Abstract
Various microorganisms were found to be cable of synthesizing gold and silver nanoparticles when gold and silver salts were supplied in the reaction system. The main objective of this study was to evaluate the extracellular synthesis of gold and silver nanoparticles by the type strain Microbacterium resistensT [KACC14505]. The biosynthesized gold and silver nanoparticles were characterized by ultraviolet–visible spectroscopy (UV–Vis), field emission transmission electron micrograph (FE-TEM), energy dispersive X-ray spectroscopy (EDX), elemental mapping, and dynamic light scattering (DLS). Moreover, the nanoparticles were evaluated for antimicrobial potential against various pathogenic microorganisms such as Vibrio parahaemolyticus [ATCC 33844], Salmonella enterica [ATCC 13076], Staphylococcus aureus [ATCC 6538], Bacillus anthracis [NCTC 10340], Bacillus cereus [ATCC 14579], Escherichia coli [ATCC 10798], and Candida albicans [KACC 30062]. The silver nanoparticles were found as a potent antimicrobial agent whereas gold nanoparticles not showed any ability. Therefore, the current study describes the simple, green, and extracellular synthesis of gold and silver nanoparticles by the type strain Microbacterium resistensT [KACC14505].
Introduction
Metal nanoparticles have abundant significance due to their unique electronic, optical, and physio-chemical properties which differ significantly from those of bulk materials (Mazur Citation2004). Gold and silver nanoparticles with large surface area, quantum effect, and small size effect exhibit excellent accomplishment in diverse fields, such as optics, optoelectronics, drug delivery, gene therapy, catalysis, and imaging (Biswas et al. Citation2004). The properties of nanoparticles make them dissimilar correspond to the tunable optical, physical, and chemical properties (Khan et al. Citation2014, Singh et al. Citation2015a). The gold nanoparticles have extensive application in the biosensors designing, photochemical, and electrochemical catalysis (Yáñez-Sedeño and Pingarrón 2005). Gold nanoparticles have been applied in various medicinal applications for instance, targeted drug delivery, imaging, diagnosis, photothermal therapy, and therapeutics. In addition, the particles show antimicrobial potential against human pathogenic bacteria and fungi (Piruthiviraj et al. Citation2015). The silver nanoparticles are also well known for various medicinal purpose including antimicrobial, antibiofilm, anti-inflammatory, and anticancer activity, out of which the antimicrobial properties are well known. The antimicrobial characteristics of silver nanoparticles have been exploited well and utilized in various processes in the medical and clinical background for the treatment against many pathogenic and multi-drug resistant microorganisms (Prabhu and Poulose Citation2012). Reports suggest that silver nanoparticles have broad-spectrum sterilization and strong bactericidal effect against many multi-drug resistant microorganisms, the particles can kill more than 650 kinds of bacteria in a few minutes (Nagasundaram et al. Citation2014). Corresponds to the antimicrobial potential, the use of silver nanoparticles has been commercialized in many industrial products such as for wound dressing, medical catheter coverings, hang gel, and cavity filler (Chaloupka et al. Citation2010).
Recently, the synthesis of gold and silver nanoparticles using biological system has been highlighted, which is non-toxic, safe, green, and economical, paves a new way to improve the synthesis methodology (Parikh et al. Citation2008, Singh et al. Citation2015e). Microorganisms such as bacteria, yeast, fungi, and actinomycetes are commonly used for biosynthesis of nanoparticles as biogenic agents (Salunkhe and Nayak Citation2012, Singh et al. Citation2015b,Citationc, Mukherjee et al. Citation2002). For instance, extracellular biosynthesis of silver nanoparticles has been conducted using Escherichia coli, Bacillius sp., B. frigoritolerans and Morganella sp. (Gurunathan et al. Citation2009, Reddy et al. Citation2010, Singh et al. Citation2015c). Additionally, gold nanoparticles were successfully synthesized using Thermus aquaticus, B. indica, and several plant extracts by biological route (Singh et al. Citation2015b–e). Consequently, since the green synthesis methods are environment benign, the present study focus on the extracellular synthesis of gold and silver nanoparticles using type strain Microbacterium resistensT [KACC14505].
Materials and methods
Media and chemicals
Microbacterium resistensT [KACC14505] was ordered form Korean Agricultural Culture Collection (KACC). Gold (III) chloride trihydrate (HAuCl4.3H2O), silver nitrate (AgNO3), and cycloheximide were purchased from Sigma-Aldrich Chemicals (St. Louis, MO), which were of analytical grade. All the media were purchased from the Difco, MB cell, Seoul, Republic of Korea. Penicillin G (P10) 10 μg/disk, oleandomycin (OL15) 15 μg/disk, and lincomycin (MY15) 15 μg/disk were purchased from Oxoid Ltd., England, UK. The pathogenic bacterial strains used were Vibrio parahaemolyticus [ATCC 33844], Salmonella enterica [ATCC 13076], Staphylococcus aureus [ATCC 6538], Bacillus anthracis [NCTC 10340], Bacillus cereus [ATCC 14579], Escherichia coli [ATCC 10798], and Candida albicans [KACC 30062]. The strains were cultured in Tryptic soy agar (TSA) media at 30 °C and preserved at −70 °C in glycerol stock vials for further study. Candida albicans was cultured on sabouraud dextrose agar (SDA) at 28 °C and preserved at −70 °C in glucose yeast peptone broth (GYP) glycerol stock vial.
Biosynthesis of gold and silver nanoparticles
For the synthesis of gold and silver nanoparticles, the bacterial isolate was inoculated in sterile tryptic soy broth (TSB) 100 ml and incubated in a shaking incubator at 30 °C for 24 h at 180 rpm. After incubation, the cell culture medium was centrifuged at 6000 rpm for 10 min to separate the bacterial pellet. The supernatant was collected and used for synthesis of nanoparticles. Filter-sterilized gold (III) chloride trihydrate (HAuCl4.3H2O) solution at 1 mM final concentration was added in the collected supernatant and incubated in shaker at 28 °C and 200 rpm. While incubation the reaction mixture was detected by visual observation of color change. For the collection and purification of gold nanoparticles, the incubated mixture was first centrifuged at low speed range from (3000–6000 rpm) for 5 min each, to remove the unwanted components. Then, the nanoparticles were obtained by high-speed centrifugation at 15,000 rpm for 15 min. The gold nanoparticles were further purified by thoroughly washing with sterile water to remove the water soluble impurities. Finally, the product was collected as a pellet after centrifugation, air dried, and used for further characterization.
Similarly, the silver nanoparticles synthesized by using AgNO3, at 1 mM final concentration into the cultured supernatant, further, after the incubation period, the silver nanoparticles were collected and purified by repeated centrifugation cycles and washing by sterile water and lastly collected in pellet form for characterization.
Characterization of nanoparticles
To verify the reduction of gold and silver ions, each culture solution was scanned at 300–800 nm in a UV–Vis spectrophotometer (Ultrospec 2100 pro, Amersham Biosciences Corp., Piscataway, NJ). The particles were further analyzed by using field emission transmission electron microscopy (FE-TEM), energy dispersive X-ray spectroscopy (EDX), and elemental mapping with a JEM-2100F (JEOL, Tokyo, Japan) instrument, operated at 200 kV. Sample preparation for FE-TEM has been done by placing a drop of gold and silver nanoparticles on a carbon-coated copper grid and subsequently drying it in an oven at 60 °C, before transferring it to the microscope (Singh et al. Citation2015c). The dynamic light scattering (DLS, Photal, Otsuka Electronics, Osaka, Japan) was used to monitor the particles size distribution (Singh et al. Citation2015d).
Analysis of antimicrobial activity of nanoparticles
The disk diffusion method was followed for the analysis of antimicrobial activity of the synthesized nanoparticles against pathogenic microorganisms such as V. parahaemolyticus, S. enterica, S. aureus, B. anthracis, B. cereus, E. coli, and C. albicans. In this assay, 100 μl of overnight log culture of each test pathogenic microorganisms were spread evenly on a Mueller–Hinton agar (MHA) plate. The antibiotics discs P10, OL15, and MY15 were kept on the plate as a control, the sterile paper discs were impregnated with 30 μl (100 mg/l) gold and silver nanoparticles solution and placed onto the MHA plates, and further incubated at 30 °C for 24 h. For C. albicans, overnight log culture of C. albicans was spread evenly on a SDA plate and the sterile paper discs impregnated with nanoparticles solution 30 μl (100 mg/l), cycloheximide were maintained as control. The plates were further kept at 25 °C for 24 h incubation. After incubation, the zones of inhibition around the disk were measured in all the plates (Singh et al. Citation2015b, Citationc).
Results and discussion
Bacteria Identification
Microbacterium resistensT [KACC14505] was ordered form Korean Agricultural Culture Collection (KACC). Microbacterium is a genus of bacteria in the family Microbacteriaceae which was defined by Orla-Jensen in 1919. As of 2015, it consists of 92 species (www.bacterio.net). The applications of Microbacterium sp. have been reported in different fields such as bioemulsifier production (Aniszewski et al. Citation2010), and bioconversion. For instance, Microbacterium sp. GS514 was isolated for ginseng field soil, which is the first report production of ginsenoside Rg3 by using microbial enzymes (Cheng et al. Citation2008). Recently, Microacterium sp. MRS-1 has been reported for the synthesis of nickel oxide nanoparticles through extracellular way (Sathyavathi et al. Citation2014). The present study describes the use of type strain Microbacterium resistensT [KACC14505] for extracellular synthesis of gold and silver nanoparticles.
Synthesis and characterization of gold and silver nanoparticles
Many ecofriendly ways have been focused for the synthesis of metal nanoparticles. Previously, silver and gold nanoparticles were successfully synthesized using plant extract, bacteria and fungi etc. has been reported (Ahmed et al. Citation2014, Reddy et al. Citation2010, Singh et al. Citation2015b–e, Sunkar and Nachiyar Citation2012, Wang et al. Citation2015). The present study describes M. resistensT for the synthesis of gold and silver nanoparticles in the cell culture supernatant. The synthesis of gold and silver nanoparticles in the supernatant of M. resistensT was monitored by the color change in the reaction mixture during the incubation period. In case of gold nanoparticle, the color changed gradually from yellow to crimson within 10 h (), whereas the control flask remains same, no change in color (). Similarly, for silver nanoparticle, reaction mixture turned from yellow to brown in 48 h (). The color changed in the reaction mixture was due to the excitation of surface plasmon vibrations due to the formation of gold and silver nanoparticles in the relevant reaction mixtures (Anil Kumar et al. Citation2007, Singh et al. Citation2015e). The mechanism of biosynthesis of gold and silver nanoparticles is still in the stage of preliminary inquiry, but the studies suggest that the extracellular enzymes secreted by microorganism are important part for the synthesis (Hulkoti and Taranath Citation2014). Additionally, it has been concluded that microorganisms growing up in a complex environment which leads to the release of many proteins and enzymes, are responsible for the reduction of gold and silver ions, as well as provide additional stability to the nanoparticles (Mukherjee et al. Citation2002). Similarly, the cells free from supernatant of M. resistensT contain many enzyme and proteins secreted by bacteria extracellulary that might have been involved in the fabrication of nanoparticles. Recently, many reports demonstrate the plants and microorganism for this potential, for illustration, fresh leaf extract of Morinda citrifolia (Pai et al. Citation2015), medicinal plant Clerodendron serratum (Priyadharshini Raman et al. Citation2015), Solanum indicum L.(Sengottaiyan et al. Citation2015), microorganism involving Pseudomonas deceptionensis DC5 (Jo et al. Citation2015), Weissella oryzae DC6 (Singh et al. Citation2015f), and fungus Aspergillus sp (Prabavathy et al. Citation2015) has been used for the synthesis of silver and gold nanoparticles. The nanoparticles synthesis was further confirmed by UV–Vis spectral analysis. The gold and silver nanoparticles reaction mixture was scanned at 300–800 nm at different time intervals and the maximum absorption peaks was observed at 568 nm and 416 nm, respectively. As per the previous studies, the obtained peak corresponds to the surface plasmon resonance of gold and silver nanoparticles, respectively (Singh et al. Citation2015e) (). Thus, the reaction mixture indicated the formation of gold and silver nanoparticles. The FE-TEM images results showed a clear morphology of obtained nanoparticles (Joseph and Mathew Citation2014). The gold nanoparticles synthesized in our study were 10–20 nm in size with spherical shaped (). For the silver nanoparticles, the results attained were spherical shape with sizes range from 10 to 20 nm (). Prior studies similarly configured the shape and size of synthesized gold and silver nanoparticles (Kalabegishvili et al. Citation2013, Singh et al. Citation2015c).
Figure 1. Reaction mixture after incubation period containing gold nanoparticles (a), control (b) and containing silver nanoparticles (c). UV–Vis spectra of the reaction mixture of gold nanoparticles (d), reaction mixture of silver nanoparticles (e).
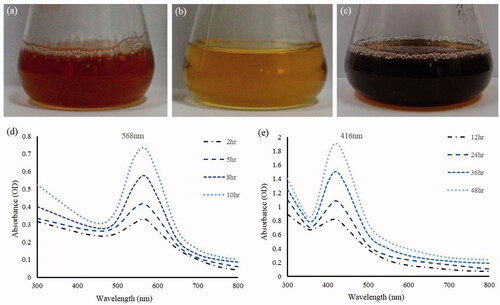
Figure 2. TEM image of gold nanoparticles at 10 nm (a), at 20 nm (b), silver nanoparticles at 10 nm (c), at 20 nm (d).
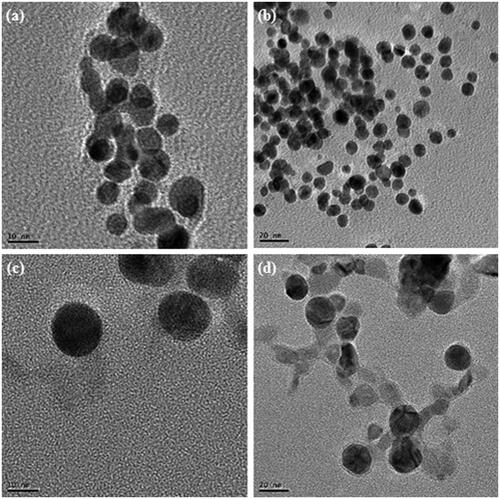
The energy dispersive X-ray analysis of gold nanocrystallites showed an optical absorption band peak at approximately 2.2 keV (), which is the typical absorption of metallic gold nanocrystallites (Elavazhagan and Arunachalam Citation2011). Elemental mapping analysis of the gold nanoparticles showed extreme distribution of metallic gold in the scanned electron micrograph image of gold nanoparticles pellet solution (). For the silver nanoparticles, in the EDX spectrum, the strong peak at 3 keV was observed () (Magudapathy et al. Citation2001). Elemental mapping analysis of the silver nanoparticles showed extreme distribution of metallic silver in the electron micrograph region (). The dynamic light scattering results showed the particle size distribution of gold and silver nanoparticles according to intensity, volume, and number of nanoparticles ().
Figure 3. EDX spectrum of gold nanoparticles (a), elemental mapping: electron micrograph region of gold nanoparticles (b), distribution of gold element (c). EDX spectrum of silver nanoparticles (d), elemental mapping: electron micrograph region of silver nanoparticles (e), distribution of silver element (f).
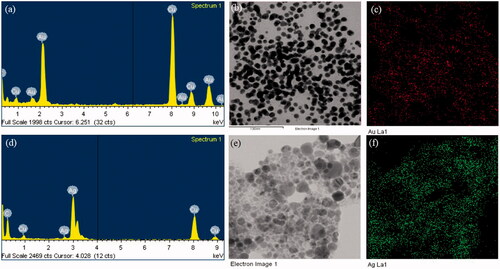
Figure 4. Particles size distribution of gold nanoparticles according to intensity (a), number (b), and volume (c); and silver nanoparticles according to intensity (d), number (e), and volume (f).
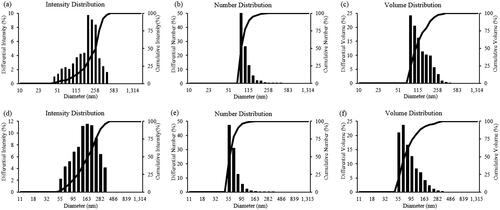
Overall, based on our results, we concluded that the strain M. resistensT is capable for efficient synthesis of gold and silver nanoparticles. Previously, Microbacterium sp. has been revealed for the activity in glycerol ester hydrolase (Park et al. Citation2014), antioxidant (Asker et al. Citation2009) and bioemulsifier (Aniszewski et al. Citation2010). To our awareness, this is first time M. resistensT was found to be proficient for synthesizing gold and silver nanoparticles. Additionally, the nanoparticles were synthesised extracellularly in the culture medium, which completely elude the downstream processing, a major necessity of intracellular synthesis.
Antimicrobial activity of nanoparticles
Silver has been used for many years for antimicrobial applications in the various fields against a range of pathogenic bacteria. As recently reported, pathogenic bacteria are becoming more resistant to antibiotics (Kotakadi et al. Citation2015), so the studies of silver nanoparticles against multi-drug-resistant micro-organisms play a crucial role. Recently, silver nanoparticles are reported for antimicrobial potential against many pathogenic microorganisms, for instance; Staphylococcus sp., Klebsiella sp., Erwinia carotovora pv. carotovora, and Alternaria solani (Abbas et al. Citation2015; Sengottaiyan et al. Citation2015; Swamy et al. Citation2015). Tran et al. (Citation2015) showed the application of silver nanoparticles in medical background by applying on medical devices, where silver nanoparticles drastically lower the pathogenic bacterial count. Other reports also describe the antimicrobial activity of silver nanoparticles against many human pathogenic and multi drug resistant microorganisms like MRSA and MRSE (Naqvi et al. Citation2013, Saravanan and Nanda Citation2010). Considering the developing resistant mechanism of the present micro-organism, in our study, gold and silver nanoparticles were tested for antimicrobial activity against a range of pathogenic micro-organisms which included V. parahaemolyticus, S. enterica, S. aureus, B. anthracis, B. cereus, E. coli, and C. albicans. For measuring the antimicrobial activity, after incubation, the mean diameter of the zone of inhibition was determined. shows the results of antimicrobial activities of synthesized gold and silver nanoparticles as compared with different standard antibiotics (). The strains V. parahaemolyticus was slight sensitivity to oleandomycin and lincomycin, but completely resistant to Penicillin G. The silver nanoparticles are well active and showed the clear inhibition of zone against V. parahaemolyticus. The strains S. enterica and S. aureus are resistant to oleandomycin and lincomycin, but showed slight sensitivity to Penicillin G, as compared with antibiotics silver nanoparticles exhibit clear and immense zone of inhibition around the disk. Other strains, B. anthracis and B. cereus showed sensitivity to both antibiotics and silver nanoparticles as well. E. coli was completely resistant to the antibiotics but sensitive to the silver nanoparticles. Similarly, C. albicans, also persist resistant to the cycloheximide, but showed sensitive zone of inhibition against silver nanoparticles. Against all the pathogenic organisms, gold nanoparticles not showed any sensitivity in the tested concentration, thus, the results clearly demonstrate that the pathogenic organism are killed successfully by silver nanoparticles, whereas, the gold nanoparticles have no influence on it.
Figure 5. Antimicrobial activity of nanoparticles in comparison with antibiotics against V. parahaemolyticus, S. enterica, S. aureus, B.anthracis, B.cereus, E. coli, and C. albicans. Note: P10, penicillin G 10 μg/disk; OL15, oleandomycin 15 μg/disk; MY15, lincomycin 15 μg/disk; cycloheximide, 10 μg/disk; AuNPs, gold nanoparticles, 30 μl (100 mg/l); AgNPs, silver nanoparticles, 30 μl (100 mg/l).
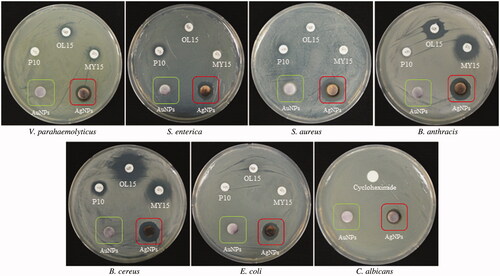
Table I. Antimicrobial activity of nanoparticles against V. parahaemolyticus, S. enterica, S. aureus, B. anthracis, B. cereus, E. coli, and C. albicans.
Thus, we synthesized the nanoparticles efficaciously by using Microbacterium resistensT which can be applied for various antimicrobial, clinical and therapeutic applications in medical biology.
Conclusions
In our study, gold and silver nanoparticles were successfully synthesized by cell filtrate of Microbacterium resistensT. The methodology is effective, simple, safe, low cost, and reduces emissions of toxic substances as compared to physical and chemical methods. The spherical silver and gold nanoparticles were obtained. Furthermore, the synthesis was extracellular which could be easily adapted to large-scale production. Additionally, the silver nanoparticles exert remarkable antimicrobial activity against human pathogenic strain V. parahaemolyticus, S. enterica, S. aureus, B. anthracis, B. cereus, E. coli, and C. albicans. Thus, the strain Microbacterium resistensT is a capable of synthesizing extracellular gold and silver nanoparticles by green and economical manner.
Declaration of interest
The authors report that they have no conflicts of interest. The authors alone are responsible for the content and writing of the paper. This research was supported by the Korea Institute of Planning & Evaluation for Technology in Food, Agriculture, Forestry & Fisheries (KIPET NO: 313038-03-2-SB010), Republic of Korea.
References
- Abbas A, Naz SS, Syed SA. 2015. Antimicrobial activity of silver nanoparticles (AgNPs) against Erwinia carotovora pv. Carotovora and Alternaria solani. Int J Biosci. 6:9–14.
- Ahmed KB, Kalla D, Uppuluri KB, Anbazhagan V. 2014. Green synthesis of silver and gold nanoparticles employing levan, a biopolymer from Acetobacter xylinum NCIM 2526, as a reducing agent and capping agent. Carbohydr Polym. 112:539–545.
- Anil Kumar S, Abyaneh M, Gosavi SW, Kulkarni S, Pasricha R, Ahmad A, Khan MI. 2007. Nitrate reductase-mediated synthesis of silver nanoparticles from AgNO3. Biotechnol Lett. 29:439–445.
- Aniszewski E, Peixoto RS, Mota FF, Leite SGF, Rosado AS. 2010. Bioemulsifier production by Microbacterium sp. strains isolated from mangrove and their application to remove cadmium and zinc from hazardous industrial residue. Braz J Microbiol. 41:235–245.
- Asker MMS, Ahmed YM, Ramadan MF. 2009. Chemical characteristics and antioxidant activity of exopolysaccharide fractions from Microbacterium terregens. Carbohydr Polym. 77:563–567.
- Avalos A, Haza AI, Drosopoulou E, Mavragani-Tsipidou P, Morales P. 2015. In vivo genotoxicity assesment of silver nanoparticles of different sizes by the Somatic Mutation and Recombination Test (SMART) on Drosophila. Food Chem Toxicol. [Epub ahead of print]. DOI:https://doi.org/10.1016/j.fct.2015.06.024.
- Biswas A, Aktas O, Sch Rmann U, Saeed U, Zaporojtchenko V, Faupel F, Strunskus T. 2004. Tunable multiple plasmon resonance wavelengths response from multicomponent polymer–metal nanocomposite systems. Appl Phys Lett. 84:2655–2657.
- Chaloupka K, Malam Y, Seifalian AM. 2010. Nanosilver as a new generation of nanoproduct in biomedical applications. Trends Biotechnol. 28:580–588.
- Cheng LQ, NA JR, Bang MH, Kim MK, Yang DC. 2008. Conversion of major ginsenoside Rb1 to 20(S)-ginsenoside Rg3 by Microbacterium sp. GS514. Phytochemistry. 69:218–224.
- Elavazhagan T, Arunachalam KD. 2011. Memecylon edule leaf extract mediated green synthesis of silver and gold nanoparticles. Int J Nanomed. 6:1265–1278.
- Gurunathan S, Kalishwaralal K, Vaidyanathan R, Venkataraman D, Pandian SR, Muniyandi J, Hariharan N, Eom SH. 2009. Biosynthesis, purification and characterization of silver nanoparticles using Escherichia coli. Colloids Surf B Biointerfaces. 74:328–335.
- Hulkoti NI, Taranath TC. 2014. Biosynthesis of nanoparticles using microbes – a review. Colloids Surf B Biointerfaces. 121:474–483.
- Jo JH, Singh P, Kim YJ, Wang C, Mathiyalagan R, Jin CG, Yang DC. 2015. Pseudomonas deceptionensis DC5-mediated synthesis of extracellular silver nanoparticles. Artif Cells Nanomed Biotechnol. [Epub ahead of print]. DOI:https://doi.org/10.3109/21691401.2015.1068792.
- Joseph S, Mathew B. 2014. Microwave assisted facile green synthesis of silver and gold nanocatalysts using the leaf extract of Aerva lanata. Spectrochim Acta A Mol Biomol Spectrosc. 136PC:1371–1379.
- Kalabegishvili TL, Murusidze IG, Kirkesali EI, Rcheulishvili AN, Ginturi EN, Gelagustashvili ES, et al. 2013. Development of biotechnology for microbial synthesis of gold and silver nanoparticles. J Life Sci. (El Monte, CA, US). 7:110–122.
- Khan AK, Rashid R, Murtaza G, Zahra A. 2014. Gold nanoparticles: synthesis and applications in drug delivery. Trop J Pharm Res. 13:1169–1177.
- Kotakadi VS, Gaddam SA, Venkata SK, Prasad TNVKV, Sai Gopal DVR. 2015. Ficus fruit-mediated biosynthesis of silver nanoparticles and their antibacterial activity against antibiotic resistant E. coli strains. Curr Nanosci. 11:527–538.
- Magudapathy P, Gangopadhyay P, Panigrahi BK, Nair KGM, Dhara S. 2001. Electrical transport studies of Ag nanoclusters embedded in glass matrix. Phys B: Condens Matter. 299:142–146.
- Mazur M. 2004. Electrochemically prepared silver nanoflakes and nanowires. Electrochem Commun. 6:400–403.
- Mukherjee P, Senapati S, Mandal D, Ahmad A, Khan MI, Kumar R, Sastry M. 2002. Extracellular synthesis of gold nanoparticles by the fungus Fusarium oxysporum. ChemBioChem. 3:461–463.
- Nagasundaram N, Rahuman MA, Raghavan PS. 2014. Antibacterial application studies of nanosilver incorporated products. Int J Pharm Res Bio – Sci. 3:153–164.
- Naqvi SZ, Kiran U, Ali MI, Jamal A, Hameed A, Ahmed S, Ali N. 2013. Combined efficacy of biologically synthesized silver nanoparticles and different antibiotics against multidrug-resistant bacteria. Int J Nanomed. 8:3187–3195.
- Pai AR, Kavitha S, Shweta RS, Priyanka P, Vrinda A, Vivin TS, Sasidharan S. 2015. Green synthesis and characterizations of silver nanoparticles using fresh leaf extract of Morinda citrifolia and its anti-microbial activity studies. Int J Pharm Pharm Sci. 7:459–461.
- Parikh RY, Singh S, Prasad B, Patole MS, Sastry M, Shouche YS. 2008. Extracellular synthesis of crystalline silver nanoparticles and molecular evidence of silver resistance from Morganella sp.: towards understanding biochemical synthesis mechanism. ChemBioChem. 9:1415–1422.
- Park HY, Son GH, Kim DY, Shin DH, Cho HY. 2014. Alkali-resistant glycoside hydrolase family 10 xylanase from Microbacterium sp. HY-17. Copyright (C) 2015 American Chemical Society (ACS). All Rights Reserved.
- Piruthiviraj P, Margret A, Krishnamurthy PP. 2015. Gold nanoparticles synthesized by Brassica oleracea (Broccoli) acting as antimicrobial agents against human pathogenic bacteria and fungi. Appl Nanosci. [Epub ahead of print]. DOI:https://doi.org/10.1007/s13204-015-0460-4.
- Prabavathy D, Niveditha R, Vaishnavie R. 2015. Antimicrobial activity of silver nanoparticles synthesized by endophytic Aspergillus sp isolated from Justicia beddomei. J Chem Pharm Res. 7:784–788.
- Prabhu S, Poulose E. 2012. Silver nanoparticles: mechanism of antimicrobial action, synthesis, medical applications, and toxicity effects. Int Nano Lett. 2:1–10.
- Priyadharshini RR, Parthiban S, Srinithya B, Vinod KV, Philip AS, Sivasubramanian A, Sundaram MM. 2015. Biogenic silver nanoparticles synthesis using the extract of the medicinal plant Clerodendron serratum and its in-vitro antiproliferative activity. Mater Lett. 160:400–403.
- Reddy AS, Chen CY, Chen CC, Jean JS, Chen HR, Tseng MJ, Fan CW, Wang JC. 2010. Biological synthesis of gold and silver nanoparticles mediated by the bacteria Bacillus subtilis. J Nanosci Nanotechnol. 10:6567–6574.
- Salunkhe PR, Nayak SS. 2012. Microbial synthesis of silver nanoparticles and their applications in medical textiles. Man-Made Text India. 40:125–128.
- Saravanan M, Nanda A. 2010. Extracellular synthesis of silver bionanoparticles from Aspergillus clavatus and its antimicrobial activity against MRSA and MRSE. Colloids Surf B Biointerfaces. 77:214–218.
- Sathyavathi S, Manjula A, Rajendhran J, Gunasekaran P. 2014. Extracellular synthesis and characterization of nickel oxide nanoparticles from Microbacterium sp. MRS-1 towards bioremediation of nickel electroplating industrial effluent. Bioresour Technol. 165:270–273.
- Sengottaiyan A, Mythili R, Selvankumar T, Aravinthan A, Kamala-Kannan S, Manoharan K, Thiyagarajan P, Govarthanan M, Kim JH. 2015. Green synthesis of silver nanoparticles using Solanum indicum L. and their antibacterial, splenocyte cytotoxic potentials. Res Chem Intermed. [Epub ahead of print]. DOI:https://doi.org/10.1007/s11164-015-2199-7.
- Singh P, Kim YJ, Wang C, Mathiyalagan R, Yang DC. 2015a. Microbial synthesis of Flower-shaped gold nanoparticles. Artif Cells Nanomed Biotechnol. [Epub ahead of print]. DOI:https://doi.org/10.3109/21691401.2015.1041640.
- Singh P, Kim YJ, Singh H, Mathiyalagan R, Wang C, Yang DC. 2015b. Biosynthesis of anisotropic silver nanoparticles by Bhargavaea indica and their synergistic effect with antibiotics against pathogenic microorganisms. J Nanomater. 2015:234741.
- Singh P, Kim YJ, Singh H, Wang C, Hwang KH, Farh mel A, Yang DC. 2015c. Biosynthesis, characterization, and antimicrobial applications of silver nanoparticles. Int J Nanomed. 10:2567–2577.
- Singh P, Kim YJ, Wang C, Mathiyalagan R, El-agamy farh M, Yang DC. 2015d. Biogenic silver and gold nanoparticles synthesized using red ginseng root extract, and their applications. Artif Cells Nanomed Biotechnol. [Epub ahead of print]. DOI:https://doi.org/10.3109/21691401.2015.1008514.
- Singh P, Kim YJ, Wang C, Mathiyalagan R, Yang DC. 2015e. The development of a green approach for the biosynthesis of silver and gold nanoparticles by using Panax ginseng root extract, and their biological applications. Artif Cells Nanomed Biotechnol. [Epub ahead of print]. DOI:https://doi.org/10.3109/21691401.2015.1011809.
- Singh P, Kim YJ, Wang C, Mathiyalagan R, Yang DC. 2015f. Weissella oryzae DC6-facilitated green synthesis of silver nanoparticles and their antimicrobial potential. Artif Cells Nanomed Biotechnol. [Epub ahead of print]. DOI:https://doi.org/10.3109/21691401.2015.1064937.
- Sunkar S, Nachiyar CV. 2012. Biogenesis of antibacterial silver nanoparticles using the endophytic bacterium Bacillus cereus isolated from Garcinia xanthochymus. Asian Pac J Trop Biomed. 2:953–959.
- Swamy MK, Akhtar MS, Mohanty SK, Sinniah UR. 2015. Synthesis and characterization of silver nanoparticles using fruit extract of Momordica cymbalaria and assessment of their in vitro antimicrobial, antioxidant and cytotoxicity activities. Spectrochim Acta Part A. 151:939–944.
- Tran PA, Hocking DM, O'Connor AJ. 2015. In situ formation of antimicrobial silver nanoparticles and the impregnation of hydrophobic polycaprolactone matrix for antimicrobial medical device applications. Mater Sci Eng C. 47:63–69.
- Wang C, Kim YJ, Singh P, Mathiyalagan R, Jin Y, Yang DC. 2015. Green synthesis of silver nanoparticles by Bacillus methylotrophicus, and their antimicrobial activity. Artif Cells Nanomed Biotechnol. [Epub ahead of print]. DOI:https://doi.org/10.3109/21691401.2015.1011805.
- Yáñez-Sedeño P, Pingarrón JM. 2005. Gold nanoparticle-based electrochemical biosensors. Anal Bioanal Chem. 382:884–886.