Abstract
Platelet micro-particles (MPs) contain CXCR4 markers and are able to transfer them into hematopoietic stem cells. Therefore, effect of platelet MPs (PMPs) on the expression levels of CXCR4 and CD34 markers in these cells was examined. Isolated CD 133+ cells cultivated for 5 d in the stem span medium and PMPs. Fold increase of CD34+ cells in the presence of 5 and 10 g/ml of PMPs was increased significantly. CXCR4+ cell percent in the presence of 10 g/ml PMPs compared with control cells (63.8 ± 6.4) was increased (P < 0.05). PMPs were no affect on clonogenicity of hematopoietic progenitor cells. Background: Cord blood CD133+ cells are able to maintain long-term hematopoiesis and to differentiate to hematopoietic lineages. CXCR4 over expression is involved in homing and successful transplantation of hematopoietic stem cells (HSCs) in the bone marrow. PMPs contain CXCR4 markers and are able to transfer them into hematopoietic stem cells. Therefore, considering the importance of CD133+ cells as primitive HSCs, the effect of PMPs on the expression levels of CXCR4 and CD34 markers in these cells was examined. Materials and methods: Cord blood CD133+ cells were isolated by MACS. Isolated cells were divided into three groups: (i) control cells, (ii) cells treated with 5 μg/ml PMPs, (iii) cells treated with 10 μg/ml PMPs. Cells were cultivated for 5 d in the stem span medium. Expression of CD 133, CD34, and CXCR4 surface marker was analyzed by flow cytometry. Total cell numbers were counted by hemocytometer and clonogenicity were measured by colony assay. Results: PMPs were no effect on CD133+ cells proliferation, but fold increase of CD34+ cells in the presence of 5 and 10 g/ml of PMPs was increased significantly. CXCR4+ cell percent in the presence of 10 g/ml PMPs compared with control cells (63.8 ± 6.4) was increased (P < 0.05). PMPs were no affect on clonogenicity of hematopoietic progenitor cells. Conclusions: Exposure of CD133+ cells isolated from cord blood to PMPs with 10 μg/ml concentration increased the expression of CXCR4 surface marker significantly.
Introduction
Self-renewal, proliferation, differentiation, and homing of hematopoietic stem cells (HSCs) are regulated by a mechanism that involves the bone marrow (BM) microenvironment. Cell adhesion molecules (CAMs) expressed on HSCs and stromal cells play a pivotal role in this process (Timeus et al. Citation1998). HSCs and stromal cells linked together by adhesion molecules (e.g., LFA-1, VLA-4, N-cadherin, CXCR-4, and integrins) and thereby keep their abilities and control their destiny (Iwasaki and Suda Citation2010). Chemokine receptor CXC chemokine receptor-4 (CXCR4) is a G-protein double receptor that pass seven times through cell membranes. Recently it has been shown that CXCR4-SDF 1 signaling pathway plays essential role in homing, transplantation (Wysoczynski et al. Citation2005), self-renewal, survival, motility (Iwasaki and Suda Citation2010), implantation in their proper niche, and proliferation of HSCs (Nie et al. Citation2008). The over expression of CXCR4 in the HSCs membranes leading to better respond to the gradient of stem cell-derived factor (SDF1) and efficient engraftment (Wysoczynski et al. Citation2005).
Micro-particles (MPs) are small membrane vesicles that are separated from the plasma membrane of different cell types such as platelets, erythrocytes, leukocytes, and endothelial cells by budding (Nomura et al. Citation2008, Rank et al. Citation2010). Platelet micro-particles (PMPs) are the most abundant MPs in blood flow, approximately 70–90%, which have a diameter of less than 1 μm (Flaumenhaft Citation2006, Siljander Citation2011). The PMPs may lead to functional changes in the recipient cells due to intracellular transferring of bio-active molecules, such as intracellular lipid, surface receptors, and enzymes (Siljander Citation2011). Platelet endothelial receptors (CD41, CD61, and CD62) (Baj-Krzyworzeka et al. Citation2002, Janowska-Wieczorek et al. Citation2001, Nomura and Fukuhara Citation2004, Rank et al. Citation2010), G-protein double receptor (PAR-1 and CXCR-4), and cytokine receptors (Baj-Krzyworzeka et al. Citation2002, Janowska-Wieczorek et al. Citation2001) have been identified in the PMPs surface marker. PMPs can be connected to CD34+ HSCs and lead to the increased HSCs transplantation ability by stimulating their proliferation, survival, adhesion, and chemotaxis (Nomura et al. Citation2008). The CD34+ HSCs are coated with PMPs express several new adhesion molecules and to be connected to endothelial cells and fixed cells represent SDF1 more strongly (Janowska-Wieczorek et al. Citation2001). In this study, we evaluated PMPs effect on expression of CXCR4 in cord blood-derived CD133+ HSCs.
Material and methods
PMPs preparation
PMPs were obtained from platelet concentrates after 3 d of preparation. The platelet concentrate was frozen at −80 °C and was thawed at 37 °C in a water bath for three times. The obtained suspension was ultra sonicated by a probe sonicator (Hielscher, Teltow, Germany). The sonication was performed at 0.5 s cycles separated by intervals of 15 s. The suspension finally was centrifuged at 3000 RPM for 10 min. The supernatant were analyzed for CD42b and CD61 expressions on PMPs by flow cytometry. The protein content of the MPs was measured by the Bradford method. The supernatant was immediately frozen and kept in −70 °C until use.
Measurement of PMPs protein content by the Bradford method
The protein concentration of PMPs was measured by Bradford assay. A standard curve based on the absorbance of different concentrations of BSA protein (Sigma, St. Louis, MO) was obtained at 595 nm.
Evaluation of CD42b and CD61 expression on PMPs by flow cytometry
Fifty microliter of PMPs was incubated with GPIb mouse monoclonal antibodies (PE conjugated-Dako, Dako Denmark A/S, Glostrup, Denmark) and GPIIIa mouse monoclonal antibodies (FITC conjugated-Dako, Dako Denmark A/S Glostrup, Denmark) at 4 °C for 30 min. Then the cells were washed in PBS to remove free antibodies. Isotype negative controls were used in every experiment. The fluorescence of MPs was analyzed in a flow cytometry (Partec, Görlitz, Germany) and CD marker expression was determined.
Isolation of CD133+cells
HSCs were collected from fresh human umbilical cord blood (n = 3) after written informed consent from normal full-term pregnant women, according to guidelines approved by the Ethics Committee of the Iranian Blood Transfusion Organization.
Due to high red blood cells in cord blood, the RBCs were precipitated using hydroxyl ethyl starch (Stem Cell Technologies, Ottawa, Canada). Mononuclear cells were isolated by density gradient centrifugation on Ficoll-Hypaque (Bio Sciences, Uppsala, Sweden). The MNCs incubated with anti-human CD133 conjugated with Micro Beads (Miltenyi Biotec, San Diego, CA) for 30 min at 4 °C. CD133+ cells were isolated using an affinity column. The purity percent of CD133 enriched cells was detected by flow cytometry.
Cultivation of CD133+ enriched cells
About 1 × 104 CD133+enriched cells (n = 3) were cultured in each well of 24 well plates (Nunc, Roskilde, Denmark) (duplicate, n = 6) in the stem span medium (Stem Cell Technology, Gentofte, Denmark). The culture medium supplemented with SCF, TPO, and FLT3L (100 ng/ml) (Stem Cell Technology, Gentofte, Denmark). About 5 or 10 µg/ml of PMPs were added to cells and was incubated at 37 °C under 5% CO2 humidified air for 5 d. Controls were used in every experiment without PMPs.
Colony-forming cell assays
Fresh CD133+cells and treated cells with 5 or 10 µg/ml of PMPs for 5 d were seeded in the semisolid culture (MethoCult GF H4434, Stem Cell Technologies, Ottawa, Canada) following the instruction of the manufacturer. Methylcellulose-based media were aliquoted in 35-mm Petri dishes and incubated at 37 °C, 5% CO2, and humidified incubator. After 14 d of culture, the number of colonies was counted under the inverted microscope.
Proliferative and phenotypic analysis of treated HSCs with PMPs
The cell viability was determined using trypan blue staining (Stem Cell Technologies, New Haven, CT), and the expression of CD133, CD34, and CXCR4 markers on fresh and treated cells were analyzed by flow cytometry (4-color, SY-Space Partec, Münster, Germany) using monoclonal antibodies at days 0 and 5 of culture. Flow cytometric analysis was performed by incubating harvested cells with different fluorescent conjugated mouse monoclonal antibodies (Dako, Glostrup, Denmark) at 4 °C for 30 min. Then the cells were washed in PBS and fixed with 2% Para formaldehyde (Sigma, St. Louis, MO). Isotype controls were used in every experiment.
Statistical analysis
The data were compared using the ANOVAs test in SPSS16 software (SPSS Inc., Chicago, IL). Statistical significance was defined as P < 0.05.
Results
Measurement of PMPs protein content by the Bradford method
PMPs were obtained from 12 × 108 PLT/ml by freeze-thaw and sonication methods. The protein concentration of PMPs was detected by Bradford assay and was 195 µg/ml.
Evaluation of CD42b and CD61 expressions on PMPs by flow cytometry
The prepared PMPs were characterized by flow cytometric analysis for CD42b (GPIb) and CD61 (β3 integrin) surface antigens. As shown in , 73.70% of PMPs were found to be dual positive for the both CD42b and CD61, whereas 93.33% were positive for CD61 and 74.72% of them for CD42b surface marker ().
Phenotypic analysis of fresh HSCs by flow cytometry
Flow cytometric analysis was shown to be 85.8% ± 4.74 purity for separated CD133+cells by MACS. Moreover, the cell viability was 90 ± 4.3 (n = 3).The expression of CD34 and CXCR4 markers on isolated cells was 73.1 ± 3.9% % and 56.4 ± 4.45%, respectively (). Moreover, 70.8 ± 4.15 of cells was shown co-expression of both CD34 and CD133, and 46.4 ± 4.22% were found dual positive for both CD34 and CXCR4.
Proliferation analysis of CD133+ and CD34+ cells
Ex vivo expansion of human cord blood CD133+enriched cells in serum-free medium supplemented with SCF, TPO, and FLT3L was evaluated either with (5 and 10 µg/ml) or without PMPs (controls) using flow cytometeric analysis. The mean fold change of total nucleated cells was 5.59 ± 0.48 for control wells. The mean fold change of CD133+ cells was 5.36 ± 0.65 for controls, 5.52 ± 0.57 for treating cells with 5 µg/ml PMPs, and 5 ± 0.59 for treating cells with 10 µg/ml PMPs at day 5 of culture (). There were no significant differences between two groups of culture and controls. Therefore, PMPs had no effect on CD133+ cells proliferation.
Figure 3. Proliferation analysis of treated and un-treated CD133+ cells at day 5 of culture. There were no significant differences between two groups of culture and controls (n = 6, P > 0.05).
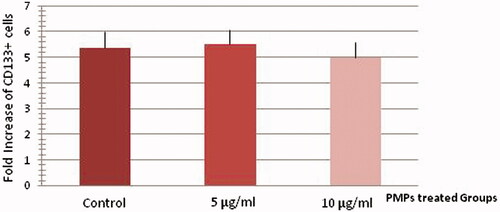
The mean fold change of treated CD34+ cells with 5 µg/ml (6.79 ± 0.45) and 10 µg/ml (6.86 ± 0.46) PMPs was higher than control CD34+ cells (5.47 ± 0.68) at day 5 of culture (n = 6, P < 0.05). There were no significant differences between two groups of treated cells ().
CXCR4 expression and CD34–CXCR4 co-expression analysis of treated HSCs with PMPs
The mean fold change of treated CXCR4+ cells with 10 µg/ml (7.18 ± 0.73) PMPs was higher than the mean fold change of control cells (5.93 ± 1.08) at day 5 of culture (n = 6, P < 0.05), but the fold increase of treated cell with 5 µg/ml (6.84 ± 0.96) PMPs was not significant in comparison with mean fold change of controls. There were no significant differences between two groups of treated cells ().
Figure 5. CXCR4 expression analysis of treated HSCs with PMPs. The mean fold change of treated CXCR4+ cells with 10 µg/ml PMPs was higher than the mean fold change of control cells at day 5 of culture (n = 6, P < 0.05).
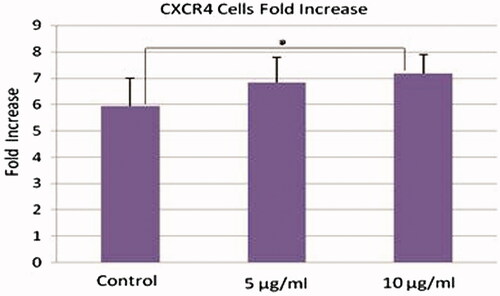
The mean fold change of treated dual positive CXCR4–CD34+ cells with 5 µg/ml (6.9 ± 1.49) and 10 µg/ml (7.51 ± 1.29) PMPs was higher than control CXCR4-CD34+ cells (5.85 ± 1) at day 5 of culture (n = 6, P < 0.05) ().
Colony-forming cell assays
Colony forming potential of freshly separated CD133+cells at day 0 was compared with control and treated cells with 5 and 10 µg/ml of PMPs at day 5 of culture in the semisolid medium. The highest CFU folds change was observed in un-treated control cells after 5 d in cytokine-supplemented culture (n = 3, P < 0.05, ).
Discussion
Examples of successful cord blood transplants in the last decade indicate that cord blood cells can be a good replacement for the bone marrow derived stem cells (Kita et al. Citation2011). Hematopoietic stem and progenitor cells from umbilical cord blood have a high capacity for self-renewal and rehabilitation of their population (Kita et al. Citation2011). HSC's ability to efficiently homing in the bone marrow is considered the main output of the transplant, and primitive HSCs need to SDF1/CXCR4 interaction for efficient implant in bone marrow (Nie et al. Citation2008). SDF-1/CXCR4 signaling is critical to migrate and anchoring of the transplanted stem cells into the bone marrow endothelial (Papayannopoulou et al. Citation2003). Binding of PMPs to hematopoietic progenitor cell membranes is a common mechanism (Janowska-Wieczorek et al. Citation2001) that increases adhesion of CD34+ cells to endothelial. So, they will improve implantation in the bone marrow (Baj-Krzyworzeka et al. Citation2002). Janowska et al. reported that the mouse bone marrow-derived HSCs treated with PMPs implant in their niche faster than the control group, without PMPs (Janowska-Wieczorek et al. Citation2001).
Therefore, in this study, we evaluated PMPs effect on expression of CXCR4 in cord blood derived CD133+ HSCs for the first time. Our data suggest that PMPs have no effect on CD133+ cells proliferation after 5 d, but the proliferation of CD34+ cells has been increased after treatment with 5 and 10 μg/ml PMPs. To support this, Krzyworzeka et al. demonstrated for the first time that under serum-free conditions, PMPs stimulate the proliferation of human CD34+ cells after 4 d (Baj-Krzyworzeka et al. Citation2002), while Janowska et al. stated that humans and mice CD34+ HSCs that were treated with PMPs had no significant difference in proliferation with untreated cells (Janowska-Wieczorek et al. Citation2001).
Krzyworzeka suggested that platelet MPs transfer platelet receptors such as PAR1, CD41, CD62, and CXCR4 to surface of human CD34+ cell (Baj-Krzyworzeka et al. Citation2002), and leading to regulate proliferation, survival, and adhesion of these cells in the hematopoietic organs (Baj-Krzyworzeka et al. Citation2002, Nomura and Fukuhara Citation2004).
We observed the CXCR4 expression after treatment with 10 μg/ml PMPs increased significantly, while after treatment with 5 μg/ml, PMPs have no significant in crescent. Also co-expression of CXCR4-CD34 surface markers increment after treatment with 5 and 10 μg/ml PMPs that it could be related to the PMPs adherence on CD34+ cells during CXCR4 transferring to CD34+ cells.
Krzyworzeka observed that, except for PAR-1, all other receptors (CD41, CD62, and CXCR4) were efficiently transferred by PMPs to normal human BFU-E-derived erythroblasts, CFU-GM-derived myeloblasts, and monocytes.
Italiano Jr et al. also observed that treated HSCs with platelet MPs express several platelet membrane receptors such as CXCR4, CD41, CD62, and PAR1 (Italiano et al. Citation2010).
In our study, we demonstrated that CD34+ cells interact with platelets and bind PMPs to their cell membranes, also the exposure of CD133+ cells isolated from cord blood to PMPs with 10 μg/ml concentration increased the expression of CXCR4 surface marker significantly that could be facilitated homing of these cells to the bone marrow. Hence, the PMPs effect on the expression of genes encoding adhesion molecules on human hematopoietic progenitor cells require further study.
Declaration of interest
The authors report no conflicts of interest. The authors alone are responsible for the content and writing of this article.
References
- Baj-Krzyworzeka M, Majka M, Pratico D, Ratajczak J, Vilaire G, Kijowski J, et al. 2002. Platelet-derived microparticles stimulate proliferation, survival, adhesion, and chemotaxis of hematopoietic cells. Exp Hematol. 30(5):450–459
- Flaumenhaft R. 2006. Formation and fate of platelet microparticles. Blood Cells Mol Dis. 36(2):182–187
- Italiano JE Jr, Mairuhu AT, Flaumenhaft R. 2010. Clinical relevance of microparticles from platelets and megakaryocytes. Curr Opin Hematol. 17(6):578–584
- Iwasaki H, Suda T. 2010. Hematopoietic stem cells and their niche. In: Kondo M, ed. Hematopoietic Stem Cell Biology, 1st ed. London: Humana Press, pp. 37–56
- Janowska-Wieczorek A, Majka M, Kijowski J, Baj-Krzyworzeka M, Reca R, Turner AR, et al. 2001. Platelet-derived microparticles bind to hematopoietic stem/progenitor cells and enhance their engraftment. Blood. 98(10):3143–3149
- Kita K, Lee JO, Finnerty CC, Herndon DN. 2011. Cord blood-derived hematopoietic stem/progenitor cells: Current challenges in engraftment, infection, and ex vivo expansion. Stem Cells Int. 27:61–93
- Nie Y, Han YC, Zou YR. 2008. CXCR4 is required for the quiescence of primitive hematopoietic cells. J Exp Med. 205(4):777–783
- Nomura S, Fukuhara S. 2004. Platelet microparticles. Methods Mol Biol. 272:269–277
- Nomura S, Ozaki Y, Ikeda Y. 2008. Function and role of microparticles in various clinical settings. Thromb Res. 123(1):8–23
- Papayannopoulou T, Priestley GV, Bonig H, Nakamoto B. 2003. The role of G-protein signaling in hematopoietic stem/progenitor cell mobilization. Blood. 101(12):4739–4747
- Rank A, Nieuwland R, Delker R, Köhler A, Toth B, Pihusch V. 2010. Cellular origin of platelet-derived microparticles in vivo. Thromb Res. 126(4):e255–259
- Siljander PR. 2011. Platelet-derived microparticles-an updated perspective. Thromb Res. 127(Suppl 2):S30–S33
- Timeus F, Crescenzio N, Basso G, Ramenghi U, Saracco P, Gabutti V. 1998. Cell adhesion molecule expression in cord blood CD34+ cells. Stem Cells. 16:120–126
- Wysoczynski M, Reca R, Ratajczak J, Kucia M, Shirvaikar N, Honczarenko M. 2005. Incorporation of CXCR4 into membrane lipid rafts primes homing-related responses of hematopoietic stem/progenitor cells to an SDF-1 gradient. Blood. 105(1):40–48