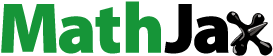
Abstract
Gene silencing by siRNA (short interfering RNA)-targeted human telomerase reverse transcriptase (hTERT) is considered a successful strategy for cancer gene therapy. Polyelectrolyte complexes (PEC) of siRNA and cationic polymers such as polyethyleneimine (PEI) have been widely used for cellular transfection; however, they demonstrate some disadvantages such as cytotoxicity and extracellular matrix restrictions. PEG grafting technology was used in an attempt to improve the biocompatibility of PECs. Considering that this technology may compromise the cellular uptake of PECs, we aimed to study the effect of degree of PEI PEGylation on the carrier cytotoxicity, cellular association, and transfection efficiency of hTERT siRNA in the lung cancer cell line A549.
Activated NHS ester of methoxy PEG–COOH 5 KDa was grafted to hyperbranched PEI 25 KDa in the molar ratios of 0.2 and 1. The copolymers were characterized by 1H-NMR spectroscopy. PECs of PEI or PEG-g-PEI with siRNA, alone or co-incubated with heparin sulfate, were studied by the ethidium bromide exclusion assay. Cytotoxicity of the polymers (PEG-g-PEI vs PEI), alone and upon formation of PEC nanoparticles with hTERT siRNA, was determined by a validated MTT assay, in comparison to a scrambled control sequence, in A549 human lung carcinoma cells. The cellular uptake of the PECs of FITC-labeled siRNA was investigated by flow cytometry at different N/P ratios, and the silencing effect of the transfected siRNA was compared to that of the control sequence for different PECs by real time RT-PCR.
The cytotoxicity of PEI decreased significantly by PEG grafting, even at a low degree of PEGylation. Moreover, the nonspecific cytotoxicity of PECs decreased by PEG grafting. PECs of PEG-g-PEI showed more biologic stability on incubation with heparin sulfate. Average particle size and zeta potential of PEC nanoparticles were diminished for those of PEG-g-PEI. The cellular association was more pronounced at an N/P ratio of 2.5 for PECs of PEI and PEG-g-PEI alike. The level of silencing of hTERT mRNA by PEC of PEG-g-PEI was sequence-dependent, and determined non-inferior when compared to the native PEI.
Conclusively, the biocompatibility of PEI was improved by a low degree of PEGylation, with no adverse effect on the cellular uptake and the transfection activity. PEC nanoparticles of hTERT siRNA and PEG-g-PEI could act as a promising weapon against A549 cells, which has to be considered for an in vivo evaluation.
Introduction
Telomerase is a ribonucleoprotein complex that is capable of preserving the length of the telomere at a stable status in cancer cells. Unlike in the cancer cells, in which telomerase is highly active, expression of telomerase in most normal somatic cells cannot be detected (CitationMorin 1989, CitationBlackburn 1994, CitationRahmati-Yamchi et al. 2011). This potential can therefore be exploited to make telomerase a target molecule for cancer therapy in humans (CitationShay et al. 2001, CitationAkbarzadeh et al. 2012). Human telomerase reverse transcriptase (hTERT) is a catalytic subunit and an essential component of human telomerase (CitationMeyerson et al. 1997). Research on various human cancer cells, such as liver and breast cancer cells, has indicated the correlation of hTERT mRNA expression and telomerase activity (CitationHisatomi et al. 2003, CitationKirkpatrick et al. 2003, CitationZhang et al. 2006).
RNAi technology is regarded as a promising therapeutic approach to cure various diseases such as cancers, viral infections, and inflammation by means of site-specific silencing of targeted genes (CitationZhou et al. 2013). This technology, known as the post-transcriptional gene regulation technique (CitationZamore et al. 2000), has been demonstrated in several eukaryotic organisms such as human cell lines (CitationElbashir et al. 2001, CitationBridge et al. 2003). Small interfering RNA (siRNA) molecules, double-stranded with 21 to 24 nucleotides and single-stranded with two nucleotide 3’-overhang, degrade the complementary mRNA with high specificity in a short period of time (CitationTuschl et al. 1999, CitationAraujo et al. 2006). Designing effective siRNA requires a combination of computer algorithms and empirical testing. Also, to avoid an off-target effect, the siRNA sequence designed should have less than 84% homology with the other sites of the genome and more than that with the target mRNA. Other parameters that affect the level of siRNA functionality include: potential for intramolecular secondary structures, the stability of the ends of the duplex strands, and specific sequence motifs within the duplex (CitationReynolds et al. 2004, CitationTakasaki et al. 2004, CitationHeale et al. 2005).
Despite the siRNA encouraging biomedical therapeutic approaches, gene silencing via siRNA faces many barriers because of the lack of stability in vivo and poor permeability of the cell membranes, due to their highly hydrophilic and anionic characteristics (CitationBumcrot et al. 2006, CitationMoreira et al. 2008).
Thus, the development of appropriate delivery systems for nucleic acid-based treatments via RNAi is crucial. Viral vectors consist of lentiviruses (CitationGrimm and Kay 2007) or adeno-associated viruses (CitationYamagishi et al. 2009), which are the most efficient carriers of target-cell transfection with gene therapy. However, the in vivo drawbacks of these vectors include toxicity, immunogenicity, and unforeseen secondary effects caused by attenuated viruses; these challenges have remained unresolved (CitationWoods et al. 2006).
Other siRNA carriers such as cationic polymers and block co-polymers have been been used, owing to their good stability, easily modified chemical structures, and simple preparation (CitationSamal et al. 2012). In the past decade, several polymer-based siRNA delivery systems composed of polyethylenimine (PEI), chitosan, cyclodextrin, and so on have been considered.
PEI is a branched or linear cationic polymer, with low immunogenic stimulation and safety issues, that is used widely in siRNA delivery (CitationSaengkrit et al. 2012). PEI participates in non-covalent reactions with nucleic acid molecules, mediated by a protonable amino group in every third position and high cationic charge density at physiological pH. Endosomal release of siRNA into the cytosol is facilitated by “a proton sponge” effect. Also, secondary and tertiary amines represent pKa buffering capacity between physiological and lysosomal pH (CitationOskuee et al. 2010). The high transfection efficacy is directly correlated to molecular weight, which makes branched PEI (25 kDa) a “gold standard” for non-viral vectors (CitationXu and Yang 2011). However, the major challenge of PEI application is inducing cell cytotoxicity, which depends on the molecular weight and concentration of the polymer (CitationChoosakoonkriang et al. 2003). Therefore, to improve biocompatibility and transfection efficiency (CitationMao et al. 2006), enhance stability (CitationKichler et al. 2002), and decrease the cytotoxicity, the PEI core is grafted to hydrophilic poly(ethylene glycol).
Evidence of previous studies in which siRNA transfection achieved by these copolymers has shown low cytotoxicity (CitationPetersen et al. 2002) and has been found appropriate for in vivo siRNA delivery (CitationMerkel et al. 2009) depending on the degree of PEG grafting and the ratio between PEI amine groups and the phosphate of nucleic acids (N/P ratio) (CitationMerkel et al. 2009).
In this study, PEG-g-PEI/siRNA polyplexes were administered to the lung adenocarcinoma cell line in vitro to analyze cytotoxicity, cellular uptake of siRNA, efficacy of gene knock-down, and most importantly, determination of the most suitable degree of PEG grafting and N/P ratio in copolymers. The promising results, as described below, showed an ability of the designed siRNA in copolymers to reduce hTERT expression in A549 cell line of lung cancer while maintaining minimal cell cytotoxicity.
Materials and methods
Materials and reagents
Poly(ethyleneglycol)-g-polyethylenimine (PEG; 5 kDa and PEI; 25 kDa) was synthesized by the pharmaceutics department of the Pharmacy School, University of Medical Sciences (Shiraz, Iran). (3-(4,5-dimethyl-thiazol-2-yl)- 2,5-diphenyl tetrazolium bromide (MTT) was purchased from Sigma-Aldrich (St Louis, MO, USA). The real-time PCR kit was purchased from TaKaRa Biotechnology, (Dalian, China). Cell culture medium and fetal bovine serum (FBS) were purchased from GIBCO (Carlsbad, CA, USA). The human lung adenocarcinoma cell line, A549, was obtained from Pasteur Institute (Tehran, Iran). siRNA designed against human hTERT, scramble siRNA, and positive control siRNA sequences were synthesized by Qiagen (Korea). The following siRNA targeting sequences were used: siRNA-hTRET, 5′ GCACUUCCUCUACUCCUCAtt 3′; negative control, 5′ UGAUUUCUUGUUGGUGACATT 3′; positive control, 5′ UUCACGCCUCUACUCCUCAtt 3′.
Cell culture
Human lung adenocarcinoma A549 cells were cultured and maintained in RPMI 1641 medium containing 10% FBS and antibiotics at 37°C in a humidified atmosphere of 5% CO2.
Preparation of PEG–PEI copolymers
Hyperbranched polyethyleneimine (PEI, 25 kDa) was the starting material for the synthesis of the PEG-grafted-PEI block copolymer. NHS-functionalized PEG (mPEG-NHS, 2 kDa) was grafted to the amine group of PEI by mixing mPEG-NHS with 1% PEI, at molar ratios of 3:1 and 10:1, in a 50 mM phosphate buffer of pH 9, and the mixture was incubated at room temperature overnight for 1 h while shaking at 400 rpm. The residual free mPEG was removed by precipitating PEG-g-PEI block copolymers in the presence of acetone and cold centrifuging at 3000 g for 30 min. The product was washed once with a mixture of deionized water and acetone (1:3) and then redispersed in deionized water. The product was characterized with the gel filtration chromatography using the Knauer HPLC system equipped with a refractive index (RI) detector. An aqueous solution of 10 mM EDTA was pumped as the mobile phase at a flow rate of 0.5 ml.min− 1. The chromatogram was compared with that of native PEI and free mPEG-NHS to study the course of the PEGylation reaction and the purity of the product (i.e., absence of unreacted PEG).
1H-NMR
H1-NMR was performed as described (CitationPetersen et al. 2002), to calculate the degree of PEG grafting and average molecular weight of the copolymer by the proton integration method, according to the following equations. The molar ratio of PEG:PEI was calculated with the following equation:
In which δ(3.5–3.6) and δ(2.5–3.1) refer to –O–CH2– units of PEG and –N–CH2–CH2–units of PEI molecules respectively.
Preparation of the polyelectrolyte complex of siRNA
PEI and PEG-g-PEI copolymers were dissolved in different concentrations according to various N/P ratios (1 μg of DNA or siRNA contains 3 nmol of phosphate, and 1 μl of 0.9 mg/ml PEI solution contains 20.9 nmol of amine nitrogen). Appropriate volumes of siRNA (20 μM) and PEG-g-PEI solutions were added to deionized water, and then gently mixed and incubated for 20 min at room temperature to form nanoparticles (CitationWeber et al. 2012).
Zeta potential and size measurements
Dynamic light scattering (DLS) was used to monitor the formation of the nanoscale complexes, as previously described (CitationMerkel et al. 2009). To prepare the sample, PEG-g-PEI/siRNA complexes were formed according to various N/P ratios, and then diluted in 1.5 mL of deionized water (CitationLiang et al. 2012). The size of nanoparticles was measured by ZetaPlus (Brookhaven, NY, USA) at 25°C. Zeta potentials of the nanoparticles were analyzed by means of the same instrument at a 45° angle and 25°C. The data represent the average ± standard deviations.
Ethidium bromide dye exclusion assay
Reaction between nucleic acid and ethidium bromide causes increased fluorescence, due to the interchelating characteristics of ethidium bromide. The formation of the copolymer consisting siRNA and PEC may decrease the fluorescence in response to reduced interchelating property. Therefore, 50 μlit of siRNA (20 μmolar) was added to the positive control well, and polyplexes with different degrees of PEG grafting and N/P ratios were added to other wells. Ethidium bromide (10 μlit, 1 μgr/1 ml) was directly added to the wells. The wells without siRNA and polylplex played the role of negative control. A fluorometer was used to measure the fluorescence at the excitation and emission wavelengths of 510 and 590 nm.
Polyanion competition assay
The relative stability of PECs of siRNA was evaluated by measuring siRNA release from nanoparticles in the presence of a competing heparin sulfate. Copolymers were prepared as described above, at different degrees of PEG grafting and different N/P ratios, then treated with different amounts of heparin from 0.1–2 International Units, over 15 min. Afterwards, 20 μlit of ethidium bromide was added to each well. Finally, the fluorescence at the excitation and emission wavelengths of 510 and 590 nm was determined by the fluorometer. Negative controls were included as wells without siRNA and polylplex.
Cytotoxicity assay in vitro
A549 cells were seeded in 96-well plates at an initial density of 30000 cells/well in 100 μl of medium, and incubated for 24 h to pass the lag phase; after this time, the subculture was ready for the addition of the polymers. The cytotoxicity of PEI and PEG-g-PEI were evaluated at different final concentrations (1–100 μgr/ml), and each concentration was tested five times. In addition, the cytotoxicity of PEG-g-PEI/siRNA at different degrees of PEG grafting and N/P ratios was also investigated; PEI was used as a control. siRNA at a concentration of 10 pM per well was used to constitute the PEG-g-PEI/siRNA complexes and cellular transfection (CitationMerkel et al. 2009). After 4–24 h of incubation, the medium was replaced with 90 μl of serum-free medium, and 10 μl of MTT solution (5 mg/mL) per well. After 4 h of additional incubation, solution containing MTT was substituted by 100 μL of DMSO per well. The plate was gently shaken and incubated for 10 min. An ELISA reader (BioTek, USA) was used to assess absorbance of the wells at 590 nm and 630 nm wavelengths. Separately, viability of cells that encountered PEC of siRNA at both degrees of PEG grafting and different N/P ratios after 4 h of treatment and 24 h of additional incubation was evaluated. Finally, the percentage of viable cells over untreated control ± standard deviation were calculated.
Quantitative real-time PCR
After transfection, 4 h of incubation with polyplexes and additional incubation for 48 h was carried out by extracting total RNA using TriPure isolation reagent (Invitrogen, Carlsbad, CA, USA) according to the manufacturer's instructions. The sequences of primers were as follows: hTERT (forward primer: 5′CCGCCTGAGCTGTACTTTGT30and reverse primer: 5′CAGGTGAGCCACGAACTGT3′); Bactin (forward primer: 5′TCCCTGGAGAAGAGCTACG3′0 and reverse primer: 5′GTAGTTTCGTGGATGCCACA3′) [3]. Reverse transcription and real-time PCR were performed based on the protocol of SYBR Green-I RT-PCR Kit (Roche, Germany). The reaction mixture contained: 2 ul of cDNA, 0.4 uL of forward primer (10 lM), 0.4uL of reverse primer (10 lM), 6uL of SYBR Premix, and 10.8 lL of RNase-free water. The thermal cycling process was then accomplished by means of the Rotor-GeneTM 6000 system (Corbett Research, Australia). The two-step cycling program was set as follows: initial denaturation at 95°C for 10 min, followed by 40 cycles of denaturation at 95°C for 15 s, annealing at 60°C for 30 s, and extension at 72°C for 30 s. At the end of each reaction, the melting curve analysis was carried out. The comparative Ct method (2−ΔΔCt method) was applied to acquire the range of hTERT mRNA level normalized to that of the housekeeping gene B-Actin, and relative expression of mRNA was assessed.
Statistical analysis
Data are expressed as mean ± standard error (SE). All statistical analyses were performed in SPSS 11.0 software. Analysis of variance (ANOVA) was used, and a P-value ≤ 0.05 was considered as significant.
Results
1H-NMR
The results of 1H-NMR showed that proton shift causes the polymer to construct with molar ratios of 0.2:1 and 1:1 (PEG:PEI), according to the molecular weight of 26 kDa, for PEG-g-PEI molecules ( and ).
Size and zeta potential of PEG-g-PEI/siRNA Complexes
The size and zeta potential of PEG-g-PEI/siRNA complexes varied at different degrees of PEG grafting and at an N/P ratio of 2.5. The results are shown in . The size of the PEI/siRNA nanoparticle was 279 ± 0.632 nm. The size reached 244 ± 0.342 nm at a PEG grafting degree of 0.2, and then decreased gradually to 114 ± 0.645 nm at a PEG grafting degree of 1. The zeta potential was negative at PEG grafting degree of 1 and positive at PEG grafting degree of 0.2, increasing linearly as a degree of PEG grafting decreased.
Table I. Particle size and zeta potential of polyplexes, determined by DLS. Values represented as mean ± S.D.
Ethidium bromide dye exclusion and polyanion competition assays
Analyzing the PEG-g-PEI/siRNA formation, fluorescence intensity was measured by a fluorometer at different degrees of PEG grafting and N/P ratios. The results in show a decrease in fluorescence with an increase in N/P ratio. To further characterize PEG-g-PEI/siRNA complexes, we next assessed the complexation efficacies of PEI-g-PEG at various N/P ratios. reveals that an enhanced degree of PEG grafting causes a significant decrease in the property of heparin release.
In vitro transfection assay
The transfection efficiencies of the complexes were evaluated using flow cytometry in A549 cells. FITC-labeled siRNA was used to form the complexes, PEI (25 kDa) was used as positive control. Cellular uptake seemed to be different at various N/P ratios; increasing in N/P ratios caused the transfection efficiencies to increase. Results showed that at the N/P ratio of 1.2, the fluorescence intensity was weak, but by increasing the ratio to 2.5, the intensity also increased. Experiments were repeated in two independent groups by means of flow cytometry. As shown in , the transfection efficiency of PEG-g-PEI/siRNA at N/P 2.5 was 95.5% ± 2.4% respectively.
Cytotoxicity assay in vitro
After 4 and 24 hours of incubation, the MTT assay was used to check the viability of cells that encountered PEG-g-PEI and PEI polymers at different concentrations ( and ). Viability values of cells without treatment, which were only incubated with free media, were used as control and set at 100%. At the same concentrations, PEI cytotoxicity was more than that of PEG-g-PEI (P ≥ 0.05). The increase in concentrations up to 10 μg/mL caused toxicity of both PEG-g-PEI and PEI to increase. As shown in , the cell viability in the presence of PEG-g-PEI/siRNA complexes was increased by the increasing degree of PEG grafting, but decreased by the increase in N/P ratio.
Figure 5. (a) Cytotoxicity of polyelectrolyte complexes alone at different degrees of PEG grafting and various concentrations on A549 cell line after 4 h of incubation. (b) Cytotoxicity of polyelectrolyte complexes alone at different degrees of PEG grafting and various concentrations on the A549 cell line after 24 h of incubation.
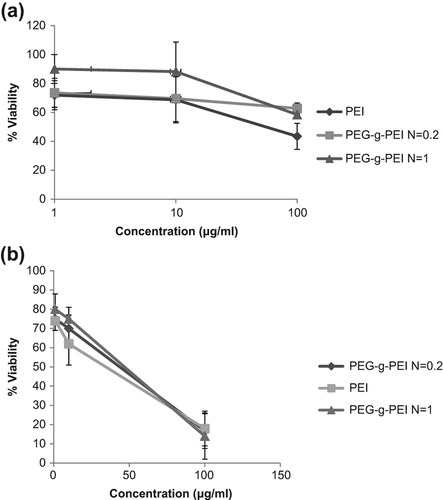
The gene-silencing effect
Real-time PCR analysis was used to evaluate the potency of the siRNA sequence designed to reduce hTERT mRNA levels respectively. The PEG grafting degree ratio of 2.5 was chosen for transfection of the A549 cells. As shown in , a reduction in expression of hTERT in both designed and positive control siRNA sequences was achieved by targeting the complementary hTERT mRNA, although the reduction caused by the designed sequence was greater than that of positive control. No obvious changes in hTERT expression of negative control siRNA confirmed the results due to other sequences. The effect of degree of PEG grafting on gene expression was evaluated, and the results showed that it had no negative effect on the reduction of gene expression.
Discussion
Improving our knowledge in the area of proteomics and genomics has enabled better understanding of the molecular pathways important for carcinogenesis, metastasis, and even treatment approaches. One of the therapeutic approaches in RNAi technology is that of targeting potential genes involved in tumorigenesis. Preferably, for specific silencing, selected targets should be exclusive and over-expressed by cancer cells (CitationBeyerle et al. 2010). Tumor gene expression profile identified some of these targets as intrinsically hTERT. To overcome impediments that inhibit siRNA from accessing the desired target mRNA disintegration, a non-viral carrier PEG-g-PEI was applied in the transfection of lung adenocarcinoma cell line with siRNA.
Our study demonstrated that this carrier ideally compacts siRNA into condensed and stable nanoparticles that may be successful transfectants. In addition, PEG-g-PEI copolymers could prevent siRNA degradation in the medium and enhance its ability to penetrate the cell membranes. Furthermore, these polyplexes, at an appropriate degree of PEG grafting and N/P ratio, could create high efficiency in cell transfection without challenging the cell viability. Finally, capability of these vectors to release siRNA into the cytoplasm to modify the expression of hTERT at the post transcriptional stage was shown.
Through this research, we have demonstrated that bridging the effect of the phosphate anion in the medium may induce aggregation of PEI into small particles and form large PEI particles (CitationTuschl et al. 1999). In contrast, expulsion of the steric band caused by flexible and electroneutral PEG chain formed small particles of PEG-g-PEI copolymers without aggregation (CitationMao et al. 2006). At the N/P ratio of 7.5, copolymers with lower degree of PEG grafting (0.2) formed complexes with the size of 244 nm. At higher degree of grafting (1), the size of the complex decreased to 114 nm. This implies that at higher degrees of PEG grafting, expulsion of the steric group prohibits the aggregation (CitationMerkel et al. 2009). Zeta potential was used to modulate surface charge of the complexes.. As shown in , at the N/P ratio of 2.5, the zeta potential of PEI/siRNA complexes was 11.8 ± 5.38 mV, whereas the zeta potential of PEG-g-PEI/siRNA remarkably decreased. Covering of the positive charge of the PEI/siRNA complexes by the hydrophilic PEG chains was a reason for this. The copolymers with lower degree of PEG grafting (0.2) formed complexes with the zeta potential reducing to 8.6 ± 6.55 mV. In contrast, at higher degree of PEG grafting (1), zeta potential reduces to − 11.8 ± 5.2 mV, because of the charge-covering effect of the higher degree of PEG grafting.
To assess copolymer construction, the ethidium bromide dye exclusion assay was performed. The results revealed that constitution of copolymer was formed at N/P ratio of 0.2, causing a decrease in fluorescence, which indicated that modification of siRNA by the PEG-g-PEI complex may inhibit the intercalating property of ethidium bromide in the siRNA backbone, leading to reduced fluorescence with increasing N/P ratio. Likewise, evidence from the results of the heparin competition assay confirmed that PEG grafting improves the stability of the PECs of siRNA, but increasing N/P ratio had no impressive effect on resistance of copolymer against the polyanionic characteristic of heparin.
The MTT assay was carried out to investigate the impact of PEGylation on polymer cytotoxicity. As shown in , at a low polymer concentration of lμg/ml, there was no significant difference between PEI and PEG-g-PEI, and cytotoxicity was also low (P ≥ 0.05). As the polymer concentration increased, the cytotoxicity gradually increased. For instance, at a polymer concentration of 10 μg/ml, the cell viability of PEG-g-PEI at a PEG grafting degree of 1 was more than a ratio of 0.2 and much more than that of PEI (CitationBeyerle et al. 2010); however increasing the concentration to 100 μg/ml caused cytotoxicity of both PEI and PEG-grafted PEI to increase. These results showed that at higher concentrations of polymer, the PEG grafting technique has no subtractive effect on cytotoxicity of polymer. In independent experiments, the cytotoxicity of polymer/siRNA complexes was determined. The results confirmed that constitution of polymer/siRNA caused cytotoxicity to reduce and cell viability was decreased by increasing N/P ratio. The evidence revealed that the effect of PEG-g-PEI on cell survival rate is much lower than that of PEI alone. Consequently, PEG-g-PEI would be utilized as a reagent of low toxicity for cell membrane transfection via siRNA.
Transfection efficiency is related to the stability of the polyplexes and their cell-penetration ability (CitationGrayson et al. 2006). The results of the assay for transfection efficiency revealed that with increase in N/P ratio, the transfection efficiency gradually increased. We found that cellular uptake may be facilitated by exacerbation of excessive positive charge in the complexes, which interacts with the negatively charged cell surface. At an N/P ratio of 2.5, maximal transfection efficiency was acquired. Other groups also confirmed these results (CitationBeyerle et al. 2010). The effect of the degree of PEG grafting on gene delivery was considered to be that the transfection efficiency of PEG-g–PEI/siRNA complexes was increased at a ratio of 0.2 but reduced with increase in degree of PEG grafting to a ratio of up to 1, maybe due to the charge-shielding effect of PEG that decreases interaction of polymer with negative charge of membrane. Although PEG grafting decreases the cytotoxicity of cationic polymers, and the PEG-g-PEI copolymers with less cytotoxicity can be used at higher N/P ratios, increasing in N/P ratio caused increased cytotoxicity. Thus, for selection of the ratio, attention should be paid to achieve a balance between cytotoxicity and transfection efficiency. On the other hand, increased degree of PEG grafting leads to increased transfection efficiency but with decreased viability of cells as a unfavorable effect, and an equilibrium should be optimized between a higher degree of PEG grafting for reducing cytotoxicity and a lower degree of PEG grafting for sufficient cellular interaction with the complexes (CitationBeyerle et al. 2010).
The results of real-time PCR showed that the PEG-g-PEI complex could release loading siRNA into cytoplasm and lead to a significant fall in hTERT expression, which indicates that PEG-g-PEI is a potent gene delivery vector. Data of biological effect of polyplexes revealed that the decline in gene expression due to the designed sequence, in comparison with that of the positive control sequence was significant. Based on classification by Ui-Tei et al. (CitationUi-Tei et al. 2004), highly ineffective siRNAs have activity lower than 20%, and those with more than 70% silencing effect are regarded as highly effective. Since the expression of hTERT in transfected cells with siRNA sequence designed with a PEG grafting degree of 0.2 and N/P ratio of 2.5 is 12.8, this sequence is classified as a highly active sequence. It implies that taking the specific rules into consideration for designing a highly effective siRNA is necessary (CitationReynolds et al. 2004, CitationTakasaki et al. 2004, CitationHeale et al. 2005). On the other hand, in comparison with PEI (25 kDa), PEG-grafted PEI has no negative effect on gene silencing; for instance, with the PEG grafting degree of 0.2 in copolymer containing designed sequence, maximal reduction in gene expression was achieved.
Conclusion
In this work, we proved that PEG-g-PEI is an appealing alternative as gene transfer system in the acquisition targeting tumor cells. The nanoscale copolymers constituted by PEG-g-PEI and siRNA were able to inhibit the hTERT expression, with low toxicity and high efficacy. However, confirmation of the claim that the PEG-g-PEI nanoparticle complex might be the ideal gene therapy agent is required, through testing the biological functions of PEG-g-PEI/siRNA polyplexes in vivo, to investigate their long-term application and to determine the most suitable dose for mediating gene expression in various cancer cell lines.
Acknowledgement
We acknowledge the Center for Pharmaceutical Nanotechnology and Biomaterials, Shiraz University of Medical Sciences, which, in addition to scientific and technical collaborations, supported us as a financial source.
Declaration of interest
The authors report no declarations of interest. The authors alone are responsible for the content and writing of the paper.
References
- Akbarzadeh A, Mikaeili H, Zarghami N, Mohammad R, Barkhordari A, Davaran S. 2012. Preparation and in vitro evaluation of doxorubicin-loaded Fe3O4 magnetic nanoparticles modified with biocompatible copolymers. Int J Nanomedicine. 7:511–536.
- Araujo R, Santos A, Pinto F, Gontijo N, Lehane M, Pereira M. 2006. RNA interference of the salivary gland nitrophorin 2 in the triatomine bug Rhodnius prolixus (Hemiptera: Reduviidae) by dsRNA ingestion or injection. Insect Biochem Mol Biol. 36:683–693.
- Beyerle A, Merkel O, Stoeger T, Kissel T. 2010. PEGylation affects cytotoxicity and cell-compatibility of poly (ethylene imine) for lung application: structure–function relationships. Toxicol Appl Pharmacol. 242:146–154.
- Blackburn EH. 1994. Telomeres: no end in sight. Cell. 77:621–623.
- Bridge AJ, Pebernard S, Ducraux A, Nicoulaz AL, Iggo R. 2003. Induction of an interferon response by RNAi vectors in mammalian cells. Nat Genet. 34:263–264.
- Bumcrot D, Manoharan M, Koteliansky V, Sah DW. 2006. RNAi therapeutics: a potential new class of pharmaceutical drugs. Nat Chem Biol. 2:711–719.
- Choosakoonkriang S, Lobo BA, Koe GS, Koe JG, Middaugh CR. 2003. Biophysical characterization of PEI/DNA complexes. J Pharm Sci. 92:1710–1722.
- Elbashir SM, Harborth J, Lendeckel W, Yalcin A, Weber K, Tuschl T. 2001. Duplexes of 21-nucleotide RNAs mediate RNA interference in cultured mammalian cells. Nature. 411:494–498.
- Grayson ACR, Doody AM, Putnam D. 2006. Biophysical and structural characterization of polyethylenimine-mediated siRNA delivery in vitro. Pharm Res. 23:1868–1876.
- Grimm D, Kay MA. 2007. Therapeutic application of RNAi: is mRNA targeting finally ready for prime time? J Clin Invest. 117:3633–3641.
- Heale BS, Soifer HS, Bowers C, Rossi JJ. 2005. siRNA target site secondary structure predictions using local stable substructures. Nucleic Acids Res. 33:e30.
- Hisatomi H, Ohyashiki K, Ohyashiki JH, Nagao K, Kanamaru T, Hirata H, et al. 2003. Expression profile of a γ-deletion variant of the human telomerase reverse transcriptase gene. Neoplasia. 5:193–197.
- Kichler A, Chillon M, Leborgne C, Danos O, Frisch B. 2002. Intranasal gene delivery with a polyethylenimine–PEG conjugate. J Control Release. 81:379–388.
- Kirkpatrick K, Clark G, Ghilchick M, Newbold R, Mokbel K. 2003. hTERT mRNA expression correlates with telomerase activity in human breast cancer. Eur J Surg Oncol. 29:321–326.
- Liang Y, Liu Z, Shuai X, Wang W, Liu J, Bi W, et al. 2012. Delivery of cationic polymer-siRNA nanoparticles for gene therapies in neural regeneration. Biochem Biophys Res Commun. 421:690–695.
- Mao S, Neu M, Germershaus O, Merkel O, Sitterberg J, Bakowsky U, Kissel T. 2006. Influence of polyethylene glycol chain length on the physicochemical and biological properties of poly (ethylene imine)-graft-poly (ethylene glycol) block copolymer/SiRNA polyplexes. Bioconjugate Chem. 17:1209–1218.
- Merkel OM, Librizzi D, Pfestroff A, Schurrat T, Buyens K, Sanders NN, et al. 2009. Stability of siRNA polyplexes from poly (ethylenimine) and poly (ethylenimine)-g-poly (ethylene glycol) under in vivo conditions: effects on pharmacokinetics and biodistribution measured by Fluorescence Fluctuation Spectroscopy and Single Photon Emission Computed Tomography (SPECT) imaging. J Control Release. 138:148–159.
- Meyerson M, Counter CM, Eaton EN, Ellisen LW, Steiner P, Caddle SD, et al. 1997. hEST2, the putative human telomerase catalytic subunit gene, is up-regulated in tumor cells and during immortalization. cell. 90:785–795.
- Moreira J, Santos A, Moura V, Pedroso de Lima M, Simoes S. 2008. Non-viral lipid-based nanoparticles for targeted cancer systemic gene silencing. J Nanosci Nanotechnol. 8:2187–2204.
- Morin GB. 1989. The human telomere terminal transferase enzyme is a ribonucleoprotein that synthesizes TTAGGG repeats. Cell. 59: 521–529.
- Oskuee RK, Philipp A, Dehshahri A, Wagner E, Ramezani M. 2010. The impact of carboxyalkylation of branched polyethylenimine on effectiveness in small interfering RNA delivery. J Gene Med. 12:729–738.
- Petersen H, Fechner PM, Martin AL, Kunath K, Stolnik S, Roberts CJ, et al. 2002. Polyethylenimine-graft-poly (ethylene glycol) copolymers: influence of copolymer block structure on DNA complexation and biological activities as gene delivery system. Bioconjug Chem. 13:845–854.
- Rahmati-Yamchi M, Zarghami N, Rahbani M, Montazeri A. 2011. Plasma leptin, hTERT gene expression, and anthropometric measures in obese and non-obese women with breast cancer. Breast cancer: Basic and Clinical Research. 5:27–35.
- Reynolds A, Leake D, Boese Q, Scaringe S, Marshall WS, Khvorova A. 2004. Rational siRNA design for RNA interference. Nat Biotechnol. 22:326–330.
- Saengkrit N, Sanitrum P, Woramongkolchai N, Saesoo S, Pimpha N, Chaleawlert-umpon S, et al. 2012. The PEI-introduced CS shell/PMMA core nanoparticle for silencing the expression of E6/ E7 oncogenes in human cervical cells. Carbohydr Polym. 90: 1323–1329.
- Samal SK, Dash M, Van Vlierberghe S, Kaplan DL, Chiellini E, Van Blitterswijk C, et al. 2012. Cationic polymers and their therapeutic potential. Chem Soc Rev. 41:7147–7194.
- Shay JW, Zou Y, Hiyama E, Wright WE. 2001. Telomerase and cancer. Hum Mol Gen. 10:677–685.
- Takasaki S, Kotani S, Konagaya A. 2004. An effective method for selecting siRNA target sequences in mammalian cells. Cell Cycle. 3:788–793.
- Tuschl T, Zamore PD, Lehmann R, Bartel DP, Sharp PA. 1999. Targeted mRNA degradation by double-stranded RNA in vitro. Genes Dev. 13:3191–3197.
- Ui-Tei K, Naito Y, Takahashi F, Haraguchi T, Ohki-Hamazaki H, Juni A, et al. 2004. Guidelines for the selection of highly effective siRNA sequences for mammalian and chick RNA interference. Nucleic Acids Res. 32:936–948.
- Weber ND, Merkel OM, Kissel T, Muñoz-Fernández MÁ. 2012. PEGylated poly (ethylene imine) copolymer-delivered siRNA inhibits HIV replication in vitro. J Control Release. 157:55–63.
- Woods NB, Bottero V, Schmidt M, von Kalle C, Verma IM. 2006. Gene therapy: therapeutic gene causing lymphoma. Nature. 440:1123–1123.
- Xu F, Yang W. 2011. Polymer vectors via controlled/living radical polymerization for gene delivery. Prog Polym Sci. 36:1099–1131.
- Yamagishi M, Ishida T, Miyake A, Cooper DA, Kelleher AD, Suzuki K, Watanabe T. 2009. Retroviral delivery of promoter-targeted shRNA induces long-term silencing of HIV-1 transcription. Microbes Infect. 11:500–508.
- Zamore PD, Tuschl T, Sharp PA, Bartel DP. 2000. RNAi: double-stranded RNA directs the ATP-dependent cleavage of mRNA at 21 to 23 nucleotide intervals. Cell. 101:25–33.
- Zhang PH, Zou L, Tu ZG. 2006. RNAi-hTERT inhibition hepatocellular carcinoma cell proliferation via decreasing telomerase activity. J Surg Res. 131:143–149.
- Zhou J, Shum KT, Burnett JC, Rossi JJ. 2013. Nanoparticle-based delivery of RNAi therapeutics: progress and challenges. Pharmaceuticals. 6:85–107.