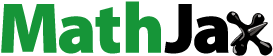
Abstract
In this article, a novel amperometric acetylcholine (ACh) biosensor with immobilization of acetylcholinesterase–choline oxidase (AChE–ChO) on electrochemically polymerized polyaniline–polyvinylsulphonate (PANI–PVS) film has been successfully developed for the first time. AChE and ChO enzymes were immobilized on PANI–PVS film by cross-linking with using glutaraldehyde (GA) and bovine serum albumin (BSA). Determination of ACh was carried out by oxidation of enzymatically produced H2O2 at +0.4 V versus Ag/AgCl. Optimum pH and temperature were found to be 8.0 and 60 °C, respectively. The linear part was in the region 1.0 × 10−7–6.0 × 10−7 M (R2 = 0.990). Storage stability and operation stability of the enzyme electrode were also studied.
Introduction
Acetylcholine [CH3COOCH2CH2N+(CH3)3] (ACh) is an ester that synthesized from choline (Ch) and acetyl coenzyme A (AcetylCoA) by choline acetyltransferase (ChAT). Systematic name of ACh is 2-acetoxy-N,N,N-trimethylethanaminium. ACh provides the transmission of messages between brain nerve cells (Carey Citation1990).
ACh is one of the most important neurotransmitters and also is the first discovered neurotransmitter in the literature (Heli et al. Citation2008, Shimomura et al. Citation2009). In the early twentieth century, Sir Henry Dale and other colleagues have done important studies on ACh and noradrenaline to contribute to the autonomic nervous system (Burnstock Citation2009). ACh plays a vital role in central and peripheral nervous system (Bowen et al. Citation1976, Deutsch & Rogers Citation1979, Shimomura et al. Citation2009). ACh affects memory, sleep, concentration ability and learning (Shimomura et al. Citation2009). Furthermore, ACh relates with dementia, Alzheimer’s Disease, Parkinson’s Disease (Davies & Maloney Citation1976, Heli et al. Citation2008) and schizophrenia (Heli et al. Citation2008, Tandon Citation1999). ACh also affects parasympathetic nerves. It causes slowing of heartbeat rate, increasing saliva and increasing bladder movements. Because of all these reasons, clinical and analytical determination of ACh is very important (Doretti et al. Citation2000, Pepeu & Giovanni Citation2004, Sattarahmady et al. Citation2010).
There are many enzyme biosensors in which dual enzymes reactions take place (Shimomura et al. Citation2009). The immobilization of enzymes on electrodes during the design and optimization of biosensors has attracted great interest (Çete et al. Citation2007). In general, ACh sensors are obtained by immobilizing suitable amounts of cholinesterase and choline oxidase (ChO) on electrode surface (Chen et al. 2008, Garguilo et al. Citation1993, Navera et al. Citation1991). In these biosensors, ACh determination is achieved by oxidation of H2O2 which occurs after AChE and ChO reactions (Chen et al. 2008). Choline is a hydrolytic product of acetylcholine; it reacts with ChO and H2O2 is formed (Özdemir et al. Citation2012, 2014).
Numerous AChE-based biosensors designed in various techniques such as potentiometric (Liu et al. Citation2005, Timur et al. Citation2004), amperometric (Hou et al. Citation2012), electrochemiluminescence (Hou et al. Citation2012, Wang et al. Citation2009) and fluorescence (Hou et al. Citation2012, Öztürk et al. Citation2007). Amperometric dual AChE/ChO biosensors are promising between AChE-based biosensors due to their high precision, good nature and improvable properties (Hou et al. Citation2012).
Various immobilization techniques have been used for amperometric ACh biosensors (Shimomura et al. Citation2009). However, traditional methods such as entrapment in polymer gel cannot always give high response or be adequately improved methods due to loss of enzyme activity during the immobilization period (Albareda-Sirvent et al. Citation2000, Cosnier Citation1999, Shimomura et al. Citation2009). Therefore, enzyme immobilization method is one of the most important factors for enzyme-based biosensors. For immobilizing of enzymes, conductive polymers are promising materials. PANI is a conductive polymer (Sari et al. Citation2002). In this work, PANI conductive polymer was doped with PVS.
Amperometric response of sensor is caused by H2O2 oxidation current (Arslan et al. Citation2006). So, the performance of ACh biosensors significantly depends on the catalytic activity of immobilized AChE and ChO on the surface (Chen et al. Citation1998).
In this work, a new amperometric biosensor for determination ACh was prepared. For this reason, AChE and ChO enzymes have been immobilized at polyaniline–polyvinylsulphonate (PANI–PVS) film by cross-linking. ACh detection was based on the oxidation of enzymatically produced H2O2, at +0.4 V. Optimum conditions of enzyme electrode were determined. The effects of pH and temperature on the response of the ACh biosensor were investigated. The linear working range of enzyme electrode was determined. After then, reuseability and storage stability of the biosensor were determined. Interference effects were investigated on the amperometric response of the biosensor.
Materials and methods
Equipment and reagents
The electrochemical studies were carried out by using Epsilon EC electrochemical analyzer with a three-electrode cell. The working electrode was a Pt plate (0.5 cm2). Auxiliary and reference electrodes were Pt wire and Ag/AgCl electrode (3.0 M KCl), respectively. The pH values of the buffer solutions were measured with an ORION Model 720A pH/ion meter. Temperature control was achieved with Grant GD 120 thermostat. Acetylcholinesterase (EC 3.1.1.7, purified from Electrophorus electricus (electric eel) Type V-S, activity of 100.0 unit/mL) and choline oxidase (EC 1.1.3.17, purified from the Alcaligenes species, activity of 5.0 unit/mL) were purchased from Sigma. Acetylcholine chloride was also purchased from Sigma. Aniline and sodium polyvinylsulphonate were supplied from Merck and Aldrich, respectively. All other chemicals were obtained from Sigma. All the solutions were prepared by using distilled water.
Preparation of Pt/PANI–PVS film electrode
The surface of the Pt plate electrode was cleaned (Gros et al. Citation2000) and covered with PANI–PVS film by electropolymerization of aniline in a medium containing sodium polyvinylsulphonate. Ten microliters of solution containing 0.2 M aniline, 2.5 mL (25%) sodium polyvinylsulphonate was prepared (Arslan et al. Citation2011). Pt electrode was immersed into prepared solution and the system was purged with argon for 10 min in order to remove the oxygen. The electropolymerization was carried out on the Pt electrode surface by using constant potential of +0.75 V for 1 h (versus. Ag/AgCl electrode (3 M KCl)) (Arslan et al. Citation2011). The electrode was washed with deionized water after the coating process to remove the unreacted aniline monomer.
Immobilization of AChE–ChO on Pt/PANI–PVS film electrode
After electropolymerization, the mixture solution of 50.0 μL of AChE (5.0 unit/mL), 50.0 μL of ChO (5.0 unit/mL), 1.0 mg of BSA, 50.0 μL of 0.1 M phosphate buffer at pH of 8.0 and 15.0 μL of 2.5% GA was dropped on Pt/PANI–PVS film (Aynacı et al. Citation2014). Electrode was dried at room temperature and washed with buffer solution (0.1 M phosphate buffer of pH 8.0) several times in order to remove the excess nonimmobilized enzyme and GA. Immobilized enzyme electrode was kept in a refrigerator at +4 °C in phosphate buffer, when not in use.
Amperometric measurements
Quantification of ACh was achieved via electrochemical detection of enzymatically released H2O2.
Enzyme electrode was immersed into phosphate buffer solution (0.1 M, at pH 8.0) containing 0.1 M sodium chloride as supporting electrolyte. Electrode was brought to equilibrium by keeping it at +0.4 V (versus Ag/AgCl electrode (3.0 M KCl)). Steady-state current (ia) was recorded. After, ACh solution was added to the cell from stock solution and the system was stirred. Current (ib) values obtained at +0.4 V were recorded and current values (Δi = ib − ia) were plotted against the ACh concentration.
Results and discussions
In this study, we reported a new AChE/ChO based amperometric biosensor for the determination of ACh. AChE and ChO were immobilized onto PANI–PVS film surface by cross-linking with GA and BSA for the first time. The determination of ACh was performed by oxidation of H2O2, which occurs after enzymatic reactions at +0.4 V versus Ag/AgCl. , which demonstrates the ACh determination, is shown below.
According to this scheme, a biochemical reaction occurs between ACh in solution and AChE–ChO enzymes which are immobilized onto PANI–PVS electrode. At first, ACh is hydrolyzed to Ch and acetate by AChE. Then, Ch is oxidized to betaine by ChO. And flavin adenine dinucleotide (FAD), which is a prosthetic group in enzyme structure, is reduced to FADH2 by taking electron. FADH2 in enzyme is oxidized by giving its electrons to oxygen in solution to make the reaction reversible. Thus, enzyme comes to its previous form. By taking the electron, oxygen is reduced to H2O2. ACh determination was made by measuring the anodic current of hydrogen peroxide on electrode surface (Aynacı et al. Citation2014). The parameters affecting the performance of the biosensor and optimum working conditions were investigated.
Determination of working potential
After preparing Pt/PANI–PVS electrode, H2O2 oxidation was carried out at different potentials (+0.2–+0.6 V) (). When results were examined, the oxidation currents of H2O2 at +0.2 V were too low; therefore, currents of H2O2 at +0.2 V was not shown in . Compared with other potentials, the oxidation currents of H2O2 at +0.3 V were too low. Interference effects of substances presented in body fluids (e.g. ascorbic acid, uric acid) could be more in high potentials (Zhang et al. Citation2007). Therefore, +0.4 V was used as working potential.
Effect of pH on amperometric response of biosensor
One of the most important factors affecting the enzyme activity is pH. Each enzyme has a pH value which shows maximum activity (Nelson and Cox Citation2005). The pH value depends on the charge of enzyme or matrix (Yıldırımoğlu et al. Citation2009). Effect of pH on the response of the ACh biosensor was tested with more than one buffer solutions varied between pH 6.0 and pH 10.0. The measurements were performed at a constant ACh concentration 2.5 × 10−6 M with 0.1 M phosphate, glycine and borate buffers diverse between pH 6.0 and 10.0. As seen in , the maximum response was obtained at pH 8.0.
Figure 3. The effect of pH on the response of the biosensor (at 2.5 × 10−6 M ACh, at a +0.4 V operating potential).





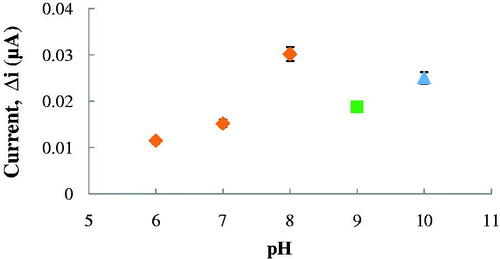
For ACh biosensors, optimum pH was reported as 8.0 (Doretti et al. Citation2000), 7.5 (Hou et al. Citation2012), 9.0 (Aynacı et al. Citation2014) and 8.3 (Larsson et al. Citation1998). The difference in pH values depends on the used polymer and immobilization type. Also, immobilized enzymes can show high activities under different pH conditions when compared with free enzyme (Aynacı et al. Citation2014, Erduran et al. Citation2013).
Effect of temperature
Temperature is an important factor which affects stability, activity and the rate of the enzymatic reaction. Therefore, it is important to examine how the current changes with the temperature of the prepared biosensor’s answer. The relationship between reaction rate of enzymes and temperature is exponential (Arslan et al. Citation2012). The amperometric response of the prepared biosensor was investigated at different temperatures by using constant Ach concentration of 2.5 × 10−6 M (). As seen from , the current difference increased with temperature up to 60 °C and decreased afterward. The highest current difference was obtained at 60 °C. The enzyme was thought to be denaturized after this temperature. Due to the difficulties involved in working at 60 °C, the studies were carried out at 25 °C.
Figure 4. The effect of temperature on the response of the biosensor (at pH 8.0, 2.5 × 10−6 M ACh at a +0.4 V operating potential).
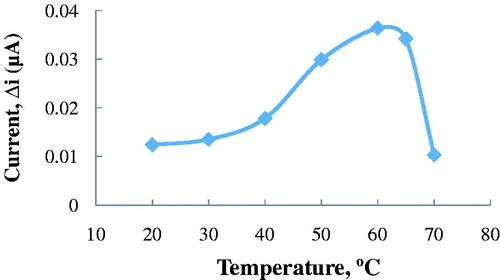
For ACh biosensors, temperature values different than 60 °C were employed in the literature as 35 °C (Doretti et al. Citation2000) and 55 °C (Şen et al. Citation2004). This was because the used polymer and the type of the immobilization were different.
Substrate concentration and calibration curves
The effect of the substrate concentration on the reaction rate, catalyzed by immobilized enzymes, was studied by using varying concentrations 1.0 × 10−7–5.0 × 10−6 M ACh (). There was a the linear part in the region between 1.0 × 10−7–6.0 × 10−7 M (R2 = 0.990) (). When compared with the literature (2.0 × 10−4–1.3 × 10−3 M and 1.2 × 10−4–1.0 × 10−2 M, respectively, for Snejdarkova et al. Citation2004 and Kanik et al. Citation2013), biosensor is sensitive to low ACh concentration. It has been shown that the linearity of this graph is highly satisfactory and it can be used for the quantitative determination of ACh.
Figure 5. The effect of ACh concentration on the amperometric response of the biosensor (Michaelis–Menten plot, at 0.1 M, pH 8.0 phosphate buffer, 25 °C, +0.4 V operating potential).
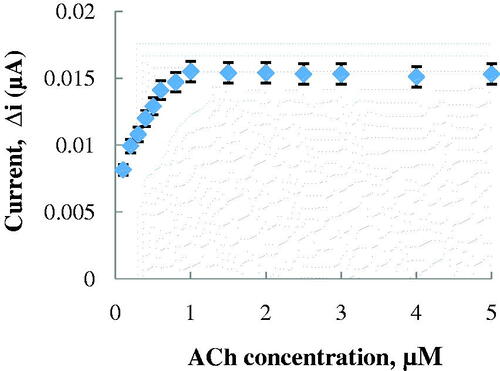
The low detection limit of the biosensor was found to be 5.0 × 10−8 M and response time for the biosensor was 200 s. Because of the reasons that prepared ACh biosensor has low detection limit and provide to work in low concentrations, ACh determination can be made with a variety of different samples with our biosensor. Km(app) and Imax(app) values were found to be 1.007 × 10−4 mM and 0.0157 μA, respectively, from Lineweaver–Burk plot (). The Km(app) value that showed the affinity of the biosensor was 1.007 × 10−4 mM and the values, cited in the literature, were 0.4 mM (Doretti et al. Citation2000), 1.74 mM (Şen et al. Citation2004) and 3.5 mM (Li et al. Citation1999). PANI–PVS film, used in our study, increased the affinity of enzymes to ACh. It can be understood from the Km(app) value which is lower than the Km(app) values in literature.
Operational stability of the Ach biosensor
In order to detect the operational stability of the prepared enzyme electrode, the current changes, obtained after subsequent usage, were plotted against the number of measurements. At the end of 15 measurements, the initial activity of the biosensor nearly unchanged. It had lost 0.5% of its initial activity. The relative standard deviation, obtained after 15 measurements at a constant ACh concentration of 2.5 × 10−6 M, was found to be 4.43% ().
Storage stabilization of the enzyme electrode
One of the most important properties for the enzyme electrode is the longevity of the electrode. Response of the enzyme electrode, prepared under optimum conditions, was measured for a period of 14 days at constant ACh concentration (2.5 × 10−6 M). Results of 8 measurements during this period were plotted in . It was seen that there was a decrease in current values between the first and fourteenth days. The electrode showed 16.51% of the initial amperometric response at the end of the 14 days.
Interference effects
Some common substances in serum were investigated with Pt/PANI–PVS film for interfering effect on the analysis of ACh. The response current for a constant concentration of ACh (4.0 × 10−5 M) was compared with the current value, obtained in the presence of the variable concentrations of the interfering species. These interferants and their concentrations were glucose (5.0 × 10−3 M), uric acid (3.0 × 10−4 M), ascorbic acid (1.0 × 10−4 M), paracetamol (1.0 × 10−4 M), cystine (6.5 × 10−5 M) and cysteine (1.0 × 10−4 M). By diluting, the interference effects of electroactive species can be eliminated (Çete et al. Citation2006, Perez et al. Citation2003). After diluting 200-fold, the results indicated that in the presence of glucose, uric acid, ascorbic acid, paracetamol, cystine and cysteine, the response current was increased by 0.05–12.2%, respectively ().
Table I. Effect of interferants on ACh biosensor.
ACh determination in synthetic blood sample
A synthetic blood sample that contains body concentrations of some interferants was prepared. These interferants and their concentrations were glucose (5.0 × 10−3 M), uric acid (3.0 × 10−4 M), ascorbic acid (1.0 × 10−4 M), paracetamol (1.0 × 10−4 M), cystine (6.5 × 10−5 M) and cysteine (1.0 × 10−4 M). ACh concentration was kept as 4.0 × 10−5 M. The synthetic blood sample was added in a cell and diluted 200-fold. With using the calibration curve, ACh amount was determined after 3 experiments as (5.21 ± 0.105) × 10−5 M.
Conclusion
In this study, we report a new AChE–ChO based amperometric biosensor for the determination of ACh. AChE and ChO were both immobilized onto a PANI–PVS film surface by cross-linking with GA and BSA, for the first time. In this study, prepared ACh biosensor has a low detection limit and an acceptable response time for a biosensor. Because of low interference effects of different interferants, ACh determination can be made with this biosensor in a variety of different samples. It was seen that the ACh biosensor was selective and its operational stability was found to be excellent. Thus, ACh determination can be made with our ACh biosensor for many times. Our ACh biosensor is easy to prepare and is highly cost-effective. PANI–PVS composite can supply a biocompatible and electrochemical microenvironment for immobilization of the enzyme, making this material a good candidate for the fabrication of highly sensitive and selective ACh biosensors.
Declaration of interest
The authors report no conflicts of interest. The authors alone are responsible for the content and writing of this article.
References
- Albareda-Sirvent M, Merkoci A, Alegret S. 2000. Configurations used in the design of screen-printed enzymatic biosensors (a review). Sens Actuators B: Chem. 69:153–163.
- Arslan F, Ustabaş S, Arslan H. 2011. An amperometric biosensor for glucose determination prepared from glucose oxidase immobilized in polyaniline-polyvinylsulfonate film. Sensors. 11:8152–8163.
- Arslan F, Yaşar A, Kiliç E. 2006. Preparation of Pt/polypyrrole-ferrocene hydrogen peroxide sensitive electrode for the use as a biosensor. Russ J Electrochem. 42:137–140.
- Arslan H, Özdemir M, Zengin H, Zengin G. 2012. Glucose biosensing at carbon paste electrodes containing polyaniline-silicon dioxide composite. Int J Electrochem Sci. 7:10205–10214.
- Aynacı E, Arslan F, Yaşar A. 2014. An amperometric biosensor for acetylcholine determination prepared from acetylcholinesterase-choline oxidase immobilized in polypyrrole-polyvinylsulpfonate film. Sens Actuators B: Chem. 202:1028–1036.
- Aynacı E, Sarı N, Tümtürk H. 2011. Immobilization of β-galactosidase on novel polymers having Schiff bases. Artif Cell Blood Substit. 39:259–266.
- Bowen DM, Smith CB, White P, Davison AN. 1976. Neurotransmitter related enzymes and indices of hypoxia in senile dementia and other abiotrophies. Brain. 99:459–66.
- Burnstock G. 2009. Autonomic neurotransmission: 60 years since Sir Henry Dale. Annu Rev Pharmacol Toxicol. 49:1–30.
- Carey J. 1990. Brain Facts: A Primer on the Brain and Nervous System. Washington, DC: Society for Neuroscience
- Chen Q, Kobayashi Y, Takeshita H, Hoshi T, Anzai J. 1998. Avidin-biotin system-based enzyme multilayer membranes for biosensor applications: optimization of loading of choline esterase and choline oxidase in the bienzyme membrane for acetylcholine biosensors. Electroanalysis. 10:94–97.
- Cosnier S. (1999). Biomolecule immobilization on electrode surfaces by entrapment or attachment to electrochemically polymerized films (a review). Biosens Bioelectron. 14:443–456.
- Çete S, Yaşar A, Arslan F. 2006. An amperometric biosensor for uric acid determination prepared from uricase immobilized in polypyrrole film. Artif Cell Blood Substit. 34:367–380.
- Çete S, Yaşar A, Arslan F. 2007. Immobilization of uricase upon polypyrrole-ferrocenium film. Artif Cell Blood Substit. 35:607.
- Davies P, Maloney AJ. 1976. Selective loss of central cholinergic neurons in Alzheimer’s disease. Lancet. 2:1403.
- Deutsch JA, Rogers JB. 1979. Cholinergic excitability and memory: Animal studies and their clinical implications. In: Davis KL, Berger PA, Eds. Brain Acetylcholine and Neuropsychiatric Disease, Springer US. 175–204.
- Doretti L, Ferrara D, Lora S, Schiavon F, Veronese FM. 2000. Acetylcholine biosensor involving entrapment of acetylcholinesterase and poly (ethylene glycol) modified choline oxidase in a poly (vinyl alcohol) cryogel membrane. Enzyme Microb Tech. 27:279–285.
- Erduran V, Tümtürk H, Aynacı E, Sarı N. 2013. Immobilization of β-galactosidase on polystyrene microspheres attached l-alanine, J Macromol Sci A. 50:871–878.
- Garguilo G, Huynh N, Proctor A, Michael AC. 1993. Amperometric sensors for peroxide, choline and acetylcholine based on electron transfer between horseradish peroxidase and a redox polymer. Anal Chem. 65:523.
- Gros P, Durliat H, Comtat M. 2000. Use of polypyrrole film containing Fe(CN)63- as pseudo-reference electrode: application for amperometric biosensors. Electrochim Acta. 46:643–650.
- Heli H, Hajjizadeh M, Jabbari A, Moosavi-Mohavedi AA. 2008. Copper nanoparticles-modified carbon paste transducer as a biosensor for determination of acetylcholine. Biosens Bioelectron. 24:2328–2333.
- Hou S, Ou Z, Chen Q, Wu B. 2012. Amperometric acetylcholine biosensor based on self-assembly of gold nanoparticles and acetylcholinesterase on the sol-gel/multi-walled carbon nanotubes/choline oxidase composite-modified platinum electrode. Biosens Bioelectron. 33:44–49.
- Kanik FE, Kolb M, Timur S, Bahadır M, Toppare L. 2013. An amperometric acetylcholine biosensor based on a conducting polymer. Int J Biol Macromol. 59:111–118.
- Larsson N, Ruzgas T, Gorton L, Kokaia M, Kissinger P, Csöregi E. 1998. Design and development of an amperometric biosensor for acetylcholine determination in brain microdialysates. Electrochim Acta. 43:3541–3554.
- Li Y, Zhou Y, Feng J, Jiang Z, Ma L. 1999. Immobilization of enzyme on screen-printed electrode by exposure to glutaraldehyde vapour for the construction of amperometric acetylcholinesterase electrodes. Anal Chim Acta. 382:277–282.
- Liu B, Yang YH, Wu ZY, Wang H, Shen LS, Yu RQ. 2005. A potentiometric acetylcholinesterase biosensor based on plasma-polymerized film. Sens Actuators B: Chem. 104:186–190.
- Navera EN, Sode K, Tamiya S, Karube I. 1991. Development of acetylcholine sensor using carbon fiber (amperometric determination). Biosens Bioelectron. 6:675.
- Nelson DL, Cox MM. 2005. Lehninger Principles of Biochemistry. 3rd ed. Turkey: Worth Publishers.
- Özdemir M, Arslan F, Arslan H. 2012. An amperometric biosensor for choline determination prepared from choline oxidase immobilized in polypyrrole–polyvinylsulfonate film. Artif Cell Blood Substit. 40:280–284.
- Özdemir M, Arslan H. 2014. Choline-sensing carbon paste electrode containing polyaniline (pani)–silicon dioxide composite-modified choline oxidase. Artif Cell Blood Substit. 42:27–31.
- Özturk G, Alp S, Timur S. 2007. A fluorescent biosensor based on acetylcholinesterase and 5-oxazolone derivative immobilized in polyvinylchloride (PVC) matrix. J Mol Catal B- Enzym. 47:111–116.
- Pepeu G, Giovanni MG. 2004. Changes in acetylcholine extracellular levels during cognitive processes. Learn Memory.11:21–27.
- Perez DM, Ferrer ML, Mateo CR. 2003. A reagent less fluorescent sol-gel biosensor for uric acid detection in biological fluids. Anal Biochem. 322:238–242.
- Sari B, Talu M, Yildirim F. 2002. Electrochemical polymerization of aniline at low supporting-electrolyte concentrations and characterization of obtained films. Russ J Electrochem. 38:707–713.
- Sattarahmady N, Heli H, Moosavi-Mohavedi AA. 2010. An electrochemical acetylcholine biosensor based on nanoshells of hollow nickel microspheres-carbon microparticles-nafion nanocomposite. Biosens Bioelectron. 25:2329–2330.
- Şen S, Gülce A, Gülce H. 2004. Polyvinylferrocenium modified Pt electrode for the design of amperometric choline and acetylcholine enzyme electrodes. Biosens Bioelectron. 19:1261–1268.
- Shimomura T, Itoh T, Sumiya T, Mizukami F, Ono M. 2009. Amperometric biosensor based on enzyms immobilized in hybrid mesoporous membranes for the determination of acetylcholine. Enzyme Microb Tech. 45:443–448.
- Snejdarkova M, Svobodova L, Evtugyn G, Budnikov H, Karyakin A, Nikolelis DP, Hianik T. 2004. Acetylcholinesterase sensors based on gold electrodes modified with dendrimer and polyaniline A comparative research, Anal Chim Acta. 514:79–88.
- Tandon, R. 1999. Cholinergic aspects of schizophrenia. Br J Psychiatry. Suppl. 174:7–11.
- Timur S, Telefoncu A. 2004. Acetylcholinesterase (AChE) electrodes based on gelatin and chitosan matrices for the pesticide detection. Artif Cell Blood Substit. 32:427–442.
- Wang XF, Zhou Y, Xu JJ, Chen HY. 2009. Signal-on electrochemiluminescence biosensors based on cds–carbon nanotube nanocomposite for the sensitive detection of choline and acetylcholine. Adv Funct Mater. 19:1–17.
- Yıldırımoğlu F, Arslan F, Çete S, Yaşar A. 2009. Preparation of a polypyrrole-polyvinylsulphonate composite film biosensor for determination of cholesterol based on entrapment of cholesterol oxidase. Sensors. 9:6435–6445.
- Zhang Y, Wen G, Zhou Y, Shuang S, Dong C, Choi MM. 2007. Development and analytical application of an uric acid biosensor using an uricase-immobilized eggshell membrane. Biosens Bioelectron. 22:1791–1797.