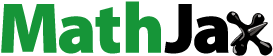
Abstract
The study reports a simple, inexpensive, and eco-friendly synthesis of copper oxide nanoparticles (CuONPs) using Piper betle leaf extract. Formation of CuONPs was confirmed by UV–visible spectroscopy at 280 nm. Transmission electron microscopy (TEM) images showed that the CuONPs were spherical, with an average size of 50–100 nm. The scanning electron microscopy (SEM)–energy dispersive spectroscopy (EDS) peak was observed approximately at 1 and 8 keV. The X-ray diffraction (XRD) studies indicated that the particles were crystalline in nature. CuONPs effectively inhibited the growth of phytopathogens Ralstonia solanacearum and Xanthomonas axonopodis. The cytotoxic effect of the synthesized CuONPs was analyzed using rat splenocytes. The cell viability was decreased to 94% at 300 μg/mL.
Introduction
The synthesis of metallic nanoparticles (NPs) has gained considerable attention in recent years due to their wide industrial applicability. Among the metallic NPs, copper oxide NPs (CuONPs) are known for their versatile applications in different fields, such as pharmaceutical, electronics, chemical sciences, solar cells, and biosensors (CitationSaterlie et al. 2011, CitationAzam et al. 2012, CitationDas et al. 2013, CitationKanhed et al. 2014). Several studies have reported the synthesis of CuONPs using physical and chemical methods (CitationZhang et al. 2001, CitationWang et al. 2002, CitationCarnes et al. 2002). Unfortunately, these methods typically make use of chemical agents that are toxic to biotic communities and pollute the environment (CitationLim and Hudson 2004, CitationZain et al. 2014). Hence, there is a need to develop alternative methods of CuONP synthesis that do not use toxic chemicals/reagents.
Recently, green synthesis of CuONPs has been widely accepted because it offers many advantages over the conventional physical and chemical methods. The green chemistry approach highlights the use of biological materials for the synthesis of CuONPs as reliable, simple and non-toxic (CitationNarayanan and Sakthivel 2010, CitationSathishkumar et al. 2012). Thus, considerable attention is given to exploit the synthesis of CuONPs using biological materials. Although there are a few reports on the use of microorganisms and plant extracts for synthesizing CuONPs (CitationHonary et al. 2012, CitationGunalan et al. 2012, CitationAbboud et al. 2014), there are none using Piper betle, and a search of existing literature indicated that this method of CuONP synthesis has been less explored.
Piper betle L. (Piperaceae) is an aromatic, shade-preferring climber cultivated mainly in India and other tropical Asian countries. The betle leaf is familiar as paan in India (CitationMazura et al. 2007, CitationSarkar et al. 2008). Research on Piper betle showed a vast number of pharmacological benefits, such as antioxidant, antimicrobial, and anti-inflammatory properties (CitationRathee et al. 2006, CitationAli et al. 2010, CitationPin et al. 2010). CitationUsha Rani and Rajasekharreddy, (2011) reported the synthesis of silver NPs using betle leaf extract. However, to our knowledge, Piper betle extract has never been used for the synthesis of CuONPs.
Plant disease control is important in agriculture. Microorganisms, especially bacteria, are associated with several crop diseases and significantly reduce the yield. Ralstonia solanacearum and Xanthomonas axonopodis are considered important bacterial pathogens and are recorded on several hundred crop varieties. Nowadays, it is difficult to control the diseases caused by these pathogens due to increased antibiotic resistance. With advances in nanotechnology, researchers have focused on the application of nanomaterials for controlling plant diseases. CuONPs are reported to have both antibacterial and antifungal activity (CitationDas et al. 2013, CitationSivaraj et al. 2014). CitationRen et al. (2009) reported that the CuONPs disrupt the cell membranes, causing cytoplasmic leakage, and eventually, the death of microorganisms.
The in vitro cytotoxicity study is an important assay to understand the toxicity caused by NPs. Most in vitro studies of NP toxicity are based upon the exposures of submerged cell cultures to particle suspensions. The Cu(II) in vitro cytotoxicity of the free Schiff base ligand and its complexes against two human carcinoma cell lines (HeLa and MCF-7) has been investigated (CitationAazam and El-Said 2014).
Thus, the objectives of this study were (i) green synthesis of CuONPs using Piper betle leaf extract, (ii) evaluation of the antibacterial activities of the synthesized CuONPs, (iii) evaluation of the RBC hemolysis in fresh blood, and (iv) evaluation of cytotoxicity of CuONP synthesized against freshly isolated rat splenocytes.
Materials and methods
Materials
Piper betle leaves were procured from a local market in Mallasamudram, Tamil Nadu, India. All the chemicals and solvents used in this study were of analytical grade and were obtained from Sigma-Aldrich (St. Louis, MO, USA).
Piper betle leaf extract
Fresh Piper betle leaves (50 g) were washed thoroughly using distilled water and allowed to dry in the shade. Later, the leaves were pulverized with methanol (95%) and filtered using Whatman No. 1 filter paper. The solvent present in the filtrate was evaporated under reduced pressure in a rotary vacuum evaporator at 50°C. The concentrated extract was transferred to Petri dishes and dried in atmospheric air. The dried extract was used for the synthesis of CuONPs.
Synthesis of CuONPs under optimized condition
Analytical grade copper sulfate (CuSO4) solution (5 mM) was prepared using ultrapure water (conductivity = 18 μΩ/m, TOC < 3 ppb) (Barnstead, Waltham, MA, USA), and the NP synthesis was carried out according to the method described by CitationSankar et al. (2014a). Briefly, 10 mL of Piper betle leaf extract was mixed with 90 mL of 5 mM CuSO4 solution, and the reaction mixture was incubated in a rotary shaker (180 rpm) at 26°C. Experiments were carried out at different levels of pH (4–9) and samples collected at predetermined time intervals (0–10 h) were monitored for copper reduction by measuring the absorbance at 200–800 nm using an UV–vis spectrophotometer (Shimadzu, Japan). The synthesis of CuONPs was carried out under optimized conditions. The reaction product was recovered by centrifugation at 12, 000 rpm for 15 min and purified by redispersion of the pellet in sterile water. The processes of centrifugation and redispersion were repeated three times and the CuONPs were freeze-dried at − 80°C for 24 h. The freeze-dried products were used for characterization and antibacterial studies. Control experiments were carried out without the Piper betle leaf extract.
Characterization of CuONPs
The transmission electron microscope (TEM, H-7650, Japan, HITACHI) was used to study the surface morphology and size of the CuONPs. The elemental analysis of the CuONPs was confirmed by scanning electron micrograph-energy dispersive spectroscopy (SEM–EDS; JEOL-64000, Japan). The X-ray diffractometry (XRD) study was carried out using the Rigaku X-ray diffractometer (XRD; Rigaku, Japan). Scanning was done in the region of 2θ from 4 to 80° at 0.041/min with a time constant of 2 s. The Fourier transform infrared (FTIR) spectra of the CuONPs were obtained (FTIR; Perkin-Elmer USA) in the diffuse reflectance mode at a resolution of 4 cm− 1 in KBr pellets. Absorbance data were obtained in the range of 500–4000 cm− 1.
Antibacterial activity of CuONPs
The phytopathogens—R. solanacearum and X. axonopodis pv. alfalfae—were procured from the Korean Agriculture Culture Collection (KACC), South Korea. The antibacterial activity was evaluated by the well-diffusion method according to (CitationBauer et al. 1966). In brief, Muller-Hinton agar was prepared and 200 μl of bacterial culture was swabbed using a sterile cotton swab. After 10 min, a well (5 mm) was made by gel puncture and 100 μl (25 μg/mL) of the CuONPs was added into the well. The plates were incubated at 25°C overnight and observed for bacterial growth. The antibacterial activity of the CuONPs was assessed based on the zone of inhibition formed around the well (CitationSivaraj et al. 2014). Experiments were repeated three times and mean values were considered.
RBC Lysis Test for CuONPs
In vitro erythrocyte lysis test was carried out as a preliminary toxicity test, which is assessed by measuring the hemoglobin released as a result of membrane leakage or disruption caused by exposure to low doses of the NPs (CitationOves et al. 2013). The extent of hemolysis was studied by incubating the RBC suspension with various concentrations of CuONPs, ranging from 50 to 200 μg/mL at 37°C for 1 h. The incubated cell suspension was centrifuged at 1500 × g for 10 min. The supernatant was analyzed by UV–vis spectroscopy (λmax = 576 nm) for hemoglobin released. The percentage hemolysis was determined by the following equation:
Abst is the absorbance of the supernatant from samples incubated with the particles, Absc is the absorbance of the supernatant from controls (normal saline), and Abs100% is the absorbance of the supernatant of controls incubated in the presence of 1% Triton® X-100, which causes complete lysis of the RBCs (total lysis).
Isolation and propagation of rat splenocytes
Adult (Sprague Dawley, 8–12 weeks old) rats were purchased from Koatech, South Korea. The rats were maintained in the specific pathogen-free facility. Fresh splenocyte of the rat was obtained by teasing the spleen under aseptic conditions according to (CitationLu et al. 1995). Single-cell suspensions were prepared from rat spleen by pressing the tissues through sterile wire mesh and washing the cells in RPMI medium containing 1% antibiotic (Anti-Anti, Gibco, South Korea). The isolated splenocytes were cultured in RPMI medium containing 10% fetal bovine serum and 1% antibiotic, maintained at a confluence of 80% and used for cytotoxicity assays (CitationAravinthan et al. 2015).
MTT assay on splenocytes
The isolated rat splenocytes were treated with series of 50–300 μg/mL phytosynthesized CuONPs in 96-well tissue culture plates. The treated cells were incubated for 48 h at 37°C with 5% CO2 for cytotoxicity analysis. The cells were then subjected for MTT assay. The stock concentration (5 mg/mL) of MTT (3-(4, 5-dimethylthiazol-2-yl)-2, 5- diphenyltetrazolium bromide, a yellow tetrazole) was prepared and 20 μL of MTT was added in each CuONP-treated well and incubated for 4 h. Purple formazone crystals were observed at the bottom of the well, and these crystals were dissolved with 200 μL of dimethyl sulfoxide (DMSO), and read at 595 nm (CitationSukirtha et al. 2012, CitationVivek et al. 2012) in a multi-well plate reader (Epoch micro plate spectrophotometer, BioTek, USA).
Results and discussion
The present study is an attempt to use Piper betle leaf extract as a bioreductant for green and rapid synthesis of CuONPs. The color of the reaction mixture turned from yellowish white to dark red after 2 h of incubation, indicating the reduction of CuSO4. The constituents present in the Piper betle leaf extract could have been responsible for the reduction of CuSO4. The intensity of the color gradually increased relative to the incubation time, and appeared darker after 10 h. However, the color of the control solution remained unchanged even after 48 h of incubation. The difference in the intensity of the color is due to excitation of surface plasmon vibrations in the CuONPs (CitationDong et al. 2014). This was supported by the UV–vis spectrum, which revealed a sharp band at 280 nm (). The results are consistent with previous studies by CitationSankar et al. (2014b) and CitationSivaraj et al. (2014), reporting the surface plasmon resonance of CuONPs at 280 nm.
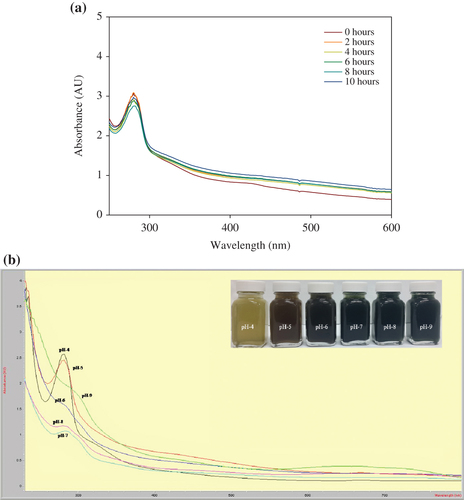
Numerous studies have reported the pH of the solution as the most important parameter influencing the formation of NPs in reaction mixture (CitationRahimi-Nasrabadi et al. 2013). Hence, CuONP formation was tested at different levels of pH, and the results are shown in . The results confirmed that CuONP formation is strongly pH-dependent, and the optimum absorbance peak was observed at a pH of 4. However, the intensity of the peak gradually decreased and broadened according to the increase in pH (5–8), and disappeared at pH 9. The differences in the peak intensity at pH 5–8 and the disappearance of peak at pH 9 could be attributed to the stability of plant compounds and NPs. However, this phenomenon requires more study. The formation of CuONPs at different time intervals is shown in . The results confirmed the formation of CuONPs within 2 h. However, a minor difference in the intensity of the peak was observed up to 10 h. The results are consistent with a previous study by CitationSankar et al. (2014b), reporting the formation of CuONPs within 90 min using Carica papaya.
The representative electron micrograph of the synthesized CuONPs is shown in . The CuONPs were mostly spherical and well dispersed, with an average size of 50–100 nm. The elemental information of CuONPs has been obtained from SEM–EDX spectroscopy (). The optical absorption peak, approximately at 1 and 8 keV, is typical for the absorption of copper oxide nanocrystallites due to surface plasmon resonance. The results are consistent with previous studies reporting the CuONP absorption peaks at 1 and 8 keV (CitationChowdhury et al. 2013, CitationSankar et al. 2014b). The XRD pattern of the CuONPs showed diffraction peaks at 2θ = 32.1, 45.84, 54.80 and 76° () that indexed the lattice planes 111, 200, and 220 of face-centered cubic structures of CuONPs. Also, the XRD pattern indicates that synthesized CuONPs were crystalline in nature (CitationEthiraj and Kang 2012). FTIR spectra of the green-synthesized CuONPs are shown in . The intense peak at 3414 cm− 1 and sharp peak at 2942 cm− 1 could be assigned to the N–H and C–H stretching of amides and alkanes, respectively. The sharp peak at 1622 cm− 1 could be ascribed to the C–O stretching of the COOH group. The peaks at 1509 and 1307 cm− 1 were due to the N–H stretching of the primary and secondary amines, respectively. The results of the FTIR studies further confirm that the compounds present in the Piper betle leaf extract reduced CuSO4 into CuONPs. The results are in agreement with previous studies reporting the involvement of plant extracts in synthesizing CuONPs (CitationSankar et al. 2014b, CitationSivaraj et al. 2014).
The green-synthesized CuONPs inhibited the growth of X. axonopodis and R. solanacearum on Muller-Hinton agar after 12 h of incubation at 25°C. The zone of inhibition for X. axonopodis and R. solanacearum were 7 ± 1 and 9 ± 1 mm, respectively. However, no inhibition was observed in the control plates. The CuONPs inhibit the growth of bacteria by damaging the cell wall of the pathogens. Our results suggest the possibility of using green-synthesized CuONPs to control phytopathogens. The results are consistent with previous studies reporting the antimicrobial activity of CuONPs (CitationSivaraj et al. 2014).
Hemolysis test was carried out as a preliminary toxicity test for hemoglobin released in the presence of CuONPs (). CuONPs significantly caused RBC lysis at 34% and 50% in 50 and 200 μg/mL, after 1 h of incubation. Thus, in vitro cytotoxicity of the CuONPs was evaluated against rat splenocytes at different concentrations (50, 100, 150, 200, 250 and 300 μg/mL), and the results are shown in (). The results clearly demonstrate that the viability of the splenocytes is directly proportional to the concentration of CuONPs. A minimum cell death (19%) was reported at 50 μg/mL, and gradually increased according to the concentration and reached the maximum (94%) at 300 μg/mL. CitationSankar et al. (2014a), also reported that CuONP cytotoxicity in A59 cells at a lower concentration (50 μg/mL) showed 70% viability, and the cell viability was decreased up to 6% at higher concentration (500 μg/mL).
Conclusion
The study reported a rapid, eco-friendly, inexpensive, and simple approach for synthesizing CuONPs using Piper betle leaf extract as the reducing agent. Stable CuONP formation was observed at pH 4 and the particles were spherical in shape. The elemental composition was confirmed by the presence of copper. Antibacterial activity of synthesized CuONPs against phytopathogens X. axonopodis and R. solanacearum suggests that it could be used as an antibacterial agent for sustainable agriculture and crop management. Results of cytotoxicity tests show that cell viability was decreased with increasing concentration (50–300 μg/mL) of CuONPs.
Acknowledgements
The research work was supported by the National Research Foundation of Korea (NRF) Grant funded by the government (MEST; No. 2011-0020202) and partially by Korea Ministry of Environment as Eco Innovation project [Project No. 2013001470006].
Declaration of interest
The authors report no declarations of interest. The authors alone are responsible for the content and writing of the paper.
References
- Aazam ES, El-Said WA. 2014. Synthesis of copper/nickel nanoparticles using newly synthesized Schiff-base metals complexes and their cytotoxicity/catalytic activities. Bioorg chem. 57:5–12.
- Abboud Y, Saffaj T, Chagraoui A, El Bouari A, Brouzi K, Tanane O, Ihssane B. 2014. Biosynthesis, characterization and antimicrobial activity of copper oxide nanoparticles (CONPs) produced using brown alga extract (Bifurcaria bifurcata). Appl Nanosci. 4:571–576.
- Ali I, Khan FG, Suri KA, Gupta BD, Satti NK, Dutt P, Khan IA. 2010. In vitro antifungal activity of hydroxychavicol isolated from Piper betle L. Ann Clin Microbiol Antimicrob. 9:1–9.
- Aravinthan A, Govarthanan M, Selvam K, Praburaman L, Selvankumar T, Balamurugan R, et al. 2015. Sunroot mediated synthesis and characterization of silver nanoparticles and evaluation of its antibacterial and rat splenocyte cytotoxic effects. Int J Nanomed. 2015:1977–1983.
- Azam A, Ahmed AS, Oves M, Khan MS, Memic A. 2012. Antimicrobial activity of metal oxide nanoparticles against Gram-positive and Gram-negative bacteria: a comparative study. Int J Nanomed. 7:3527–3535.
- Bauer AW, Kirby WM, Sherris JC, Turck M. 1966. Antibiotic susceptibility testing by a standardized single disk method. Am J Clin Pathol. 45:493–496.
- Carnes CL, Stipp J, Klabunde KJ. 2002. Synthesis, characterization, and adsorption studies of nanocrystalline copper oxide and nickel oxide. Langmuir. 18:1352–1359.
- Chowdhury MNK, Beg MDH, Khan MR, Mina MF. 2013. Synthesis of copper nanoparticles and their antimicrobial performances in natural fibres. Mater Lett. 98:26–29.
- Das D, Nath BC, Phukon P, Dolui S. 2013. Synthesis and evaluation of antioxidant and antibacterial behavior of CuO nanoparticles. Colloids Surf B. 101:430–433.
- Dong C, Cai H, Zhang X, Cao C. 2014. Synthesis and characterization of monodisperse copper nanoparticles using gum acacia. Physica E. 57:12–20.
- Ethiraj AS, Kang DJ. 2012. Synthesis and characterization of CuO nanowires by a simple wet chemical method. Nanoscale Res Lett. 7:70.
- Gunalan S, Sivaraj R, Venckatesh R. 2012. Aloe barbadensis Miller mediated green synthesis of mono-disperse copper oxide nanoparticles: optical properties. Spectrochim Acta A Mol Biomol Spectrosc. 97:1140–1144.
- Honary S, Barabadi H, Fathabad EG, Naghibi F. 2012. Green synthesis of copper oxide nanoparticles using penicillium aurantiogriseum, penicillium citrinum and penicillium wakasmanii. Digest J Nanomater Biostruct. 7:999–1005.
- Kanhed P, Birla S, Gaikwad S, Gade A, Seabra AB, Rubilar O, et al. 2014. In vitro antifungal efficacy of copper nanoparticles against selected crop pathogenic fungi. Mater Lett. 115:13–17.
- Lim S, Hudson SM. 2004. Application of a fiber-reactive chitosan derivative to cotton fabric as an antimicrobial textile finish. Carbohydr Polym. 56:227–234.
- Lu L, Hsieh M, Oriss TB, Morel PA, Starzl TE, Rao AS, Thomson AW. 1995. Generation of DC from mouse spleen cell cultures in response to GM-CSF: immunophenotypic and functional analyses. Immunology. 84:127.
- Mazura MDP, Nuziah H, Rasadah MA, Kiong LS. 2007. Evaluation of Piper betle on platelet activating factor (PAF) receptor binding activities. Malayas J Sci. 26:79–83.
- Narayanan KB, Sakthivel N. 2010. Biological synthesis of metal nanoparticles by microbes. Adv. Colloid Interface Sci. 156:1–13.
- Oves M, Khan MS, Zaidi A, Ahmed AS, Ahmed F, Ahmad E, Azam A. 2013. Antibacterial and cytotoxic efficacy of extracellular silver nanoparticles biofabricated from chromium reducing novel OS4 strain of Stenotrophomonas maltophilia. PloS one. 8:59140.
- Pin KY, Chuah AL, Rashih AA, Mazura MP, Fadzureena J, Vimala S, Rasadah MA. 2010. Antioxidant and anti-inflammatory activities of extracts of betel leaves (Piper betle) from solvents with different polarities. J Trop For Sci. 22:448–455.
- Rahimi-Nasrabadi M, Pourmortazavi SM, Davoudi-Dehaghani AA, Hajimirsadeghi SS, Zahedi MM. 2013. Synthesis and characterization of copper oxalate and copper oxide nanoparticles by statistically optimized controlled precipitation and calcination of precursor. Cryst Eng Comm. 15:4077–4086.
- Rathee JS, Patro BS, Mula S, Gamre S, Chattopadhyay S. 2006. Antioxidant activity of Piper betel leaf extract and its constituents. J Agric Food Chem. 54:9046–9054.
- Ren G, Hu D, Cheng EWC, Reus MAV, Reip P, Allaker RP. 2009. Characterisation of copper oxide nanoparticles for antimicrobial applications. Int J Antimicrob Agents. 33:587.
- Sankar R, Maheswari R, Karthik S, Shivashangari KS, Ravikumar V. 2014a. Anticancer activity of Ficus religiosa engineered copper oxide nanoparticles. Mater Sci Eng C Mater Biol Appl. 44:234–239.
- Sankar R, Manikandan P, Malarvizhi V, Fathima T, Shivashangari KS, Ravikumar V. 2014b. Green synthesis of colloidal copper oxide nanoparticles using Carica papaya and its application in photocatalytic dye degradation. Spectrochim Acta A. 12:746–750.
- Sarkar A, Sen R, Saha P, Ganguly S, Mandal G, Chatterjee M. 2008. An ethanolic extract of leaves of Piper betle (Paan) Linn mediates its antileishmanial activity via apoptosis. Parasitol Res. 102:1249–1255.
- Saterlie M, Sahin H, Kavlicoglu B, Liu Y, Graeve O. 2011. Particle size effects in the thermal conductivity enhancement of copper-based nanofluids. Nanoscale Res Lett. 6:1–7.
- Sathishkumar G, Gobinath C, Karpagam K, Hemamalini V, Premkumar K, Sivaramakrishnan S. 2012. Phyto-synthesis of silver nanoscale particles using Morinda citrifolia L. and its inhibitory activity against human pathogens. Colloids Surf B Biointerfaces. 95:235–240.
- Sivaraj R, Rahman PK, Rajiv P, Narendhran S, Venckatesh R. 2014. Biosynthesis and characterization of Acalypha indica mediated copper oxide nanoparticles and evaluation of its antimicrobial and anticancer activity. Spectrochim Acta A Mol Biomol Spectrosc. 129:255–288.
- Sukirtha R, Priyanka M, Antony JJ, Kamalakkannan S, Thangam R, Gunasekaran P, Achiraman S. 2012. Cytotoxic effect of Green synthesized silver nanoparticles using Melia azedarach against in vitro HeLa cell lines and lymphoma mice model. Process Biochem. 47:273–279.
- Usha Rani P, Rajasekharreddy P. 2011. Green synthesis of silver-protein (core–shell) nanoparticles using Piper betle L. leaf extract and its ecotoxicological studies on Daphnia magna. Colloids Surf A. 389:188–194.
- Vivek R, Thangam R, Muthuchelian K, Gunasekaran P, Kaveri K, Kannan S. 2012. Green biosynthesis of silver nanoparticles from Annona squamosa leaf extract and its in vitro cytotoxic effect on MCF-7 cells. Process Biochem. 47:2405.
- Wang H, Xu JZ, Zhu JJ, Chen HY. 2002. Preparation of CuO nanoparticles by microwave irradiation. J Cryst Growth. 244:88–94.
- Zain NM, Stapley AGF, Shama G. 2014. Green Synthesis of Silver and Copper Nanoparticles using Ascorbic acid and Chitosan for Antimicrobial Applications. Carbohydr Polym. 112:195–202.
- Zhang Q, Li Y, Xu D, Gu Z. 2001. Preparation of silver nanowire arrays in anodic aluminum oxide templates. J Mater Sci Lett. 20: 925–927.