Abstract
Objective: To study the cellular compatibility of the magnetic nanocomposite (n-HA/PLLA/Fe2O3) and to discuss the biological behaviors of cells including surface adhesion, proliferation, and expression. The present work provides an experimental basis for medical application. Method: Rat osteoblasts (OB) were co-cultured with the magnetic nanocomposite. Cell proliferation, cell adhesion, and the expression of type I collagen (Col-I) and osteocalcin (OCN) gene were characterized by the cell counting kit-8 (CCK-8) method, scanning electronic microscopy (SEM), and reverse transcription polymerase chain reaction (RT-PCR), respectively. Results: CCK-8 detection showed that there was no difference in cell proliferation on the magnetic nanocomposite between the experimental group and control group (P > 0.05). SEM indicated that a large amount of cells adhered to the surface and in the pores of the magnetic nanocomposite. As the co-culture time increased, the cells adhering to the magnetic nanocomposite showed an obvious increase. RT-PCR detection showed that as the co-culture time increased, the expression of the Col-I gene was enhanced (P 0.05). There was no obvious difference in the expression of the OCN gene (P > 0.05). Conclusion: The magnetic nanocomposite is suitable for cell adhesion, growth, and differentiation with a high cellular compatibility.
Biomaterials such as n-HA (nano-hydroxyapatite) and PLLA (poly(L-lactic acid)) have found extensive applications in orthopedics, with significant clinical efficacy. In recent years, with the progress of biological engineering and biomedicine, biocomposites have been researched intensively. The introduction of some functional materials is necessary to construct biocomposites with high performance. For instance, magnetic Fe2O3 particles, which are monodispersed, nano-sized, and functional, have a large specific surface area, high adsorption, and strong agglutination effect. Moreover, the surface of the magnetic particles is reactive, which confers the magnetic particles with a wide applicability (Chen et al. 2013, Mehdikhani-Nahrkhalaji et al. 2012, Zhu et al. 2008, Zhu et al. 2014a). In this research, the low-temperature rapid-modeling instrument was used to prepare a magnetic nanocomposite with n-HA, PLLA, and Fe2O3 (Zhu et al. 2014b). In the present work, rat osteoblasts (OB) were co-cultured with the magnetic nanocomposite to elucidate the biological behaviors of cells including surface adhesion, proliferation, and expression. The results provide an experimental basis for medical application.
Materials and methods
Experimental materials, reagents, and instruments
α-MEM, fetal bovine serum, 500 U/ml penicillin and 500 μg/ml streptomycin (Gibco, USA), CCK-8 cell proliferation and cytotoxicity assay kit (Keygen). TRIzol@Reagent (Invitrogen, USA). Magnetic nanocomposite (n-HA/PLLA/Fe2O3) (Shenzhen Institute of Advanced Technology, Chinese Academy of Sciences), cube (25mm × 25mm × 25mm) (see ). The composite was a 3D porous cubic structure with a porosity of 80%–85% (Mehdikhani-Nahrkhalaji et al. 2012). SEM (MIRA3 TESCAN, Research Institute of Tsinghua University in Shenzhen) showed evenly distributed and interconnected pores. Local amplification showed an uneven and rough surface (). The n-HA/PLLA/Fe2O3 composite was first irradiated by ultraviolet rays for half an hour, then rinsed with PBS, disinfected by 75% ethanol, and dried.
Isolation and culture of rat osteoblasts (OB)
Rat OBs were isolated by the enzyme digestion method. Three fetal rats within 24 h were killed by cervical dislocation and disinfected by 75% ethanol. The scalp was excised and the skull was harvested. The brain tissues and blood were removed in PBS. The surrounding connective tissues were scraped off to obtain the white skull, which was then cut into a mud-like sample with scissors. A quantity of 5 mL of trypsin was added for cell digestion at 37°C in a water bath for 30 min. The digestion solution was discarded, the cells were rinsed with PBS once, and 5 mL of type II collagenase was added. During digestion at 37°C in the water bath for 30 min, the tube was shaken mildly. The collagenase was collected and centrifuged at 1000r/min for 5 min. The supernatant was discarded and an appropriate amount of α-MEM medium was added. The cells were gently blown to prepare a cell suspension, which was transferred to a cell culture flask. Next, 5 mL of type II collagenase was added into the remaining bones and digestion was performed at 37°C in a water bath for 30 min. The above steps were repeated to prepare a cell suspension. The cell suspension was collected and inoculated to the cell culture flask. The cells were cultured in an incubator at 37°C, 5% CO2, and saturated humidity. The liquid was replaced on the second day, and after that, the frequency of replacement was changed to once every two days. The rat OBs adhered well to the wall, showing an oval shape, spindle shape, triangular shape, or the shape of an irregular polygon (). The cells adhered together via pseudopodia. The cytoplasm was clear, and the nuclei were round or oval and were located in the middle or on one side of the cells. There were 1–3 nuclei, and 1 or more nucleoli were found. The third-generation OB were used for the co-culture experiment with the n-HA/PLLA/Fe2O3 composite.
Co-culture of OBs and the n-HA/PLLA/Fe2O3 composite
The n-HA/PLLA/Fe2O3 composite was inoculated with OBs in α-MEM medium containing 10% fetal bovine serum under sterilized conditions. The experiment consisted of two groups: in the experimental group (Group A), OBs were co-cultured with the n-HA/PLLA/Fe2O3 composite; in the control group (Group B), OBs were cultured independently. The 24-well plate was used, and the cell inoculation density was 5 × 105 cells/well. The cells were cultured in an incubator at 37°C and 5% CO2. The liquid was replaced once every 3 days.
Detection indicators
Detection of cell proliferation activity
The CCK-8 method was adopted. Five replicate wells were set up in Group A and Group B, respectively. A quantity of 50 μL of CCK-8 solution was added into each well on days 1, 3, 5, 7, and 9 of incubation. After incubation in the incubator at 37°C for 4 h, the liquid in each well was transferred to a 96-well plate, with the amount of liquid in each well being equal. The absorbance (A value) was detected with a microplate reader at the wavelength of 490 nm. The cell proliferation curve was plotted.
SEM
The n-HA/PLLA/Fe2O3 composite was taken out at weeks 1, 2, and 3 of co-culture. The composite was rinsed with PBS twice and fixed in 2.5% glutaraldehyde for 2 h. The sample was dehydrated with gradient ethanol and dried at the critical point of CO2. The sample was sprayed with metal and observed under SEM for the status of cell adhesion.
RT-PCR detection
Saqomonyants et al.‘s method (Weir et al. 2004) was employed and 1 × 106 rat OBs were collected at weeks 1, 2, and 3 of co-culture in Group A. When the primary rat OBs (P0) grew to 90% confluence, total RNA was extracted as blank control. Total RNA extraction was performed for 1 × 106 cells using 1 ml of TRIzol (Invitrogen). The extracted RNA was quantified by absorbance and measured with a UV spectrophotometer (WPA UV1101), with A260/280 > 1.8. Two-step RT-PCR detection was performed. The method and conditions of first-strand cDNA synthesis conformed to the instruction on the assay kit (Fermentas). The 25 μL reaction system consisted of 2.5 μL of 10xTaq buffer, 3.0 μL of 25 mmol/L MgCl2, 2.0 μL of 10 mmol/L dNTP, 1.0 μL of Taq DNA polymerase (1 U/μL), 18.5 μL of ddH20, 1.0 μL of template cDNA, and 1.0 μL each of forward primer and reverse primer (20 μL). The PCR program was as follows: predenaturation at 95°C for 3 min, 1 cycle; annealing for 45 s (see the annealing temperature below), extension at 72°C for 1 min, 30 cycles, and extension at 72°C for 7 min. Type-I collagen (Col-I), upstream primer 5’-CACTGGCGATAGTGGTCCTG-3’, downstream primer 5’- CGGCCACCATCTTGAGACTT-3’, annealing temperature 53°C, amplified fragment 132 bp; OCN, upstream primer 5’-TGCCAGGTCACCAAATACCA-3’, downstream primer 5’-TGAGTACTGAGAGGCCCCAA-3’.
Annealing temperature 58°C, amplified fragment 112 bp; reference GAPDH, upstream primer 5’-TGAGACCTTCAACACCCC-3’, downstream primer 5’-GCCATCTCTTGCTCGAAGTC-3’, annealing temperature 56°C, amplified fragment 316 bp. Next, 5 μL of products amplified using the primers of Col-I, OCN and GAPDH were stained by GelRed and treated with 2% agarose gel electrophoresis, respectively. The gray scales of different bands were measured with the Quantity One software, and semi-quantitative analysis was performed.
Statistical analysis
The measurement data were expressed as x ± s. One-way ANOVA was carried out for each group of data using SPSS 13.0. For intergroup comparison, the SNK-q test was performed.
Results
CCK-8
The proliferation of OBs on the n-HA/PLLA/Fe2O3 composite can be seen in . There was no obvious difference in cell proliferation between the experimental group and the control group (P > 0.05).
SEM
SEM images of co-culture of OBs and the n-HA/PLLA/Fe2O3 composite are shown in . An observation of the morphology showed that the cells were well-spread on the surface of the n-HA/PLLA/Fe2O3 composite, in fusiform or scale-like shape. The pseudopodia were fully extended and interconnected into the sheet. As the time of co-culture increased, the cells increased and fused gradually.
RT-PCR detection of the expression of Col-I and OCN
After co-culture for 1, 2 and 3 weeks, the expression of Col-I increased, and the difference was of statistical significance (P < 0.05). However, no obvious difference was observed with respect to the expression of OCN (P > 0.05). At week 1, the expression of Col-I did not show significant difference from P0 (P > 0.05). At weeks 2 and 3, the expressions of Col-I were both higher than that of P0 (P < 0.05). At weeks 1, 2, and 3, the expression of OCN was not significantly different from that of P0. See .
Figure 6. At weeks 1, 2, and 3, the expression of Col-I was increased and the difference was of statistical significance (P < 0.05). The expression of OCN did not show obvious difference (P > 0.05). At week 1, the expression of Col-I was not significantly different from that of P0 (P > 0.05). At weeks 2 and 3, the expression of Col-I was always higher than that of P0 (P < 0.05). At weeks 1, 2, and 3, the expression of OCN was not significantly different from that of P0.
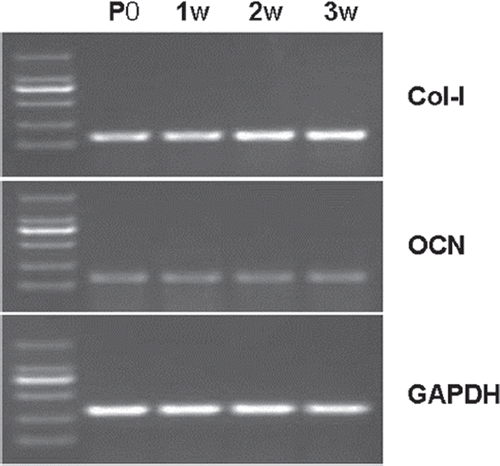
Discussion
The bionic composites for interface fixation can be degraded into products absorbable by the human body. During the early and middle stages of tendon–bone healing stages, these composites can provide good mechanical strength and properties to fix the transplanted tendon. With the degradation and absorption of the biomaterials, the load is transferred and the stress shielding effect is reduced. Due to the action of stress, the osteogenesis is enhanced and the healing of the local tendon–bone interface is accelerated. Thus, a series of implications are avoided. The n-HA/PLLA composites for interface fixation are currently at the research and development stage. Earlier experiments have confirmed that when the n-HA content reached 20%, the bending strength of the n-HA/PLLA composites was 175 Mpa, which satisfied the requirements of biomechanics for the fixation of the transplanted tendon. The nano-HA/PLLA material of this research is different from any other single material. It is composed of n-HA and PLLA via computer-aided rapid prototyping technology and freeze-drying compound. Thus, it has the characteristics of the two kinds of materials. (1) It is more advantageous for extracellular matrix protein adhesion and interaction due to the nanostructure of the material. It is able to guide tissue cells to grow, and the biodegradability increases as the result of the increase of surface area. (2) PLLA degradation products can be neutralized to some extent by n-HA, thus effectively preventing aseptic inflammation. It can also accelerate the degradation of the material. (3) Its composition, shape, microstructure, and mechanical properties can be predicted in advance and it can be made to meet the clinical needs in the process of material production.
Significance of the 3D interconnected structure
Although biomaterials have been widely applied in orthopedic surgery and relevant fields, the effective integration between the implant and bone tissues is still a great challenge. The existing studies on the biological stability and compatibility of biomaterials mostly concern the physiochemical properties or surface morphology. Experiments show that a rough surface is more favorable to capture the cellulose. An implant with a rough and porous surface makes it easier for the cells to adhere onto it. The accumulation of a large amount of cellulose can induce the aggregation of OBs and the ingrowth of bone tissues, thus accelerating osseointegration at early stage and increasing the strength of binding between bone and the implant (Zhu et al. 2012). The long-term stability of the implant can be realized by the binding between bone and the implant (Russias et al. 2006). Experimental results show that when the porous implant and the implant with thread are implanted, the porous implant has a larger contact area with the surrounding bone under load than the implant with thread. This means that the bone ingrowth can be accelerated (Weir et al. 2004). It is also shown that a 3D porous surface can promote OB proliferation and osteogenic capability (Ehrenfried et al. 2008).
Influence of aperture and porosity of porous structure on bone ingrowth
The aperture and porosity of the porous structure play an important role in bone ingrowth (Zhou et al. 2009). The diameter of a mature OB is about 20 μm. If the pore size of a material is smaller than this value, bone ingrowth will be impossible. The size of an osteon (bone matrix surrounding the nucleus of the osteocyte) is about 60–80 μm. To facilitate bone ingrowth into the porous structure, the aperture should be larger than 80 μm (Delabarde et al. 2012). As indicated by the research, a material with interconnected pores and apertures ranging from 430 to 650 μm provides a favorable condition for bone ingrowth (Russias et al. 2006). Porosity represents the number of pores in unit volume. A porosity favorable for bone ingrowth is 75%–85%. The n-HA/PLLA/Fe2O3 composite with interconnected pores had an aperture of about 500 μm and a porosity of 80%–85%, which meets the condition for bone ingrowth. However, the pores were occluded to a certain extent, which affected bone ingrowth (Varila et al. 2012).
Disagreement with experimental results in similar research studies
For a long time, the cellular compatibility of different scaffolds has been compared by taking the plastic culture plate as the “gold standard”. In the present work, the OB were co-cultured with n-HA/PLLA/Fe2O3 composite (Zhu et al. 2010). The cell proliferation on n-HA/PLLA/Fe2O3 composite did not show obvious differences from that on the plastic culture plate. RT-PCR detection indicated that Col-I expression was enhanced at week 3 of co-culture, but there was no obvious difference in OCN expression. Semi-quantitative PCR was employed, and a large variability of data was found. Therefore, it was very difficult to detect the significant differences in mRNA expression. Moreover, the mRNA of bone matrix proteins may be expressed to varying extents in different organisms of the same species (Kobayashi and Sakamoto 2009). The differences in experimental results can also be attributed to different physiochemical properties of the scaffold. To sum up, the n-HA/PLLA/Fe2O3 composite did not have an adverse impact on OB adhesion, growth, and differentiation. Thus, the n-HA/PLLA/Fe2O3 composite can serve as a scaffold with high cellular compatibility.
Conclusion
The aperture of the n-HA/PLLA/Fe2O3 composite prepared in this study was about 500 μm and the porosity was 80%–85%, which met the conditions of bone ingrowth. The n-HA/PLLA/Fe2O3 composite was co-cultured with rat OBs, and no obvious difference was observed in cell proliferation rate of the scaffold group (Group A) and the plastic culture plate (Group B) (P > 0.05). With the increase of co-culture time, more cells adhered to the scaffold. At weeks 1, 2, and 3 of co-culture, Col-I expression was increased, with statistical significance (P < 0.05). However, there was no obvious difference in OCN expression (P > 0.05). Our experiment showed that the n-HA/PLLA/Fe2O3 composite had no adverse impact on OB adhesion, growth, and differentiation. The composite can serve as a scaffold with good cell compatibility.
Acknowledgment
This study received financial support from Guangdong Science and Technology Project (the project number is 2013B021800100), and the Shenzhen Science and Technology Project (the project numbers are CXZZ20130321152713220 and JCYJ20140414170821164).
Declaration of interest
The authors report no declarations of interest. The authors alone are responsible for the content and writing of the paper.
References
- Chen S, Hao Y, Cui W, Chang J, Zhou Y. 2013. Biodegradable electrospun PLLA/chitosan membrane as guided tissue regeneration membrane for treating periodontitis. J Mater Sci. 48:6567–6577.
- Delabarde C, Plummer CJG, Bourban P-E, Manson EJA. 2012. Biodegradable polylactide/hydroxyapatite nanocomposite foamscafolds for bone tissue engineering applications. J Mater Sci Mater Med. 23:1371–1385.
- Ehrenfried LM, Patel MH, Cameron RE. 2008. The effect of tri-calcium phosphate (TCP) addition on the degradation of polylactide-co-glycolide (PLGA). J Mater Sci Mater Med. 19:459–466.
- Kobayashi S, Sakamoto K. 2009. Effect of hydrolysis on mechanical properties of tricalcium phosphate/poly-L-lactide composites. J Mater Sci Mater Med. 20:379–386.
- Mehdikhani-Nahrkhalaji M, Fathi MH, Mortazavi V, Mousavi SB, Hashemi-Beni B, Razavi SM. 2012. Novel nanocom-posite coating for dental implant applications in vitro and in vivo evaluation. J Mater Sci. 23:485–495.
- Russias J, Saiz E, Nalla RK, Tomsia AP. 2006. Microspheres as building blocks for hydroxyapatite/polylactide biodegradable composites. J Mater Sci Mater Med. 41:5127–5133.
- Varila L, Lehtonen T, Tuominen J, Hupa M, Hupa L. 2012. In vitro behaviour of three biocompatible glasses in composite implants. J Mater Sci Mater Med. 23:2425–2435.
- Weir NA, Buchanan FJ, Orr JF, Dickon GR. 2004. Degradation of poly-L-lactide. Part. 1:in vitro and in vivo physiological temperature degradation. Proc Inst Mech Eng [H]. 218:307–319.
- Zhou Z, Yi Q, Liu X, Liu L, Liu Q. 2009. In vitro degradation behaviors of poly-L-lactide/bioactive glass composite materials in phosphate-bufered solution. Polym Bull. 63:575–586.
- Zhu W, Chen K, Lu W, Sun Q, Peng L, Fen W, et al. 2014a. In vitro study of nano-HA/PLLA composite scaffold for rabbit BMSC differentiation under TGF-β1 induction. In Vitro Anim Cell Dev Biol. 50: 214–220.
- Zhu W, Huang J, Lu W,Sun Q, Peng L, Fen W, et al. 2014b. Performance test of Nano-HA/PLLA composites for interface fixation. Artif Cells Nanomed Biotechnol. 42:331–335.
- Zhu W, Lu W, Zhang X, Cai ZM, Liu HF, Peng LQ, et al. 2012. Nano-hydroxyapatite/fibrin glue/recombinant human osteogenic protein-1 artificial bone for repair of bone defect in an animal model. Micro Nano Lett. 7:467–471.
- Zhu W, Xiao J, Wang D, Liu J, Xiong J, Liu L, et al. 2008. Experimental study of nano-HA artificial bone with different pore sizes for repairing the radial defect. Int Orthop. 33:567–571.
- Zhu W, Zhang X, Wang D, Lu W, Ou Y, Han Y, et al. 2010. Experimental study on the conduction function of nano-hydroxyapatite artificial bone. Micro Nano Lett. 1:19–27.