA-MEND solution was obtained.
Physicochemical properties of SiRNA-MEND
Characterization of SiRNA-MEND
SiRNA-MEND (10 μL) was diluted with 2 ml of deionized water and dropped on a 300 mesh copper grid, and the excess fluid was removed with absorbent paper. Negative staining was performed using 5% aqueous phosphotungstic acid for 5 min before observation with a transmission electron microscope (TEM). In , A is the TEM photograph of globules with lipid bilayers, B is the TEM photograph of the fingerprint structure ().
Particle size and zeta potential
The particle size and zeta potential of SiRNA-MEND were determinated with a Zetasizer Nano instrument. The particle size ranged from 163.5 ± 20 nm ( is 0.124). The zeta potential of the SiRNA-MEND was near neutral ().
Encapsulation efficiency of SiRNA
Six samples of SiRNA-MEND, with different amounts of SiRNA and the same amount of MEND were prepared respectively. By adjusting the pH of the solution to be PI of PLL, SiRNA would be separated from the non-encapsulated PLL/SiRNA complex. Four hundred μl of each sample was treated with ultrafiltration centrifugation for 10 min in centrifuge tubes with a MWCO of 30,000. The concentration of SiRNA in the supernatant was determined with a nanophotometer. As is shown in , the encapsulation efficiency (EE) of SiRNA was calculated using the following formula: EE = (N ˗ CV)/N × 100%, where N is the content of SiRNA packaged within liposome, V is the volume of the supernatant remaining after centrifugation, and C is the concentration of SiRNA in the supernatant.
Table I. EE of SiRNA in the MEND.
When the SiRNA concentration was 18 μg/ml, the highest EE of 82.8% was obtained and the zeta potential was nearly neutral. We chose 18 μg/ml as the optimal prescription because it could improve the utilization rate of SiRNA, even though it was not the highest loading content. The SiRNA-MEND was used in the following experiments.
SiRNA-MEND cleavage responds to MMP-2
Three groups of different samples were prepared. Group 1 was the sample with GA-PEG-MEND mixed with 1 ml of 56 nM bovine serum albumin (BSA) dissolved in PBS, Group 2 was the sample with GA-PEG-Pp-MEND dissolved in PBS, and Group 3 was the sample with GA-PEG-Pp-MEND with 1 ml 56 nM BSA dissolved in PBS. To each of the three groups, 1 ml of 50 nM MMP-2 solution was added and incubated at 37°C, respectively. For each group, 1 ml of sample was taken to detect particle size with a Zetasizer Nano instrument, at 3 h, 12 h, and 24 h after the start of incubation.
DOPE forms an inverted hexagonal phase and readily aggregates under physiological conditions (CitationHolland et al. 1996). When DOPE was modified by PEG, the hexagonal phase becomes a bilayer phase and the size of the lipid particles shrinks (to a diameter less than 200 nm) to form a stable liposomal structure. When the Pp is degraded by MMP-2, GA-PEG is cleaved, PEG is cut off from the liposome, and the particle size mushrooms, leading to the drastic expansion of particle size. Within 24 h, while there was no significant discrepancy between Group 1 and Group 2, the particle size of Group 3 had rocketed ().
Degradation of SiRNA-MEND by serum
The degradation of SiRNA-MEND was estimated by AGE. Naked SiRNA, liposomes of DOTAP encapsulating SiRNA, and SiRNA-MEND were mixed with equal volumes of FBS in 3 Eppendorf tubes, respectively. All tubes were incubated at 37°C. The integrity of SiRNA at different time intervals was examined by AGE, after breaking the membrane with 1% Triton X-100, in every well that was loaded with 10 μl of sample.
According to , the lifespan of naked SiRNA in serum was 2 h, that of DOTAP/SiRNA was 15 h, and that of SiRNA-MEND was up to 120 h, which indicated that the MEND may extend the circulation of SiRNA in serum.
Activity of SiRNA-MEND in vitro
Determination of cell survival rate
The cytotoxicity of the MEND was assessed using the standard MTT assay. BEL-7402 cells were seeded in 96-well plates at a density of 4 × 104 cells per well. After 24 h of incubation, 0, 0.5, 1, 1.5, 2, 2.5, 3, 3.5, 4, and 4.5 μl of MEND solutions and DMEM medium were added into designated wells, respectively. The cells were incubated for another 24 h, 48 h, and 72 h, after which 20 μl of MTT was added into each well and incubated at 37°C for 4 h; the cell medium containing MTT was then replaced with 150 μl of DMSO. The microplate was incubated at 37°C for 5 min and shaken for 10 min at room temperature, to complete the dissolution of formazan. The absorbance of each well at 490 nm was determined through ELISA. The cell survival rate was calculated as the percentage of the absorbance of treated cells to that of untreated cells. The mean cell survival rate at all concentrations of MEND was more than 87%. There were no significant differences (p > 0.05) between the 9 groups during the 3 time-points. The results demonstrated that the MEND has an excellent biocompatibility profile and great promise for safely delivering SiRNA.
Transfection efficiency test
BEL-7402 cells were used to examine the transfection efficiency of the MEND. BEL-7402 cells were seeded in a Petri dish of 35 mm at a density of 5 × 105 cell/ml and incubated for 24 h. Cy3-SiRNA, Cy3-SiRNA-Liposomes, and Cy3-SiRNA-MEND were added into the petri dish at a final SiRNA concentration of 100 nM respectively. After incubation for 6 h, transfection efficiency of Cy3-SiRNA was examined according to fluorescence emitted by the fluorescent label Cy3, using a fluorescence microscope.
The fluorescence intensity and transfection efficiency were the highest in the Cy3-siRNA-MEND group. It was found to be almost 4-fold higher than the values for the control group, when studied with a fluorescence microscope (). The results indicated that the MEND could transfer SiRNA into cells with a high efficiency.
Endocytosis of the MEND
To confirm the cellular endocytosis of SiRNA-MEND, double staining was performed. The cells were treated with Cy3-SiRNA-MEND, which emits red fluorescence, for 6 h, then the cell nucleus was stained with DAPI (4’,6-diamidino-2-phenylindole), which emits blue fluorescence. The cells were observed under a fluorescence microscope, and the results suggested that the Cy3-SiRNA-MEND could be endocytosed into the cytoplasm ().
Cell invasion assay
To evaluate the inhibition of SiRNA on migration of the BEL-7402 cells in vitro, the Boyden chamber cell invasion assay was performed. The lower compartment was filled with 600 μl of 10% FBS. The upper surface of the transwell was previously coated with extracellular matrix (ECM). A sample of 200 μl of MEND transfected with Ras (CitationNakano et al. 2001) for 48 h in suspension cells, and 200 μl of non-transfected suspension cells were seeded in a 6-well plate with a transwell filter membrane, respectively. The cells in the upper compartment were observed at 5-h intervals. Crystal violet was used to dye the invasive cells, which migrated across the transwell to the lower counterpart. The cells were counted with a cell counter chamber under a microscope.
The average number of cells of the non-transfected cell group and the transfected cell group were 373 and 132 respectively (), indicating that the cell migration was effectively inhibited by the transfection of SiRNA-MEND.
Gene silencing test
The real-time fluorescent quantitative RT-PCR method was performed to detect gene expression. We used Ras as the target gene, and GAPDH as the internal control gene. BEL-7402 cells were seeded in 6-well plates at a density of 6 × 105 cells per well, and incubated for 24 h. Ras-SiRNA-MEND was added to ensure that the final concentration was 100 nM, MEND without SiRNA was added as the control group, and the total RNA was isolated with trozol reagent from the cells in each well.
, A and B, represent normalized melting peaks of the Ras gene in BEL-7402 cells treated with Ras-SiRNA-MEND and MEND without SiRNA respectively. C and D, appearing to overlap, represent normalized melting peaks of the GAPDH gene in BEL-7402 cells treated with Ras-SiRNA-MEND and MEND without SiRNA respectively. The results showed that with the transfection by Ras-SiRNA-MEND, the gene expression in cells is lower than when treated with MEND without SiRNA, by 78.61-fold ().
Statistical analysis
Values are presented as the mean ± standard deviation. One-way analysis of variance (ANOVA) was used to determine the significance among groups. A value of p < 0.05 was considered to be significant.
Discussions
This study investigated the synthesis of a novel polymer GA-PEG-Pp-DOPE, the preparation of SiRNA-MEND particles modified with the polymer, and the estimation of the SiRNA-MEND particles. RNAi is a promising and potential gene therapy for the treatment of cancer. However, its clinical application is limited by the deficiencies of naked SiRNA: rapid enzymatic degradation, poor cell membrane penetration, and no tumor-targeting property. To achieve efficient transfection of SiRNA to tumor cells, vectors are necessary for the effective protection and systematic delivery of SiRNA.
Using PEG, which ameliorates the hydrophilicity of nanoparticles, we extended the in vivo circulation time of PEG-modified MEND particles by 4-fold, to up to 120 h. However, with improved hydrophilicity, it might prove difficult for PEG-modified particles to be engulfed by cells. To overcome the difficulty, we attached to PEG a specific Pp with an amino acid sequence of PLGLAG. By cleavage of MMP-2 within the 6 amino acid segment, the PEG was cut off from the particle, exposing the hydrophobic surface of the MEND particle. It is more likely that cells will endocytose hydrophobic particles. The results showed that the transfection rate was as high as 90%. GA, the main constituent in Glycyrrhiza uralensis Fisch, has high affinity for specific receptors on the membrane of parenchymal hepatic cells (CitationHolland et al. 1996), and engenders active and efficient liver-targeting property, using Ras-SiRNA, a specific SiRNA which inhibits the transcription of liver cancer gene. There is a high expression of Ras protein in the tumor. The interference of reverse transcription of Ras can be achieved by transfer of Ras-SiRNA (CitationNakano et al. 2001), and the expression of Ras can be inhibited. We evaluated the liver-targeting property and transfection efficiency of our MEND particles. These particles performed well in both assays.
For the successful preparation of the MEND, all ingredients were connected via amidation using DCC and NHS as catalysts (CitationHuang et al. 2011). Extraction and purification of the products is required after every single reaction. The results show that there is no statistically significant difference among groups. The average diameter is 163.5 ± 20 nm, which meets the requirement of EPR effect for particle size. Our MEND particles showed good SiRNA condensing ability. The EE is up to 82.8%. The in vitro study showed that the MEND particles had low toxicity and high transfection efficiency.
Declaration of interest
The authors report no conflicts of interest. The authors alone are responsible for the content and writing of the paper.
References
- Dong W, Zhou Y, Liang W. 2012. Advances in nano lipid carrier of SiRNA delivery. Prog Biochem Biophys. 39:396–401.
- Greory RI, Chendrimada TP, Cooch N, Shiekhattar R. 2005. Human RISC couple microRNA biogenesis and posttranscriptional gene silencing. Cell. 123:631–640.
- Hatakeyama H, Akita H, Kogure K, Oishi M, Nagasaki Y, Kihira Y, et al. 2007. Development of a novel systemic gene delivery system for cancer therapy with a tumor-specific cleavable PEG-lipid. Gene Ther. 14:68–77.
- Holland JW, Cullis PR, Madden TD. 1996. Poly(ethylene glycol)-lipid conjugates promote bilayer formation in mixtures of nonbilayer-forming lipids. Biochemistry. 35:2610–2617.
- Huang W, Wang W, Wang P, Zhang CN, Tian Q, Zhang Y, et al. 2011. Glycyrrhetinic acid-functionalized degradable micelles as liver-targeted drug carrier. J Mater Sci Mater Med. 22:853–863.
- Jiang T, Olson ES, Nguyen QT, Roy M, Jennings PA, Tsien RY. 2004Tumor imaging by means of proteolytic activation of cell-penetrating peptides. Proc Natl Acad Sci USA. 101:17867–17872.
- Kogure K, Moriguchi R, Sasaki K, Ueno M, Futaki S, Harashima H. 2004. Development of a non-viral multifunctional envelope-type nano device by a novel lipid film hydration method. J Control Release. 98:317–323.
- Levi J, Kothapalli SR, Ma T-J, Hartman K, Khuri-Yakub BT, Gambhir SS. 2010. Design, synthesis, and imaging of an activatable photoacoustic probe. J Am Chem Soc. 132:11264–11269.
- Nakano M, Aoki K, Matsumoto N, Ohnami S, Hatanaka K, Hibi T, Terada M, Yoshida T. 2001. Suppression of colorectal cancer growth using an adenovirus vector expressing an antisense K-ras RNA. Mol Ther. 3:491–499.
- Tian Q, Wang W, He XT, Zhu XC, Huang W, Zhang CN, Yuan Z, Chen XS. 2009. Glycyrrhetinic acid-modified nanoparticles for drug delivery: preparation and characterization. Chinese Sci Bull. 54:3121–3126.