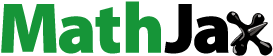
Abstract
The aim of the present study was to develop solid lipid nanoparticles (SLN) and formulate a hydrogel for enhanced topical delivery of aceclofenac (ACF). The SLN were prepared by the ultrasonic emulsification method and optimized on the basis of stirring speed and lipid content. The optimized formulation was characterized for particle size (189 ± 9.2 nm), polydispersity index (PDI) (0.162 ± 0.02), zeta potential (−32.51 ± 0.12 mV), entrapment efficiency (86.51 ± 2.46%), surface morphology, differential scanning calorimetry (DSC) and X-ray diffraction (XRD). In vivo performance of ACF-loaded SLN hydrogel showed prolonged inhibition of edema, as compared to that observed using plain ACF hydrogel, after 24 h. The results demonstrated that the ACF–SLN formulation for skin targeting could be a promising carrier for topical delivery of ACF.
Introduction
Aceclofenac (ACF) is a non-steroidal anti-inflammatory drug (NSAID) and has been indicated orally for the relief of pain and inflammation in osteoarthritis, rheumatoid arthritis, and ankylosing spondylitis. The oral administration of ACF produces side effects like gastric irritation, ulcer, abdominal pain, and flatulence on chronic use (CitationChakraborty et al. 2010, CitationDua et al. 2010). In order to achieve better efficiency and high compliance with the therapy, these agents should be administered once daily and their therapeutic action should last for 24 h even with a daily single-dose administration. A controlled-release formulation could be a better option that will provide prolonged action, with minimum side effects and improved patient compliance (CitationBeaulieu et al. 2008, CitationPareek et al. 2011).
Solid lipid nanoparticles (SLN) are novel controlled-release carriers that have been proposed for various routes of administration such as parenteral, oral and topical (CitationMuller et al. 1995). They are made up of biocompatible and biodegradable material, with the capability to incorporate lipophilic and hydrophilic drugs (CitationJenning et al. 2000, CitationMai et al. 2000). In the last decade, these carriers have gained wide interest in topical administration due to their occlusive properties or film formation on the skin surface (CitationPuglia et al. 2012). They also reduce the transepidermal water loss and may enhance the penetration of drugs through the stratum corneum (SC) by increased hydration (CitationMei et al. 2005, CitationChen et al. 2006, CitationLiu et al. 2007). SLN not only function as an alternative carrier but are also well suited for dermal application on inflamed skin, because of the non-irritant and nontoxic lipid content (CitationJenning and Gohla 2001, CitationSouto et al. 2004a). Moreover, SLN could be formulated into creams (Citationde Vringer and de Ronde 1995, CitationJenning et al. 2000) and hydrogels (CitationSouto et al. 2004b, CitationShah et al. 2007, CitationChawla and Saraf 2012) without altering their properties, for better skin retention and improved topical delivery.
The present investigation was aimed to develop and characterize ACF-loaded SLN, and incorporate them into a hydrogel. SLN were prepared using the ultrasonic emulsification technique, with glyceryl monostearate (GMS) as the solid lipid. The results of in vitro skin permeation and in vivo anti-inflammatory studies indicate that the ACF-loaded SLN formulation may be a promising carrier for topical delivery of ACF.
Materials and methods
Materials
ACF was obtained as a generous gift sample from Cipla Laboratories (Mumbai, India). Glyceryl monostearate (GMS) and Carbopol 934 were purchased from Loba Chemie (India). Soya lecithin was purchased from Sigma-Aldrich (USA). Tween 80 was supplied by Merck Limited (Mumbai, India). All other chemicals used were of analytical grade.
Preparation of ACF-loaded SLN
SLN were prepared by the previously reported ultrasonic emulsification technique, with slight modifications (CitationCastelli et al. 2005, CitationLuo et al. 2006). Briefly, GMS and ACF were dissolved in a minimum quantity of absolute ethanol at 50 ± 1°C. After evaporation of ethanol, the melt was dispersed in preheated water (50 ± 1°C) containing a mixture of emulsifier (soya lecithin and Tween 80, at a ratio of 1:1) under mechanical stirring (Remi, India) at 4000 rpm for 10 min. The resulting emulsion was subjected to ultrasonication using a probe sonicator (Soniweld, India) for 6 min at 40 watts. Further, the dispersion was immediately dispersed in cold distilled water (4 ± 1°C), followed by continued mechanical stirring for 10 min. The suspension was then filtered through a 0.45 μm filter to remove impurities from the material. The influence of process parameters (i.e., stirring speed and lipid concentration) on particle size are shown in and , respectively.
SLN characterization
Particle size and zeta potential
The average particle size and size distribution of the SLN were determined by photon correlation spectroscopy using a Zetasizer DTS version 4.10 (Malvern Instruments, UK). Measurements were performed in distilled water adjusted with a solution of sodium chloride at a concentration of 0.1 mmol/L, to a conductivity of 50 μS/cm at 25°C. The surface charge of SLN was determined by measuring zeta potential of the lipid nanoparticles.
Particle shape and surface morphology
Transmission Electron Microscopy (TEM) (Philips CM12 Electron Microscope, Eindhoven, The Netherlands) was used for the determination of SLN morphology. The aqueous dispersion (one drop) was placed over a 400 mesh carbon-coated copper grid followed by negative staining with phosphotungstic acid solution. For the scanning electron microscopy (SEM) examination, the SLN were dried for 24 h before the analysis. The prepared samples were characterized for shape and surface structure by SEM (Leo 435 VP, Cambridge, UK).
X-ray diffraction (XRD)
The internal crystalline structure was investigated by small-angle XRD. Copper Kα X-rays (1.542 A°) were produced by X-ray generation (FLCU 4KE, Bruker, Germany) and operated at 40 KV and 45 mA. Experiments were performed in triplicate.
Differential Scanning Calorimetry (DSC)
DSC was used to investigate the melting and recrystallization behavior of crystalline materials like SLN (CitationHou et al. 2003). The transition temperature of lipid nanoparticles was measured in triplicate using modulated DSC (TA Instruments 2910) with a programmed heating rate of 10°C/min.
Entrapment Efficiency
The pH value of the SLN dispersion obtained was adjusted to 1.2 with 0.1 M HCl to form an aggregation of nanoparticles. These aggregated SLN were centrifuged at 25 000 rpm (Z36 HK, Hermle Labortechnik, Germany) for 30 min. Then, entrapment efficiency was determined () using the HPLC method (CitationMusmade et al. 2007) and calculated from the following equation:
Table I. Optimized parameters of ACF-loaded SLN.
Formulation and characterization of ACF-loaded SLN hydrogel
For preparation of ACF-loaded SLN hydrogel, Carbopol 934 (1 g) was dispersed in SLN dispersion (equivalent to 1.5 g of ACF) followed by the addition of glycerin (10 ml). After 12 h, triethanolamine (0.4 ml) was added and gently stirred with a glass rod to avoid inclusion of air. Similarly, plain ACF-loaded hydrogel was also prepared.
The prepared gel was characterized for spreadability by placing 1 g of gel in 1 cm2 on a glass plate, with a second glass plate placed over it. A weight of 50 grams was then applied on the second glass plate for 5 min, and the increase in the diameter of the gel sample due to spreading was noted.
In vitro skin permeation study
In vitro skin permeation of the prepared formulations was studied using a locally fabricated Franz diffusion cell with an effective permeation area and receptor cell volume of 1.0 cm2 and 10 mL, respectively (CitationShakeel et al. 2007 and CitationLopes et al. 2006). The temperature was maintained at 32 ± 1°C. The receptor compartment contained 10 mL of phosphate buffer (pH 6.5) and was constantly stirred at 100 rpm on a magnetic stirrer (Expo India Ltd, Mumbai, India). Rat skin of full thickness (Sprague-Dawley, 120–150 g) was excised from the abdominal region, and hair was removed. The subcutaneous tissue was removed surgically and the dermis side was wiped with isopropyl alcohol to remove adhering fat. The cleaned skin was washed with distilled water and stored at − 20°C until further use. The skin was then carefully checked through a magnifying glass to ensure that samples were free from any surface irregularities such as tiny holes or crevices in the portion that was used for transdermal permeation studies. After assurance, the skin was mounted on a receptor compartment with the stratum corneum (SC) side facing upward into the donor compartment. The formulation was applied on the skin from the donor-compartment side. Samples were withdrawn through the sampling port of the diffusion cell at predetermined time intervals over 24 h, and analyzed. The receptor medium was immediately replenished with equal volume of fresh buffer. The amount of ACF in the samples was analyzed by HPLC.
Skin retention study
To determine the amount of drug retained in the hairless rat skin, the skin stripping experiment was performed using protecting tape (Scotch Magic Tape, India) (CitationJayaraman et al. 1996, CitationWissing and Muller 2002). The formulation was applied to the skin uniformly and occluded for 24 h. After occlusion, the residual formulation was removed by cotton, followed by washing with water, and then air dried. The first strip was set for removing the SC layer of skin. The same skin was subsequently stripped another 10 times (). The remaining skin was homogenized in methanol, followed by centrifugation for 10 min at 7000 rpm. The content of drug in both tapes and in remaining skin was determined by the HPLC method.
Table II. Skin retention studies of different formulations (tape-stripping experiment).
High-Performance Liquid Chromatography (HPLC) analysis of ACF
The HPLC analysis of ACF was done as reported elsewhere (CitationMusmade et al. 2007). Briefly, mobile phase methanol/triethyl amine (0.3% in deionized water, pH 7.0) at a ratio of 60/40 v/v was run at a flow rate of 1 mL/min, and detection was carried out at 275 nm.
Confocal Laser Scanning Microscopy (CLSM)
Skin penetration of Rhodamine Red (RR)-loaded SLN was investigated using CLSM, as reported previously (CitationSchatzlein and Leve 1998, CitationAlvarez-Roman et al. 2004, CitationDubey et al. 2006). Sprague-Dawley albino rats (120–150 g) were used, and the study protocol (Animal Eths. Comm./07/274) was approved by the Institutional Animal Ethical Committee of Dr. Harisingh Gour University, Sagar. Briefly, RR-loaded SLN hydrogel was applied non-occlusively for 24 h to the dorsal skin of 5–6 week-old nude albino rats. The full skin thickness was optically scanned at different increments through the z-axis of a CLS microscope (LSM 510 attached with a Zeiss universal epifluorescence microscope) (Carl Zeiss, Jena, Germany).
In vivo anti-inflammatory activity
In vivo anti-inflammatory activity was determined using a rat paw model of carrageenan-induced edema. Male albino rats (Sprague-Dawley, 120–150 g) were randomly divided into three groups containing six rats in each group. Each weighed rat was marked on the right hind paw, just behind the targal junction, to ensure constant paw volume. Different formulations were applied to the shaved abdominal rat skin according to body weight. The control group was treated with normal saline (0.9% NaCl). Carrageenan (0.1 mL, 1% w/v) was injected in the subplantar region of the right hind paw of the rats after 30 min of application of each sample. The initial observation just after injection and the subsequent paw volume were measured for up to 6 h with 1-h intervals, and again after 24 h. The percent rate of edema and rate of inhibition were calculated for each group using the following equations:
where V0 = the mean paw volume before carrageenan injection (mL), Vt = the mean paw volume after carrageenan injection (mL), Ec = the edema rate of control group, and Et = the edema rate of treated group.
Statistical Analysis
The results were expressed as mean values ± standard deviation (SD). The statistical analysis was done by using the Student's t-test and one-way analysis of variance (ANOVA). A probability level of P < 0.05 was considered to be significant.
Result and discussion
Preparation of SLN
The biocompatibility and acceptability of GMS for oral or topical use has been well documented and hence used for preparation of SLN (CitationRowe et al. 2002). To obtain nanosized SLN for topical application of ACF, the effects of various parameters were studied. The stirring speed during SLN formulation was varied from 1000 to 6000 rpm, and the results demonstrate a reduction in particle size with an increase in stirring speed (); 4000 rpm was selected as the optimum speed that yielded particles with a size of 178 ± 7.13 nm and the lowest PDI (0.14 ± 0.03). Further increase in stirring speed resulted in increased PDI due to formation of particles with a range of different sizes, hence 4000 rpm was considered to be optimal.
The effect of GMS concentration on particle size and size distribution (at 4000 rpm) was studied, and it was observed that enhancing GMS concentration from 1% to 3% produced no significant effect (P > 0.05), either on particle size or on PDI (). Further increase in GMS concentration led to an abrupt increase in particle size and PDI, possibly due to the unavailability of more surfactant (Tween 80 and soya lecithin) to exert its effect on particle size regulation. Hence, the parameters of 3% GMS and 4000 rpm stirring speed were considered as optimal for SLN formulation, because they led to the formation of particles with a size of 189 ± 9.2 nm and the lowest PDI (0.16 ± 0.02). The zeta potential of the optimized formulation was − 32.51 ± 0.12 mV.
Entrapment efficiency
Relatively higher drug entrapment is one major advantage of SLN, and the less crystalline state of lipid structure could offer space to accommodate the drug (CitationMuller et al. 2002). High entrapment efficiency (86.51 ± 2.46%) of ACF was obtained with SLN, possibly due to the lipophilic nature of drug.
Surface morphology
TEM and SEM images of the optimized SLN have been presented in , respectively. Both techniques confirmed that the SLN were spherical in shape and well dispersed. The size of the SLN, determined by the Zetasizer, was found to be smaller than that shown by TEM results. TEM images of SLN show spherical and homogeneous particles ranging approximately from 150 to 250 nm in size, and the SEM photograph suggest that the SLN possessed a slightly rough surface.
X-ray diffraction (XRD)
The XRD patterns of ACF, bulk lipid, and drug-loaded SLN are shown in , revealing significant difference between diffraction of ACF and drug-loaded SLN. It could be inferred via XRD that the ACF existed in the amorphous form, because of the absence of a sharp peak of ACF in the diffraction pattern of drug-loaded SLN. The XRD of SLN was broader and much weaker than that of bulk lipid, confirming that the GMS in SLN was partially recrystallized.
Differential scanning calorimetry (DSC)
DCS is a plot of heat flux (rate) versus temperature at a specified temperature. Polymorphic transformation of the lipid drug delivery system may occur during the preparation of the dosage form. Therefore, the DSC thermograms of ACF, GMS, plain SLN, and drug-loaded SLN were investigated (). DSC graph confirmed that ACF presented a sharp peak at 185°C, corresponding to the melting point behavior of the crystalline form of the drug, and GMS presented a sharp peak at 55.46°C. The thermogram of drug-loaded SLN did not show the melting peak of crystalline ACF at around 185°C, which indicated that ACF in SLN was in the amorphous state. The bulk lipid melted at 55.46°C. However, the melting peak of ACF-loaded SLN decreased by about 3.21°C, suggesting the formation of less ordered lipid crystals due to embedding of the drug within. The decline in melting point of SLN is compared to that of pure drug, can be assigned to the colloidal dimension of the particles, particularly to their large surface to volume ratio. The XRD patterns of different substances were in good agreement with results obtained by DSC measurements.
Preparation and evaluation of SLN-loaded hydrogel
The SLN aqueous dispersion could be formulated into the hydrogel consisting of nearly uncharged polymer, without significant change in the particle size and zeta potential (CitationSouto et al. 2004b). Because of the neutralization of the carboxylic group of carbopol with NaOH, reduced zeta potentials of particles and ions caused destabilization of the SLN dispersion (CitationMei et al. 2005). Hence, triethanolamine (organic base) was used as a neutralizing agent to improve the stability of SLN. The SLN-loaded hydrogel was further evaluated for pH and spreadability. The diameter of spreading was found to be 6.7 ± 0.2 cm, which indicates good spreadability (), while the pH was similar to that of skin as seen with the use of conventional gel, indicating no skin irritation.
Table III. pH and spreadability of different hydrogels.
Skin permeation studies
After application of ACF-loaded SLN hydrogel for 24 h, only 39.32 ± 3.4% of the applied dose was found to have permeated through the skin (hairless rat skin), while 30.64 ± 4.3% of drug had permeated when the ACF gel was used (). The standpoints about penetration of drug-loaded SLN into skin are its occlusive effect and the small size of the lipid particles (high specific surface area), which ensure close contact with the SC. The small particle size may help in a stronger occlusive effect due to the membrane formation of SLN (CitationSouto et al. 2004a). In addition, the interaction of SLN lipids and surfactant with skin lipid is an important factor affecting the degree of penetration (CitationMuller et al. 2002). These data also suggest good retention of drug in the epidermal layer. The data show that initially, drug penetration with SLN-loaded gel was slightly lower than that with ACF gel. After 6 h, increased drug penetration due to polymorphic transition of the lipid matrix correlated with drug release, since different polymorphic forms differ in their ability to hold host molecules (CitationMai et al. 2000, CitationBunjes et al. 1996). Thus, drug expulsions may likely occur and expel ACF, which is poorly water soluble, and may increase thermodynamic activity, which leads to skin permeation.
Skin retention studies (Tape stripping)
The amount of ACF in hairless rat skin was found to be 8.71 ± 0.61 μg/cm2 with ACF gel and 25.31 ± 4.80 μg/cm2 with ACF-loaded SLN gel. The three-fold increase in skin retention was attributed to the higher lipophilicity of ACF and the possible effect of SLN on skin.
Confocal laser scanning microscopy
The extent of penetration of SLN, measured by CLSM microscopy 24 h after application over skin, clearly depicted the topical/transdermal potential of this carrier. The use of RR-loaded SLN increased both the depth of penetration up to 120 μm, and the fluorescence intensity, as compared to the RR solution that penetrated to a depth of only a few micrometers, with very low fluorescence (). Enhanced penetration of RR-loaded SLN could possibly be due to its structural similarity and close contact with the SC, providing good epidermal targeting of dye.
In vivo anti-inflammatory activity
In vivo performance assays of simple gel and SLN-loaded gel were carried out in a rat paw model of carrageenan-induced edema (CitationWinter 1965). The SLN-loaded hydrogel not only decreased inflammation by a large magnitude, but also prolonged the effect. The extent of inhibition of carrageenan-induced edema by different formulations is shown in . The SLN-loaded hydrogel provided maximum inhibition at 6 h, with a magnitude of 81% after. The simple gel provided prolonged effect, but showed lower anti-inflammatory magnitude than the SLN-loaded hydrogel.
Conclusion
The optimized protocol of the ultrasonic emulsification method was successfully applied for preparation of ACF-loaded SLN. Furthermore, the ACF-loaded SLN gel provided higher localization of drug in the epidermal layer as compared with the simple hydrogel. The size of the SLN and close contact with the SC proved enhanced skin deposition of drug. The SLN-loaded hydrogel achieves inhibition of edema with a magnitude of 81% after 6 h. The final results suggest that ACF-loaded SLN could have promising potential as an alternative against conventional topical delivery of ACF.
Acknowledgement
The authors are grateful to the University Grants Commission (New Delhi, India) for providing financial assistance as JRF. The authors also thankful to NIPER, Chandigarh, India for providing use of the Zetasizer facility, and AIIMS, New Delhi, India for providing TEM and SEM facilities.
Declaration of interest
The authors report no declarations of interest. The authors alone are responsible for the content and writing of the paper.
References
- Alvarez-Roman R, Naik A, Kalia YN, Fessi S, Guy RH. 2004. Visualisation of skin penetration using confocal laser scanning microscopy. Eur J Pharm Biopharm. 58:301–306.
- Beaulieu AD, Peloso PM, Haraoui B, Bensen W, Thomson G, Wade J, et al. 2008. Once-daily, controlled-release tramadol and sustained-release diclofenac relieve chronic pain due to osteoarthritis: A randomized controlled trial. Pain Res Manage. 13:103–110.
- Bunjes H, Westensen K, Koch MHJ. 1996. Crystallization tendency and polymorphic transition in triglyceride nanoparticles. Int J Pharm. 129:159–173.
- Castelli F, Puglia C, Sarpietro MG, Rizza L, Bonina F. 2005. Characterization of indomethacin-loaded lipid nanoparticles by differential scanning calorimetry. Int J Pharm. 304:231–238.
- Chakraborty S, Khandai M, Sharma A, Khanam N, Patra CN, Dinda SC, Sen KK. 2010. Preparation, in vitro and in vivo evaluation of algino-pectinate bioadhesive microspheres: an investigation of the effects of polymers using multiple comparison analysis. Acta Pharm. 60:255.
- Chawla V, Saraf SA. 2012. Rheological studies on solid lipid nanoparticle based carbopol gels of aceclofenac. Coll Sur B: Biointerfaces. 92:293–298.
- Chen H, Chang X, Du D, Liu W, Liu J, Weng T, et al. 2006. Podophyllotoxin-loaded solid lipid nanoparticles for epidermal targeting. J Control Rel. 110:296–306.
- de Vringer T, de Ronde HAG. 1995. Preparation and structure of water in oil cream containing lipid nanoparticles. J Pharm Sci. 84:466–472.
- Dua K, Pabreja K, Ramana MV. 2010. Aceclofenac topical dosage forms: in vitro and in vivo characterization. Acta Pharm. 60:467.
- Dubey V, Mishra D, Asthana A, Jain NK. 2006. Transdermal delivery of a pineal hormone: melatonin via elastic liposomes. Biomaterials. 27:3491–3496.
- Hou Z, Xie C, Haung K, Zha C. 2003. The production and characteristics of solid lipid nanoparticles (SLNs). Biomaterials. 24:1781–1785.
- Jayaraman CS, Ramachandran C, Weiner N. 1996. Topical delivery of erythromycin from various formulations: an in vivo hairless mouse skin. J Pharm Sci. 85:1082–1085.
- Jenning V, Gohla S. 2001. Encapsulation of retinoids in solid lipid nanoparticles. J. Microencap.18:149–158.
- Jenning V, Gysler A, Schafer-Korting M, Gohla SH. 2000. Vitamin A loaded solid lipid nanoparticles for topical use: occlusive properties and drug targeting to the upper skin. Eur J Pharm Biopharm. 49:211–218.
- Liu J, Hu W, Chen H, Ni Q, Xu H, Yang X. 2007. Isotretinoin-loaded solid lipid nanoparticle with skin targeting for topical delivery. Int J Pharm. 328:191–195.
- Lopes LB, Ferreira DA, Poula D, Garcia MJ, Thiomazini JA, Fantini MA, Bentley MB. 2006. Reverse hexagonal nanodispersion of monoolean and oleic acid for topical delivery of triptolide: in vitro and in vivo skin penetration of cyclosporine A. Pharm Res. 23:1332–1342.
- Luo Y, Chen D, Ren L, Zhao X, Qin J. 2006. Solid lipid nanoparticles for enhancing vinpocetine’s oral bioavailability. J Control Rel. 114:53–59.
- Mai CS, Mehnert W, Schäfer-Korting M. 2000. Solid lipid nanoparticles as drug carriers for topical glucocorticoids. Int J Pharm. 196:165–167.
- Mei Z, Wu Q, Hu S, Li X, Yang X. 2005. Triptolide loaded solid lipid nanoparticle hydrogel for topical application. Drug Develop Ind Pharm. 31:161–168.
- Muller RH, Mehnert W, Lucks JS, Schuwarz C, Muhlen ZA, Weyners H, et al. 1995. Solid lipid nanoparticles (SLN) an alternative colloidal carrier system for controlled drug delivery. Eur J Pharm Biopharm. 41:62–69.
- Muller RH, Radtke M, Wissing SA. 2002. Solid lipid nanoparticles (SLN) and nanostructured lipid carrier (NLC) in cosmectic and dermatological preparation. Adv Drug Deliv Rev. 54:S131–135.
- Musmade P, Subramanian G, Srinivasan KK. 2007. High performance thin layer chromatography and pharmacokinetic of aceclofenac in rats. Analytica Chem Acta. 585:1082–1085.
- Pareek A, Chandurkar N, Gupta A, Sirsikar A, Dalal B, Jesalpura B, et al. 2011. Efficacy and Safety of Aceclofenac-CR and Aceclofenac in the Treatment of Knee Osteoarthritis: A 6-Week, Comparative, Randomized, Multicentric, Double-Blind Study. The J Pain.12:546–553.
- Puglia C, Bonina F, Rizza L, Blasi P, Schoubben A, Perrotta R, et al. 2012. Lipid nanoparticles as carrier for octyl-methoxycinnamate: in vitro percutaneous absorption and photostability studies. J Pharma Sciences. 101:301–311.
- Rowe RC, Shesky PJ, Weller PJ. 2002. Glyceryl monostearate. In: Rowe RC, Sheskey PJ, Cook WG, et al. Handbook of Pharmaceutical Excipient, 4th Ed. New York: Pharmaceutical Press, pp. 264–265.
- Schatzlein A, Leve G. 1998. Non uniform cellular packing of the stratum corneun and permeability barrier fuction of the intact skin, high resolution confocal scanning microscopy study using highly deformable vesicles (tranferosome). British J Dermat. 138:583–592.
- Shah AK, Date AA, Joshi MD, Patravale VB. 2007. Solid lipid nanoparticles (SLN) of tretinoin: potential in topical delivery. Int J Pharm. 345:163–171.
- Shakeel F, Baboota S, Ahuja A, Ali J, Aquil M, Shafiq S. 2007. Nanoemulsion as vehicles for transdermal delivery of acelofenac. AAPS Pharm SciTech. 8:E1–9.
- Souto EB, Wissing SA, Barbosa CM, Muller RH. 2004a. Development of controlled release formulation based on SLN and NLC for topical clotrimazole delivery. Int J Pharm. 278:71–78.
- Souto EB, Wissing SA, Barbosa CM, Muller RH. 2004b. Evaluation of the physical stability of SLN and NLC before and after incorporation into hydrogel formulations. Eur J Pharm Biopharm. 58:83–90.
- Winter CA. 1965. Anti-inflammatory testing methods: comparative evaluation of indomethacin and other agents. Nonsteroidal Anti-inflmmat Drug. 52:190–202.
- Wissing A, Muller RH. 2002. Solid lipid nanoparticles as carrier for sunscreens: in vitro release and in vivo skin penetration. J Control Rel. 81:225–233.