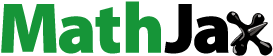
Abstract
Poly(2-hydroxyethyl methacrylate-glycidyl methacrylate) [p(HEMA-GMA)] cryogel discs were prepared under sub-zero temperatures. Gelatin was attached covalently on the p(HEMA-GMA) cryogel discs and reversible immobilization of horseradish peroxidase (HRP) was performed. The p(HEMA-GMA) cryogel discs were characterized by swelling tests, scanning electron microscopy, and surface area measurements. HRP immobilization capacity of p(HEMA-GMA)/gelatin cryogel discs was 24.8 mg/g. Removal of phenol from aqueous solutions was performed using HRP immobilized p(HEMA-GMA)/gelatin cryogel. It was observed that within 2 h of contact time, the percentage of phenol removal reaches up to 91% in the presence of H2O2.
Introduction
Phenol is highly soluble in water and is an effective disinfectant because of its germicidal effect. Phenol is very dangerous for human and marine life since it can be absorbed through the skin (Abay et al. Citation2005). Phenol and its derivatives are widely used in many petrochemical and petroleum refineries as well as chemical and pharmaceutical industries (Lin and Juang Citation2009). According to the EPA in USA, phenols and its derivatives are considered to constitute the 11th of the 126 chemicals which have been indicated as priority pollutants (Shaarani and Hameed Citation2010). Hence, removal of phenol and its derivatives from wastewater is of great importance (Aydemir and Güler Citation2015). Many technologies have been reported for degradation and removing of phenolic compounds in wastewater. They included adsorption, biodegradation, UV/Fe+3, extraction and oxidation (Dabrowski et al. Citation2005). There are many studies reported for developing processes in which peroxidase enzymes are used to remove phenolic contaminants from industrial wastewater (Ghioureliotis and Nicell Citation1999). Horse radish peroxidase (HRP) catalyses dehydrogenation of many organic compounds such as phenols, hydroquinones, hydroquinoid amines, and aromatic amines (Ashida et al. Citation2015, Shukla et al. Citation2004). However, the use of free enzymes also shows some important drawbacks including separating of enzyme from reaction medium. To overcome this drawback, enzymes have been immobilized on synthetic and natural supports (Crecchio et al. Citation1995). Enzyme immobilization provides a multi-purpose physicochemical tool that allows the reuse or continuous use of enzymes. Immobilization of enzymes also simplifies the reactor design, avoids product contamination, and, in certain cases, improves stability or tolerability (Wang et al. Citation2013). The immobilization of enzymes on different kinds of supports in heterogeneous systems is currently an active area of research in biotechnology (Soomro et al. Citation2013). Because immobilization gives additional stability to a variety of enzymes against several forms of denaturation (Akgöl et al. Citation2009). Reversible enzyme immobilization methods could be advantageous for the immobilization of many industrial enzymes (Wang et al. Citation2013). Different procedures such as adsorption to insoluble materials, encapsulation, entrapment in polymeric matrix, covalent linking to an insoluble carrier or cross linking with a bifunctional reagent have been developed for enzyme immobilization and the various supports for enzymes immobilization such as membranes, polymeric materials, hollow fibers, or microbeads (Akgöl et al. Citation2009, Akgöl and Denizli Citation2004, Soomro et al. Citation2013).
In this study, poly(2-hydroxyethyl methacrylate-glycidyl methacrylate) [p(HEMA-GMA)] cryogel disc was prepared as a support matrix. After covalently attachment of gelatin on p(HEMA-GMA) cryogel, reversible immobilization of HRP was carried out. HRP (E.C.1.11.1.7) is the most commonly used peroxidase that belongs to the group of heme proteins [9] and has wide applications for the removal of phenol. Some parameters such as gelatin and HRP enzyme concentration, pH, and temperature were also optimized for the best immobilization of HRP on p(HEMA-GMA)/gelatin cryogel.
Materials and methods
Materials
N, N′-methylene-bis(acrylamide) (MBAAm), 2-hydroxyetyhl methacrylate (HEMA), gelatin (from porcin skin, Type A), ammonium persulfate (APS), N, N, N′, N′-tetramethylenediamine (TEMED, more than 99% pure), horseradish peroxidase (hydrogen peroxide oxidoreductase – E.C. 1.11.1.7) Type I were supplied from Sigma (St. Louis, MO). Glycidyl methacrylate was purchased from Fluka (Tokyo, Japan). All other chemicals were of the highest purity and used without further purification. Ultra-pure water filtered by Millipore SAS 67120 (Millipore Inc., Molsheim, France) was used for all experiments unless otherwise stated. Before use, all the laboratory glassware were rinsed with distilled water and dried in a dust-free environment.
Preparation of p(HEMA-GMA) cryogel
Preparation of p(HEMA-GMA) cryogel discs is described below (Uygun et al. Citation2013). Briefly 1.132 g of MBAAm was dissolved in 32 mL purified water. Then, 4.8 mL of HEMA and 100 μL of GMA were dissolved in 20 mL deionized water. Then, these two solutions were mixed together. The mixture was degassed to remove the soluble oxygen under vacuum for about 5 min. The p(HEMA-GMA) cryogel disc were prepared by free radical polymerization initiated by TEMED (100 μL) and APS (80 mg). After APS was added, the mixture was stirred in an ice bath on magnetic stirrer for 2–3 min. Then, TEMED was added and stirring was continued for 30 s. The reaction mixture was poured between two glass plates separated with 1.5 mm thick spacers. The polymerization mixture was frozen at −12°C for 24 h. After the polymerization was completed, the cryogel was thawed at room temperature. The resulting cryogel plate was cut into circular pieces (0.8 cm diameter) with a perforator. The p(HEMA-GMA) cryogel discs were washed with water many times. They were stored in a buffer containing 0.02% sodium azide at 4°C until use.
Characterization studies
The swelling degree of the p(HEMA-GMA) cryogel discs (S) were determined as follows: after the preparation process, the p(HEMA-GMA) cryogel discs were washed until no monomer was detected in washing solution. Then, they were dried on a filtered paper and transferred on a clean paper. The weight of the p(HEMA-GMA) cryogel discs were determined (mwet gel). After drying in vacuum oven at 55°C, the mass of dried discs was determined (mdrygel). The swelling degree is calculated as
(1)
(1)
The total volume of supermacropores in the swollen cryogel was calculated as follows: the weight of the sample (msqueezedgel) was determined after squeezing free water from the swollen gel. The porosity was calculated as follows:
(2)
(2)
The percent of swollen gel weight was also calculated as
(3)
(3)
All measurements were done in triplicate and the average values were presented.
The bulk structure of cryogel was examined using scanning electron microscope (SEM). The p(HEMA-GMA) cryogel discs swelled in water were initially frozen at −20°C and then they were dehydrated at −50°C in lyophilizator (Lyophilizer, Christ Alpha 1–2 LD plus, Christ Alpha, Deutsch, Germany). Finally, p(HEMA-GMA) cryogel discs were coated with gold–palladium (40:60) and analyzed using a scanning electron microscope (JEOL JSM 5600, Tokyo, Japan).
Gelatin attachment on p(HEMA-GMA) cryogel
Gelatin is a complex protein and prepared by denaturation of collagen (Bailey and Paul Citation1998). Gelatin was covalently attached to the p(HEMA-GMA) cryogel discs through epoxy groups of GMA. Initially, gelatin was dissolved in 0.5 M NaOH solution to make the concentration 0.5 mg/mL. The gelatin solution in NaOH was stirred for 1 h at room temperature. Then, the p(HEMA-GMA) cryogel discs were added into the gelatin solution. The p(HEMA-GMA) cryogel discs were kept in gelatin solution for 24 h. At the end of 24 h, the p(HEMA-GMA) cryogel discs were taken out and washed with deionized water. The amount of attached gelatin was determined using the Bradford method (Bradford Citation1976)
Reversible immobilization of HRP
Reversible immobilization of HRP was performed in a batch experimental set-up. Different amounts of HRP (0.5–3.0 mg/mL) were dissolved in 5 mL pH 7.5 phosphate buffer in reaction vessels. Then, two pieces of p(HEMA-GMA)/gelatin cryogel discs were added to the reaction vessels. The immobilization process was continued for 2 h at room temperature. Also, effects of pH, temperature, and initial HRP concentration on HRP immobilization on gelatin attached p(HEMA-GMA) cryogel disc were determined. The amount of immobilized HRP was calculated from the difference between the initial and the final HRP concentration using the equation below. The method used to detect HRP amount was Bradford:
(4)
(4)
where Ci and Cf are the initial and final HRP concentrations (mg/mL), respectively, V is the volume of the HRP solution (mL) and m is the amount of p(HEMA-GMA) cryogel discs (g).
Application of phenol removal
Phenol removal capacity of HRP immobilized p(HEMA-GMA)/gelatin cryogel discs was tested. Phenol and naphthol were selected as priority pollutants as they have very noxious effects on human as well as marine life. Phenol (50 mg/L) and naphthol (50 mg/L) solutions were prepared in two separate beakers in phosphate buffer pH 7.5 in the presence of 1 mM H2O2. After adding of cryogel discs to the beaker, phenol removal process was started for 2 h. Phenol and naphthol were analyzed spectrophotometrically at 270 nm and 290 nm, respectively.
Desorption and reusability
Desorption of HRP from P(HEMA-GMA)/gelatin cryogel discs was achieved using 0.5 M NaSCN solution pH 8.0 in order to test reusability of P(HEMA-GMA)/gelatin cryogel discs. Cheap and reusable materials for enzyme immobilization are necessary especially for industrial usage. Therefore, p(HEMA-GMA)/gelatin cryogel discs were used ten times in adsorption–desorption cycle and phenol removal efficiency of HRP immobilized p(HEMA-GMA)/gelatin cryogel discs was tested in each step.
Results and discussion
Characterization
The SEM images of the internal structure of the p[HEMA-GMA]/gelatin cryogel are shown in . The p(HEMA-GMA) cryogel discs were porous and had smooth polymer walls. The p(HEMA-GMA) cryogel discs had large continuous interconnected pores (10–100 μm in diameter). The interconnected pores let the macromolecules flow through the cryogel. The advantages of macroporosity make cryogels suitable materials for the purification and immobilization of macromolecules (Tuzmen et al. Citation2012). The p(HEMA-GMA) cryogel discs were elastic and the water inside the pores could be removed squeezing by hand. In addition, when p(HEMA-GMA) cryogel discs were put in to the water again, they regained their original size and shape due to their shape memory. Specific surface area was also calculated for p(HEMA-GMA) cryogel discs according to the Brunauer–Emmett–Teller (BET) theory. The surface area of p(HEMA-GMA) cryogels discs was 9.2 m2/g ().
Table 1. Some properties of p(HEMA-GMA) cryogel discs.
Reversible immobilization of HRP
Effect of pH
Optimization of pH plays an important role in reversible immobilization studies. The effect of pH was observed by varying pH of the immobilization medium between 5.0 and 9.0 using phosphate buffer. shows HRP immobilization capacity of p(HEMA-GMA)/gelatin cryogel discs at different pH values. Maximum immobilization capacity which was 9.6 mg/g was obtained at pH 7.5. HRP immobilization capacity of p(HEMA-GMA)/gelatin cryogel discs was decreased at pH values lower and higher than pH 7.5. The results were related with suitable conformations of gelatin and HRP to interact with each other. When it is considered that both gelatin and HRP contain many kinds of amino acids in their structure, different kinds of interactions such as hydrophobic and electrostatic should play significant role between gelatin and HRP (Baily and Light Citation1989).
Effect of temperature
The effect of temperature on HRP immobilization capacity of gelatin attached p(HEMA-GMA) cryogel discs is shown in . Maximum immobilization capacity was achieved at 37°C. It was clear that immobilization of HRP increased with the increasing of temperature. When the temperature of immobilization medium was increased from 4°C to 37°C, HRP immobilization capacity increased from 7.2 mg/g to 15.5 mg/g. As temperature increases, entropy increases and makes contribution to hydrophobic interactions. This result supported that the molecular interactions between gelatin and HRP were mostly hydrophobic. Since HRP is a glycoprotein and contains 18% carbohydrate, it is expected that most of the interactions between gelatin and HRP are hydrophobic.
Effect of initial HRP concentration
Enzymes are the biological catalysts and enzymatic reactions are dependent on the amount of enzyme (Soomro et al. Citation2013). The effect of initial HRP concentration on HRP immobilization capacity of p(HEMA-GMA)/gelatin cryogel discs is presented in . Maximum amount of HRP immobilized on p(HEMA-GMA)/gelatin cryogel discs was 24.8 mg/g. It was shown that after 2 mg/ml HRP concentration, it was not meaningful to load more HRP to the immobilization medium. In addition, it was not economical to immobilize more ligand than the capacity of adsorbent. Therefore, we have chosen 2 mg/ml as optimized initial HRP concentration. HRP immobilization capacity of p(HEMA-GMA) cryogel discs was also determined and the results are shown in . Immobilization capacity of p(HEMA-GMA) cryogel discs was very low. Therefore, non-specific immobilization of HRP on p(HEMA-GMA) matrix does not occur and affinity between HRP and gelatin is specific.
Figure 4. Effect of initial HRP concentration on immobilization of HRP. Gelatin loading: 65.3 mg/g; pH: 7.5; T: 25°C.
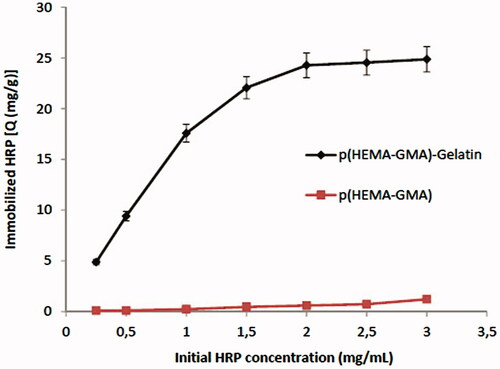
HRP adsorption from aqueous solutions was applied for Langmuir and Freundlich adsorption isotherms (Le Van and Vermeulen Citation1981). Langmuir adsorption isotherm can be defined as Q = QmaxbCe/(1+bCe). Here, Q is the actual HRP adsorption capacity (mg/g), Ce is the equilibrium HRP concentration (mg/mL), and b is the Langmuir adsorption equilibrium constant (mL/mg), which indicates monolayer adsorption. Freundlich adsorption isotherm is explained as Q = QFCe1/n. Here QF is the Freundlich adsorption constant, Ce is the equilibrium HRP concentration (mg/mL), and n is the Freundlich exponent.
According to the adsorption isotherm results shown in , HRP adsorption on the surface of p(HEMA-GMA)/gelatin cryogel discs fitted very well with Langmuir isotherm. When correlation coefficients values were compared, it was clear that the correlation coefficient value for Langmuir isotherm (R2 = 0.99) was higher than the correlation coefficient value for Freundlich isotherm (R2 = 0.93). As a result, HRP adsorption on p(HEMA-GMA)/gelatin cryogel discs was monolayer. In addition, “b” is a coefficient related to the affinity between the analyte and the ligand. The value of “b” is 0.46 in our system and it indicates an affinity between HRP and gelatin.
Table 2. Langmuir and Freundlich adsorption isotherm constants for reversible immobilization of HRP.
In order to determine mechanisms controlling adsorption process such as mass transfer and/or chemical reaction, first- and second-order kinetic models were applied to the experimental data (Cheung et al. Citation2001). The pseudo-first-order rate of Lagergren equation which is defined in EquationEquation (5)(5)
(5) is usually used for the adsorption of solute from a liquid solution
(5)
(5)
where qe is the experimental amount of HRP adsorbed at equilibrium (mg/g); qt is the amount of HRP adsorbed at time t (mg/g); k1 is the rate constant of the pseudo-first-order adsorption (min−1). A plot of log (qeq−qt) versus t should give a straight line to confirm the applicability of the first-order kinetic model.
The pseudo-second-order kinetic model is defined in the following equation:
(6)
(6)
where k2 is the rate constant of the pseudo-second-order adsorption (g mg−1 min−1). If the pseudo-second-order kinetics is applicable, the plot of t/q versus t should be linear.
The results derived from the first- and second-order kinetic models are given in . The correlation coefficient was 0.99 in pseudo-second-order kinetic model. As a result, the pseudo-second-order kinetic model was suitable for the adsorption of HRP on p(HEMA-GMA)/gelatin cryogel discs and the adsorption was a chemical adsorption. Immobilization of HRP on p(HEMA-GMA)/gelatin controls the kinetic behavior.
Table 3. The first- and second-order kinetic constants.
Application of phenol removal
To check the capability of novel cryogel based material, the removal of phenol has been performed. Reaction medium contained 10 mg/l of phenol and 1 mM of H2O2 as oxidant. p(HEMA-GMA)/gelatin cryogel discs were treated with the reaction medium at room temperature. It was observed that the HRP-immobilized p[HEMA-GMA]/gelatin cryogel discs showed up to 91% removal within 2 h of contact time. So we further concluded that the maximum removal of <90% can be achieved by increasing the amount of HRP immobilized p(HEMA-GMA)/gelatin cryogel discs and H2O2 concentration in the reaction medium.
Desorption and reusability
Adsorption–desorption cycle was repeated ten times and the results are presented in . Phenol removal capacity of HRP immobilized p(HEMA-GMA)/gelatin cryogel discs is also shown in . Immobilization of HRP on p(HEMA-GMA)/gelatin cryogel discs was decreased less than 10% after 10 adsorption–desorption cycles. It was also obvious that phenol removal capacity of HRP immobilized p(HEMA-GMA)/gelatin cryogel discs was 80% after 10 cycles. The phenol removal capacity was decreased only 12%. Reusable HRP immobilized p(HEMA-GMA)/gelatin cryogel discs are promising materials for phenol removal in industry.
Conclusion
Cryogels are known as novel generation materials for the biosorption of toxic substances (Koç et al. Citation2011). The p(HEMA-GMA)/gelatin cryogel discs have been used for reversible immobilization of HRP for the purpose of phenol removal. p(HEMA-GMA) cryogel discs are cheap materials and because of their large pores they are suitable for the immobilization of macromolecules such as enzymes. Cheapness of p(HEMA-GMA) cryogel discs can compensate for high prices of immobilized HRP. In addition, immobilization of HRP through gelatin molecules makes the material more stable. HRP immobilized p(HEMA-GMA)/gelatin cryogel dics presented here can be a model system for phenol removal from industrial wastewater streams.
Declaration of interest
Rabel Soomro is grateful to the financial support provided by the Turkish Science and Technology Research Council (TUBITAK-BIDEB).
References
- Abay I, Denizli A, Biskin E, Salih B. 2005. Removal and pre-concentration of phenolic species onto b-cyclodextrin modified poly(hydroxyethylmethacrylate-ethyleneglycoldimethacrylate) microbeads. Chemosphere. 61:1263–1272.
- Akgöl S, Denizli A. 2004. Novel metal-chelate affinity sorbents for reversible use in catalase adsorption. J Mol Catal B: Enzym. 28:7–14.
- Akgöl S, Ozturk N, Denizli A. 2009. New generation polymeric nanospheres for catalase immobilization. J Appl Polym Sci. 114:962–970.
- Ashida T, Sakai S, Taya M. 2015. Propagation of human iPS cells in alginate-based microcapsules prepared using reactions catalyzed by horseradish peroxidase and catalase. Artif Cells Nanomed Biotechnol. DOI:https://doi.org/10.3109/21691401.2015.1029631.
- Aydemir T, Güler S. 2015. Characterization and immobilization of Trametes versicolor laccase on magnetic chitosan–clay composite beads for phenol removal. Artif Cells Nanomed Biotechnol. 43(6):425–432.
- Bailey AJ, Paul RG. 1998. Collagen: a not so simple protein. J Soc Leath Tech Ch. 82:104–110.
- Baily AJ, Light ND. 1989. Connective Tissue in Meat and Meat Products. London and New York: Elsevier Applied Science.
- Bradford MM. 1976. Rapid and sensitive method for the quantitation of microgram quantities of protein utilizing the principle of protein-dye binding. Anal Biochem. 72:248–254.
- Cheung CW, Porter JF, McKay G. 2001. Sorption kinetic analysis for the removal of cadmium ions from effluents using bone char. Water Res. 35:605–612.
- Crecchio C, Ruggiero P, Pizzigallo MDR. 1995. Polyphenoloxidases immobilized in organic gels: properties and detoxification of aromatic compounds. Biotechnol Bioeng. 48:585–591.
- Dabrowski A, Podkościelny P, Hubicki Z, Barczak M. 2005. Adsorption of phenolic compounds by activated carbon – a critical review. Chemosphere. 58:1049–1070.
- Ghioureliotis M, Nicell JA. 1999. Assessment of soluble products of peroxidase catalyzed polymerization of aqueous phenol. Enzyme Microb Tech. 25:185–193.
- Koç İ, Baydemir G, Bayram E, Yavuz H, Denizli A. 2011. Selective removal of 17β-estradiol with molecularly imprinted particle-embedded cryogel systems. J Hazard Mater. 192:1819–1826.
- Le Van MD, Vermeulen T. 1981. Binary Langmuir and Freundlich isotherms for ideal adsorbed solutions. J Phys Chem. 85:3247–3250.
- Lin SH, Juang RS. 2009. Adsorption of phenol and its derivatives from water using synthetic resins and low-cost natural adsorbents: a review. J Environ Manage. 90:1336–1349.
- Shaarani FW, Hameed BH. 2010. Batch adsorption of 2,4-dichlorophenol onto activated carbon derived from agricultural waste. Desalination. 255:159–164.
- Shukla SP, Mod K, Ghosh PK, Devi S. 2004. Immobilization of horseradish peroxidase by entrapment in natural polysaccharide. J Appl Polym Sci. 91:2063–2071.
- Soomro R, Memon N, Bhanger MI, Samoon MK. 2013. Microemulsion based organogel-silica composite as feasible matrix for immobilization of horseradish peroxidase: application to oxidative removal of phenol from aqueous system. J Chem Soc Pak. 35:938–946.
- Tuzmen N, Kalburcu T, Denizli A. 2012. Immobilization of catalase via adsorption onto metal-chelated affinity cryogels. Process Biochem. 47:26–33.
- Uygun M, Akduman B, Akgol S, Denizli A. 2013. A new metal-chelated cryogel for reversible immobilization of urease. Appl Biochem Biotech. 170:1815–1826.
- Wang J, Zhao G, Li Y, Liu X, Hou P. 2013. Reversible immobilization of glucoamylase onto magnetic chitosan nanocarriers. Appl Microbiol Biotechnol. 97:681–692.