Abstract
Studies on the effect of curcumin/PLGA ratio (CPR), stabilizer (PVA) concentration, homogenization speed, homogenization time, and sonication time on mean particle size (MPS) and percentage drug encapsulation (PDE) were performed using the multivariate technique. MPS and PDE were found to be more dependent on the interaction of sonication time with the other variables. Curcumin was released in a sustained manner from curcumin-PLGA nanoparticles (CPN). CPN improved the survival rate of Ehrlich ascites carcinoma (EAC)-bearing mice and controlled the EAC-induced change in hematological parameters. Histopathology of vital organs showed that the formulation was safe. Polycaprolactone was used in preparing an in situ implant containing CPN.
Introduction
Curcumin is a broad-spectrum chemotherapeutic agent useful in treating a wide range of pathological conditions including cancer and inflammation (CitationTabatabaei Mirakabad et al. 2014). Curcumin, in spite of its wide range of pharmacological activities, is highly safe even at high doses (CitationGosangari and Watkin 2012). Reduced solubility and poor bioavailability restrict the clinical use of curcumin. A number of delivery systems including supersaturable formulations (CitationGosangari and Dyakonov 2013), liposomes (CitationGosangari and Watkin 2012), nanosuspensions (CitationGao et al. 2010), and many polymer-based delivery systems which include chitosan (CitationChuah et al. 2013), PLGA (CitationKhalil et al. 2013) and PCL (CitationRaveendran et al. 2013) are continuously being developed and evaluated to circumvent the limitations associated with in vivo use of curcumin. Many studies show that polymer-based curcumin nanoparticles could be one of the best delivery systems to improve the bioavailability of curcumin (CitationYallapu et al. 2012).
Although the PLGA-based nanoparticulate delivery systems are effective in improving bioavailability and achieving a better efficacy of poorly soluble drugs (CitationKhalil et al. 2013, CitationKasinathan et al. 2014b), it is important that the variables involved in preparation of these formulations are properly evaluated so that a consistent product with less batch-to-batch variation is produced. With regulatory agencies insisting on using quality by design (QbD) in the development and evaluation of a formulation, it has become compulsory to optimize the conditions using statistical tools (CitationLionberger et al. 2008). Design of experiments (DoE) is one of the mathematical models available for systematic evaluation of variables involved in any process (CitationGuideline 2009). DoE represents a multivariate approach in identifying the conditions for achieving a product with the highest quality attributes and less batch-to-batch variations (CitationKasinathan et al. 2014a). Although DoE has its own pitfalls, it helps in mining and analyzing complex information in real time, besides improving the understanding of the process in a short time (CitationFerreira and Tobyn 2014). These mathematical models will ensure that the “quality is built into the product”, and allow one to achieve the predetermined quality at each step (CitationGuideline 2009, CitationNarayanan et al. 2014). Although curcumin-PLGA nanoparticles (CPNs) were previously reported, very few studies on optimizing the conditions using statistical models are available (CitationRanjan et al. 2012). Apart from meeting the regulatory requirements, mathematical model-based optimization will help in achieving the reproducible results during scale-up (CitationRanjan et al. 2012). CPNs can be prepared by various methods such as solid/oil/water emulsion technique (CitationRanjan et al. 2012) and nanoprecipitation (CitationAnand et al. 2009). Among the various methods used in the preparation of nanoparticles, the emulsion-based technique using homogenization as the mechanical force is widely used (CitationPiñón-Segundo et al. 2012). The homogenization technique, using a high-speed homogenizer, is one of the “mechanical reduction methods based on a top-down approach” currently approved by the US FDA (CitationLeleux and Williams 2013).
Although there are a few studies that describe the effect of variables involved in preparing CPN (CitationRanjan et al. 2012), a lot of information is still required as the process variables and the dependent variables studied differ depending on the preparation technique used and the outcome to be achieved. In the present study, CPNs were prepared using the emulsion–solvent evaporation technique. The effect of process variables on the outcome was investigated using factorial design and Box–Behnken design (BBD). The ability of nanoparticles to control tumor progression was studied using the EAC model in Swiss albino mice. A PCL-based in-situ implant containing CPNs was prepared using acetone as a model solvent.
Materials and methods
Chemicals
PLGA 75:25 (poly(lactic-co-glycolic acid)) was procured from Wako Pure Chemical Industries, Ltd., VA, USA (PLGA-7505). PCL (molecular weight 70 000–90 000 by GPC), polyvinyl alcohol (PVA) (cold water-soluble) was obtained from Sigma, St. Louis, MO, USA. Curcumin (95% purity) was bought from Venbiotech Private Limited, India. Analytical grade solvents were procured from Merck, Mumbai, India.
Method
Experimental design
The role of process variables in preparing CPNs was studied using experimental design. Initially, the significance and percentage contribution of the curcumin/PLGA ratio (CPR), PVA concentration (% w/v), homogenization speed (rpm) (HS), and homogenization time (min) (HT) respectively, on size, were studied using factorial design. These four variables were analyzed using a study design consisting of eight runs with zero blocks and zero center points.
Two variables from the selected four variables that had maximum influence on the outcome (in terms of percentage contribution and level of significance) were selected for further studies along with the third variable, sonication time. The effect of interaction of the selected three variables at various levels on the dependent variables, namely, mean particle size (MPS) and percentage drug encapsulation (PDE) was investigated using the BBD. During BBD, the levels of the other two variables that were a part of the factorial design were fixed at the levels determined at the end of the factorial study. The selected three factors were studied using the design matrix consisting of 15 runs with 3 points.
Preparation of curcumin-PLGA nanoparticles
CPNs were prepared by the emulsion–solvent evaporation technique. Both the drug (curcumin 10 mg) and the polymer (PLGA) () were completely solubilized in 10 ml of dichloro methane (DCM). The mixture of drug–polymer was added slowly into the stabilizer (PVA, 20 ml), kept under high speed homogenization (Polytron Mixer, Kinematica, Luzen, Switzerland), and homogenized for a specific period of time (), to allow the formation of emulsion. Depending on the study, namely, factorial or BBD, the speed was adjusted to the level indicated in and . The emulsion mixture was maintained overnight under constant revolutions (150 rpm, 30°C) in an orbital shaking incubator (CIS-24, Remi Instruments Ltd., India) and DCM was allowed to evaporate. The mixture containing drug–polymer stabilized with PVA was sonicated at 65% amplitude control (10 s during factorial studies, and as detailed in during CCD), after which it was centrifuged at low speed (2500 rpm for 3 min) to remove coarse particles and unentrapped drug. The nanoparticles present in the supernatant were collected by centrifuging at 34,600 g for 60 min (Sigma Laborzentrifugen 3k30, Germany) and suspended in Milli-Q water (Millipore, Bangalore, India). The suspension was once again sonicated for a brief time (65% amplitude control for 5 s). The particle size distribution was then analyzed using a Malvern zeta sizer (Zetasizer Nano ZS, Malvern Instruments, Malvern, UK). For analyzing percentage drug encapsulation, the supernatant collected after removing the coarse particles was lyophilized (Ilshin Corp., Korea) at − 45°C for 28–30 h. Freeze-dried cake was solubilized in DCM to dissolve the drug and the polymer. DCM was allowed to evaporate at 40°C. The absorbance of curcumin was measured at 422 nm (Biospec-1601, Shimadzu, Japan), after dissolving the free drug in methanol. The quantity of the drug was calculated by matching with the standard curve.
Table I. Factorial design showing the design matrix and effect of independent variables on mean particle size.
Table II. Box–Behnken design showing the effect of selected variables on mean particle size and percentage drug encapsulation.
Drug release
The amount of curcumin released from curcumin-PCL nanoparticles was analyzed using the vial method (CitationKhalil et al. 2013). Phosphate buffered saline (PBS) (2 ml, pH 7.4) was added to each of the vials containing a specific quantity of lyophilized cake of CPNs (the amount of curcumin present was 1 mg) and mixed thoroughly. The vials were placed in an orbital shaker incubator (Scigenics Ltd, India) under controlled conditions (37°C, 120 rpm). At specific intervals, the vials were removed and the contents were centrifuged (22,000 rpm for 60 min) to pellet out the nanoparticles. The supernatant was replaced with a fresh quantity (2 ml) of PBS and the pellet was completely redispersed. The vials were placed back in the shaker until the next sampling time. The amount of curcumin present in the supernatant was estimated at 422 nm (Biospec-1601, Shimadzu, Japan).
Animal studies
Acute toxicity studies were not performed, as a few studies showing the efficacy of CPNs using other animal models were already reported (CitationKhalil et al. 2013, CitationAnand et al. 2009).
The in vivo efficacy of CPNs in controlling tumor was studied using the EAC model. Swiss albino mice were used for the studies. The studies were carried out after getting approval from the Institutional Animal Ethical Committee (IAEC/KMC/66/2013). Experiments on animals were performed as per the standard protocol stated under the guidelines of Committee for Purpose of Control and Supervision of Experiments on Animals (CPCSEA). All the Swiss albino mice used in the experiment were bred in-house. Animals of 9 ± 1 weeks old weighing 23–32 g (at the start of the experiments) were selected for the studies. The animals were kept under controlled conditions of environment and were provided with regular pellet diet and water ad libitum. Group I (normal) consisted of eight animals with no tumor induction (and no treatment). On the day of experiment (day 0), viable EAC cells (2.5 × 106 cells) were injected (intraperitoneal) in the remaining 24 mice. The animals injected with EAC cells were randomly distributed into three groups, namely, the EAC control (group II)–no intervention, standard drug treatment group (curcumin) (group III), and curcumin-PLGA treatment group (group IV). Plain curcumin (40 mg/kg body weight) (CitationPal et al. 2001) and CNPs (20 mg/kg body weight suspended in PBA) were given (CitationRanjan et al. 2013) on day 1, day 4, and day 7 through the intraperitoneal route. Starting from day 1, development and progression of the tumor were monitored by recording the change in the body weight of the animals until day 14.
Hematological parameters, namely, hemoglobin (Hb), RBC, and WBC levels were analyzed using an automatic hematology analyzer (PCE-210VET, Erma Inc., Tokyo) from the blood sampled from the retro-orbital plexus. On day 14, three animals in each group were sacrificed to record the tumor volume. Major organs (liver, spleen, and kidney) were collected from these sacrificed animals for histopathological section. The remaining animals were used for studying the mean survival time (MST) and percentage increase in mean life span (IMLS). The statistical analysis (ANOVA, Tukey's post hoc test) was performed using GraphPad Prism (V.5) software (InStat Software, San Diego, CA, USA trial version). A value of p less than 0.05 was considered significant (CitationKoganti et al. 2013).
PCL-based in situ implant
An in situ implant containing CPN was developed using PCL as a carrier system. The amount of PCL required to achieve 25% w/v was dissolved in acetone. After dispersing CPN in the polymer solution, the polymer–CPN mixture was slowly injected into 25 ml of phosphate buffer (pH 7.4). The implant formed was collected and placed in 3 ml of phosphate buffer (pH 7.4). The buffer containing the implant was incubated in an orbital shaker at 37°C and 150 rpm. After each sampling interval, namely, 2, 6, 12, 24, 36, 48 and 72 h, the supernatant (3 ml) was collected and analyzed for curcumin using a UV spectrophotometer. The amount of curcumin in the supernatant was calculated by comparing the absorbance measured at 422 nm with the standard curve. A fresh amount (3 ml) of phosphate buffer (pH 7.4) was added to the implant and incubated once again on the shaker for the next sampling.
Results and discussion
Factorial design
Factorial design helps in identifying the variables, playing a significant role in determining the outcome. It helps to know the effect of interaction between the variables on the outcome. Based on the level of significance (p value < 0.05), percentage contribution, and the main effect, the process variables are selected and studied for interaction using response surface methodology. Factorial design allows rapid analysis of a large number of variables for identification of the important factor required to achieve the predetermined attribute.
In the present study, although the effect of the independent variables as such did not have a significant effect (p value > 0.05) on the mean particle size, speed had the maximum influence on the outcome. More than 45% of the outcome was under the direct influence of HS.
CPR, HS, and HT had a negative effect on the dependent variables, and the stabilizer had a positive effect on the dependent variable. This study on the main effect of the variables showed that mean particle size reduces as the level of CPR, HS, and HT are increased and when the level of stabilizer is decreased (, ).
The analysis of the effect of interaction between any two selected variables showed that mean particle size increases when the level of stabilizer is increased, irrespective of the level of CPR. However, the mean particle size decreases when HS and HT are maintained at a high level, irrespective of CPR. When the homogenization speed is maintained at a low level (4000 rpm), the change in the HT did not affect the mean particle size. However, when the speed was maintained at 12,000 rpm, the particle size decreased as the homogenization time increased. The mean particle size decreased when the homogenization speed was increased, irrespective of the level of the stabilizer. However, as the homogenization time was increased, the particle size decreased when the level of PVA was 0.5% w/v and increased when the level of PVA was 2% w/v ().
Figure 2. Contour plot. The effect of various levels of CPR and homogenization speed on mean particle size when the level of remaining variables are maintained either at (a) low level or (b) high level.
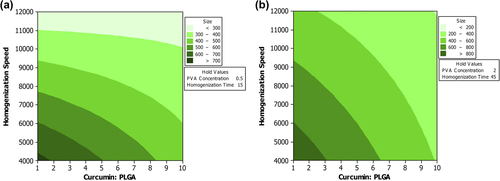
When the levels of CPR and stabilizer were low, the mean particle size was dependent on HS, and HT did not have any significant role. However, when CPR and stabilizer were high, a high level of HS and HT was required to reduce the mean particle size (). The viscosity of the system will increase as the level of stabilizer is increased. A high viscosity will reduce the effectiveness of the homogenization speed. Therefore, a longer HT would need to be achieved to reduce the particle size (CitationJelvehgari et al. 2011).
Figure 3. Overlaid contour plot showing the level of CPR and PVA concentration required to achieve mean particle size less than 400 nm when the homogenization speed and homogenization time were maintained at 12000 rpm and 45 min respectively.
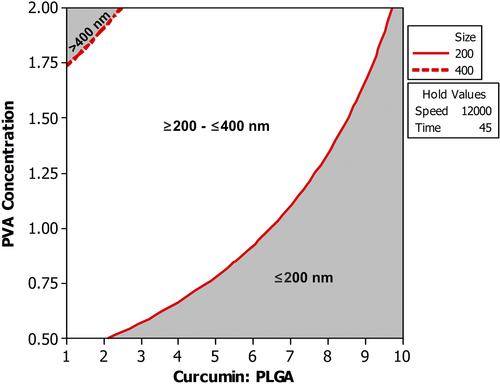
This study shows that although the individual factors have a particular effect on the outcome, it is their interaction with other factors that ultimately determines the outcome. Therefore, it is always better to understand the behavior of independent variables in the presence of varying levels of other variables. This could be only carried out if the experiments are analyzed using a multivariate technique. This useful information would be missed if the effect of process variables is investigated using the univariate approach (CitationYu 2008).
BBD
Optimization following a univariate approach cannot predict the effect of interaction on the outcome. Factorial design and response surface design represent the multivariate approach. In comparison to factorial design, response surface design is useful in interpreting interactions more effectively as the factors are varied at three levels. However, as mentioned earlier, factorial design is useful in reducing the number of variables so that it is practically possible to perform the experiment using response surface design. Among the various response surface methodologies available, BBD is widely used, as it is more efficient than the other models (CitationFerreira et al. 2007).
The study on the main effect of the individual variables showed the mean particle size decreases as the levels of HS, CPR, and sonication time are increased. The decrease in the size is most prominent with increasing level of sonication time. In case of homogenization speed, the decrease in size is most prominent when the speed is increased from 9000 to 10,500, after which a change in size is not significant. In case of D/P ratio, the decrease in the size is less prominent till the ratio reaches 5.5. However, once the ratio is increased to a level above 5.5, the decrease in the size becomes more prominent. The effect of individual variables on PDE shows that increasing the level of homogenization speed and sonication time increases the amount of drug encapsulated. In case of CPR, the PDE reaches a maximum at 5.5, after which there is a decrease in the amount of drug encapsulated ().
Figure 4. Main effect plot (a) Linear effect of the variables on mean particle size (b) Linear effect of the variables on PDE.
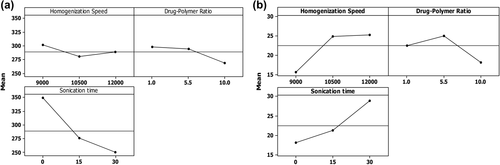
The study on interaction ( and ) showed that when the HS is maintained at a low level (9000 rpm), a small increase in the MPS (from 250 nm to 300 nm) is observed as the CPR is increased. Decrease in particle size with increase in CPR becomes prominent (from a size 350 nm to a size < 250 nm) when the speed is maintained at a high level, particularly at 12,000 rpm. However, the study on interaction between HS and CPR showed that PDE also reduces with increasing CPR, and the role of HS on PDE becomes less significant when the speed is above 10,500 rpm. Similarly, when the HS was above 10,500 rpm, change in the PDE was less prominent as the CPR increased from 1 to 5.5. However, there was a significant fall in PDE when the CPR was increased beyond 5.5. PDE was at least 10% lower when the HS was maintained at 9000 rpm in comparison to the PDE obtained, when the speed was maintained above 9000 rpm.
Figure 5. Interaction effect plot (a) Effect of interaction of the variables on mean particle size (b) Effect of interaction of the variables on PDE.
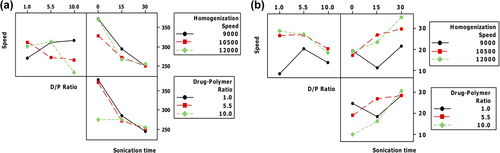
Figure 6. Overlaid contour plot showing the effect of various levels of homogenization speed and CPR on mean particle size and percentage drug encapsulation when the sonication time was maintained at 30 s.
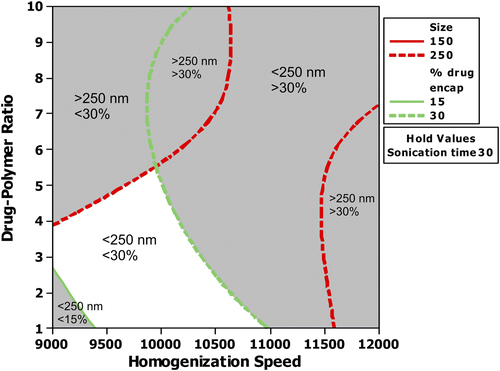
There was a significant reduction in the particle size as the sonication time increased, irrespective of the homogenization speed. However, the analysis of interaction of CPR with sonication time showed that the reduction in the mean particle size was prominent until the D/P ratio reached the middle level. The interaction (sonication time and CPR) did not produce any significant change in the particle size distribution when the CPR was 10. The mean particle size was already at the lowest possible size when the sonication time was zero. Therefore, sonication would not have affected the mean particle size, even when the sonication time was maintained at the selected maximum level. The interaction between CPR and sonication time showed that PDE is lowest when the level of CPR is 10 and sonication time is zero seconds. However, as the sonication time is increased, the effect of CPR on PDE becomes less significant. At 30 s, PDE is almost the same, irrespective of CPR. A CPR of 5.5 was found to be more suitable, as maximum possible PDE and low particle size (close to 250 nm) were achieved within 15 s of sonication time. Further studies using sonication time above 30 s could help in understanding whether PDE still increases. However, a sonication time above a certain level would not only affect the drug encapsulation, but could also cause degradation of drug. This may require new set of experiments analyzing the effect of sonication time above the level used in the present study.
Sonication produces shear force by inducing cavitation in the system (CitationEsmaeilzadeh-Gharedaghi et al. 2012). In many top-down approaches, sonication—either alone or in combination with other techniques—is used as the primary source of mechanical force to produce the shear force required for preparing an emulsion containing nanoparticles (CitationBilati et al. 2003, CitationLeleux and Williams 2013). In the present study, sonication was primarily used for breaking the aggregates formed after removal of the solvent from the initial emulsion. The effect of sonication on size was less pronounced at high CPR. Increase in the CPR increases the viscosity. This reduces the cavitation, resulting in lower efficiency of shear force, thereby reducing the influence of sonication (CitationEsmaeilzadeh-Gharedaghi et al. 2012). However, an optimum level is required to achieve stable particles with maximum encapsulation at a desired size. Therefore, conditions have to be chosen such that a balance in MPS and PDE could be achieved.
Curcumin-PLGA nanoparticles of size 360.33 ± 19.72 nm, zeta potential − 4.63 ± 0.53, PDI 0.36 ± 0.004 and PDE 26.51 ± 5.93% were obtained under optimized conditions (CPR 5.5, PVA 1% w/v, HS 12,000 rpm, HT 20 min and sonication time 15 s). These were used for further studies.
Drug release
Drug release studies showed that the amount of drug released immediately after reconstitution of lyophilized curcumin-PLGA with PBS was high, after which the release was in a sustained manner. The release of curcumin attached to the surface of the polymer would have resulted in the initial burst release. Curcumin released after the initial release could be either due to the diffusion of the drug entrapped within the polymer, or due to slow degradation of the polymer ().
In vivo studies
Administration of curcumin-PLGA nanoparticles was found to control the development and progression of EAC in Swiss albino mice. Tukey's multiple comparisons test showed that plain curcumin and curcumin-PLGA were able to significantly control EAC-induced increase in the body weight. At the end of the 14th day, the percentage increase in body weight in the EAC control, plain curcumin, and curcumin-PLGA groups was 38.04 ± 6.56, 18.75 ± 7.07, and 5.87 ± 3.10 () respectively, and tumor volume was 7.83 ± 1.00, 4.0 ± 0.5, and 3.2 ± 0.9 respectively (). Nanoparticles were able to increase the survival of EAC-bearing mice () to the same extent as that of the plain curcumin. The mean life span and percentage increase in mean life span in case of intervention were also high when compared to the EAC control ().
Figure 8. Change in body weight and tumor volume in various animal groups (a) Percentage increase in body weight of EAC-bearing animals receiving treatment (b) Tumor volume in EAC-bearing mice receiving treatment. ‘*’ shows that the difference in the tumor volume between the EAC control and the treatment group was significant.
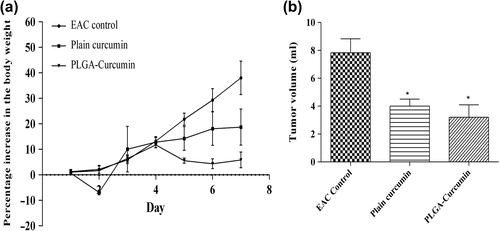
Table III. Mean life span and percentage increase in mean life span in EAC-bearing mice receiving treatment.
The analysis of effectiveness of the nanoparticles on hematological parameters showed that the nanoparticles were able to effectively control the rise in WBC level and the fall in the RBC and hemoglobin levels, which are normally observed during tumor development. This indicates the effectiveness of treatment ().
Figure 10. Effect of treatment on hematological parameters. ‘a’ shows that there was a significant increase in WBC level and decrease in Hb level in the EAC control compared to the normal. ‘b’ shows that the change in WBC level and Hb level is not significant when compared to the normal control. ‘c’ shows that treatment significantly reversed EAC-induced increase in WBC level and EAC-induced decrease in Hb level when compared to EAC control.
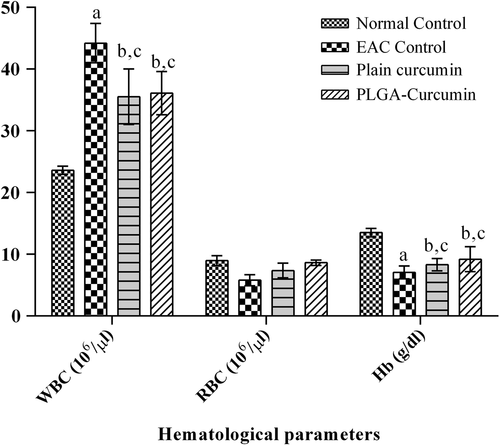
In vivo studies showed that CPNs were as effective as the standard plain drug, even when administered at half the dose of plain curcumin. Generally, the main objective of developing a nanoparticle-based delivery system is to achieve a better therapeutic efficacy than the original drug or the previously existing formulation (CitationJagani et al. 2015). In the present study, the therapeutic efficacy was analyzed using the EAC model. Although the EAC model allows rapid screening of the effectiveness of the formulation, the model may not be useful to assess the effectiveness of the formulation if the advantage of the formulation is mainly due to the improvement in the pharmacokinetic properties. For such a formulation, other animal models such as the solid tumor model would be required to understand the true benefit of the formulation. Still, the EAC model is useful to know if the formulation is effective in controlling tumor progression. The EAC model could be used as a primary study, before proceeding with robust studies.
Histopathology of vital organs, namely the liver, spleen, and kidney showed that although there were EAC-induced pathological changes in the major organs, there was no drug (curcumin and CPNs)-induced toxicity ().
PCL-based in situ injectable implant
The in situ implants are prepared as liquid formulations, which form a depot at the injected site. These are less invasive and can be easily administered compared to the implants that need to be surgically placed at the required site. These implants are useful in achieving drug release for a long period in a controlled manner (CitationPackhaeuser et al. 2004). In the present study, the in situ implant released curcumin in a sustained manner. The amount of drug released at 2 h was less compared to the amount of curcumin released from the CPNs (). This shows that the implant was able to control the initial burst release observed with CPN. Once the PCL-CPN is added into the aqueous (PBS) medium, PCL containing CPN will precipitate, resulting in entrapment of CPN within PCL. Curcumin would first release from CPN, and then diffuse through the porous mesh of PCL. Therefore, this will effectively reduce the amount of curcumin released in a given period. This kind of implant would be useful in treating conditions such as cancer for releasing the drug near the target site for an extended period to avoid relapse after chemotherapy. Further studies on this PCL-based CPN implant using suitable animal models would help to understand their efficacy.
Conclusion
The effect of process variables, namely curcumin/PLGA ratio, PVA concentration, homogenization time, and homogenization speed involved in preparation of CPNs by the emulsion–solvent evaporation technique was studied using the multivariate approach. The study showed that effect of interaction of the independent variables—particularly the interaction of sonication time with other independent variables—determined the outcome, namely mean particle size and percentage drug encapsulation. When curcumin/PLGA ratio, PVA concentration, homogenization time, homogenization speed, and sonication time were 5.5, 1% w/v, 20 min, 12,000 rpm, and 15 s respectively, CPNs of size 360.33 ± 19.72 nm, zeta potential − 4.63 ± 0.53, PDI 0.36 ± 0.004, and PDE 26.51 ± 5.93% were achieved. Curcumin was released in a sustained manner from the CPNs. These nanoparticles were able to control EAC-induced changes in Swiss Albino mice. PCL based in situ implant was able to reduce the burst release observed with CPN.
Acknowledgement
The authors would like to acknowledge the authorities of Manipal University for providing the necessary facilities required for carrying out this experiment.
Conflict of interest
All the authors included in the manuscript declare that there is no conflict of interest regarding the material discussed in the manuscript with any of the financial organizations.
References
- Anand P, Nair HB, Sung B, Kunnumakkara AB, Yadav VR, Tekmal RR, Aggarwal BB. 2009. Design of curcumin-loaded PLGA nanoparticles formulation with enhanced cellular uptake, and increased bioactivity in vitro and superior bioavailability in vivo. Biochem Pharmacol. 79:330–338.
- Bilati U, Allémann E, Doelker E. 2003. Sonication parameters for the preparation of biodegradable nanocapsules of controlled size by the double emulsion method. Pharm Dev Technol. 8:1–9.
- Chuah LH, Billa N, Roberts CJ, Burley JC, Manickam S. 2013. Curcumin-containing chitosan nanoparticles as a potential mucoadhesive delivery system to the colon. Pharm Dev Technol. 18:591–599.
- Esmaeilzadeh-Gharedaghi E, Faramarzi MA, Amini MA, Rouholamini Najafabadi A, Rezayat SM, Amani A. 2012. Effects of processing parameters on particle size of ultrasound prepared chitosan nanoparticles: An Artificial Neural Networks Study. Pharm Dev Technol. 17:638–647.
- Ferreira AP, Tobyn M. 2014. Multivariate analysis in the pharmaceutical industry: enabling process understanding and improvement in the PAT and QbD era. Pharm Dev Technol. 1–15.
- Ferreira SL, Bruns RE, Ferreira HS, Matos GD, David JM, Brandao GC, et al. 2007. Box-Behnken design: An alternative for the optimization of analytical methods. Anal Chim Acta. 597:179–186.
- Gao Y, Li Z, Sun M, Li H, Guo C, Cui J, et al. 2010. Preparation, characterization, pharmacokinetics, and tissue distribution of curcumin nanosuspension with TPGS as stabilizer. Drug Dev Ind Pharm. 36:1225–1234.
- Gosangari S, Dyakonov T. 2013. Enhanced dissolution performance of curcumin with the use of supersaturatable formulations. Pharm Dev Technol. 18:475–480.
- Gosangari SL, Watkin KL. 2012. Effect of preparation techniques on the properties of curcumin liposomes: Characterization of size, release and cytotoxicity on a squamous oral carcinoma cell line. Pharm Dev Technol. 17:103–109.
- Guideline ICHHT. 2009. Pharmaceutical development. Q8 (2R). As revised in August.
- Jagani H, Kasinathan N, Meka SR, Josyula VR. 2015. Antiapoptotic Bcl-2 protein as a potential target for cancer therapy: A mini review. Artif Cells Nanomed Biotechnol. 24:1–10.
- Jelvehgari M, Hassanzadeh D, Kiafar F, Loveym BD, Amiri S. 2011. Preparation and determination of drug-polymer interaction and in-vitro release of mefenamic acid microspheres made of celluloseacetate phthalate and/or ethylcellulose polymers. Iran Journal Pharm Res. 10:457–467.
- Kasinathan N, Amirthalingam M, Reddy ND, Jagani HV, Volety SM, Rao JV. 2014a. In-situ implant containing PCL-curcumin nanoparticles developed using design of experiments. Drug Deliv. 23:1–9.
- Kasinathan N, Jagani HV, Alex AT, Volety SM, Rao JV. 2014b. Strategies for drug delivery to the central nervous system by systemic route. Drug delivery. 1–15.
- Khalil NM, Nascimento TCFD, Casa DM, Dalmolin LF, Mattos ACD, Hoss I, et al. 2013. Pharmacokinetics of curcumin-loaded PLGA and PLGA–PEG blend nanoparticles after oral administration in rats. Colloids Surf B Biointerfaces. 101:353–360.
- Koganti S, Jagani HV, Palanimuthu VR, Mathew JA, Rao MC, Rao JV. 2013. In vitro and in vivo evaluation of the efficacy of nanoformulation of siRNA as an adjuvant to improve the anticancer potential of cisplatin. Exp Mol Pathol. 94:137–147.
- Yu LX. 2008. Pharmaceutical quality by design: product and process development, understanding, and control. Pharm Res. 25:781–791.
- Leleux J, Williams RO. 2013. Recent advancements in mechanical reduction methods: particulate systems. Drug Dev Ind Pharm. 1–12.
- Lionberger RA, Lee SL, Lee L, Raw A, Lawrence XY. 2008. Quality by design: concepts for ANDAs. AAPS J. 10:268–276.
- Narayanan K, Subrahmanyam VM, Rao JV. 2014. Application of experimental design in preparation of nanoliposomes containing hyaluronidase. J Drug Deliv. 2014:948650.
- Packhaeuser CB, Schnieders J, Oster CG, Kissel T. 2004. In situ forming parenteral drug delivery systems: an overview. Eur J Pharm Biopharm. 58:445–455.
- Pal S, Choudhuri T, Chattopadhyay S, Bhattacharya A, Datta GK, Das T, Sa G. 2001. Mechanisms of curcumin-induced apoptosis of Ehrlich's ascites carcinoma cells. Biochem Biophys Res Commun. 288:658–665.
- Piñón-Segundo E, Nava-Arzaluz MG, Lechuga-Ballesteros D. 2012. Pharmaceutical Polymeric Nanoparticles Prepared by the Double Emulsion-Solvent Evaporation Technique. Recent Pat Drug Deliv Formul. 6:224–235.
- Ranjan AP, Mukerjee A, Helson L, Gupta R, Vishwanatha JK. 2013. Efficacy of liposomal curcumin in a human pancreatic tumor xenograft model: inhibition of tumor growth and angiogenesis. Anticancer Research. 33:3603–3609.
- Ranjan AP, Mukerjee A, Helson L, Vishwanatha JK. 2012. Scale up, optimization and stability analysis of Curcumin C3 complex-loaded nanoparticles for cancer therapy. J Nanobiotechnology. 10:38.
- Raveendran R, Bhuvaneshwar GS, Sharma CP. 2013. In vitro cytotoxicity and cellular uptake of curcumin-loaded Pluronic/Polycaprolactone micelles in colorectal adenocarcinoma cells. J Biomater Appl. 27:811–827.
- Tabatabaei Mirakabad FS, Akbarzadeh A, Milani M, Zarghami N, Taheri-Anganeh M, Zeighamian V, et al. 2014. A Comparison between the cytotoxic effects of pure curcumin and curcumin-loaded PLGA-PEG nanoparticles on the MCF-7 human breast cancer cell line. Artif Cells Nanomed Biotechnol. 17:1–8.
- Yallapu MM, Jaggi M, Chauhan SC. 2012. Curcumin nanoformulations: a future nanomedicine for cancer. Drug Discov Today. 17:71–80.