Abstract
RNA interference (RNAi) guided by small interfering RNAs (siRNA), because of its potential to target and silence the expression of specific genes is utilized as an effective tool in a variety of biological applications. RNAi guided by siRNAs is a powerful tool to attain gene silencing in mammalian cells. One of the features which make siRNA as an amazing biological tool is extremely specific knockdown of target genes by degradation of analogous mRNAs. However, various non-specific effects limit the use of RNAi including the activation of innate immunity and inhibition of inadvertent target genes. One of the most common non-specific effects is inducing the innate immune system including cytoplasmic and endosomal activation of innate immune system, potentially offending the single in mammals. This activation is mainly interceded by immune cells, regularly through a Toll-like receptor (TLR) pathway. The siRNA sequence association of these pathways changes with the sort and position of the TLR involved. In contrast, non-immune cell activation can also arise generally siRNAs which enter into cytoplasm interacting with cytoplasmic RNA sensors such as retinoic acid-inducible gene I. Here, we explain the off-target effects of siRNAs that activate innate immune system and methods to alleviate them, to help enable impressive application of this exciting technology, Also we bold the aspect of molecular strategies permitting the design of therapeutic siRNAs with minute off-target effects.
Introduction
In 1998, Fire and Mello first explained RNA interference (RNAi) in Caenorhabditis elegans. RNAi – referring to a part of non-coding RNA (ncRNA) – is a conserved post-transcriptional gene silencing mechanism used in plant and animal to block gene expression by sequence-specific degradation of mRNA. Small interfering RNA (siRNA) and short hairpin RNA (shRNA) are the two major categories of RNAi. A siRNA usually consists of 21–28 nucleotides, with two 3′-overhangs at either terminus (Czauderna et al. Citation2003, Elbashir et al. Citation2001, Mansoori et al. Citation2014, Meister and Tuschl Citation2004, Tuschl Citation2001, Tuschl and Borkhardt Citation2002). SiRNA-mediated gene silencing is an intracellular mechanism through the targeting and degradation of complementary messenger RNA (mRNA) within the cell cytoplasm (Elbashir et al. Citation2001, Liu et al. Citation2004, Mansoori et al. Citation2014). siRNAs are cut out from longer double-stranded RNA (ds-RNA) chains via Dicer. The siRNA antisense strand is used by an RNA-induced silencing complex (RISC) to induce mRNA cleavage and ultimately mRNA degradation (Mansoori et al. Citation2014). Interest about RNAi has increased as researchers have taken benefit of this intracellular mechanism to find an extensive span of diseases using specially designed siRNAs. shRNA is delivered by bacterial or viral vectors and merged with cell genome and synthesized in the nucleus. shRNA is then transported into the cytoplasm for final processing and mRNA cleavage like siRNA (Mansoori et al. Citation2014). Since the discovery of RNAi, >30 clinical trials including 21 investigations for various biological siRNA drugs – have been conducted. Unlike primary results that showed outstanding specificity in RNAi-interceded gene silencing, over the last 5 years plenty of studies have reported out cases of non-specific mRNA inhibition (Bridge et al. Citation2003, Jackson et al. Citation2003, Citation2006, Sledz et al. Citation2003). Such inadvertent effects on gene expression interceded by RNAi are known as “off-target effects.” These off-target effects include specific and non-specific off-target effects. Specific off-target effects are occurred by partial sequence complementarity of the RNAi manufacture to mRNAs other than the predestinate target (Jackson et al. Citation2003, Citation2006). Non-specific off-target effects include a broad diversity of immune- and toxic-associated effects that are associated with the process of manufacturing of the RNAi itself or its delivery carrier (Bridge et al. Citation2003, Sledz et al. Citation2003). One of the most common non-specific off-target effects is activation of the innate immune system in mammals, resulting in induction of interferon (IFN) and pro-inflammatory cytokines formation. Over time, has been noted that siRNA could induce immune system cells and cytokine production in vitro and in vivo (Karikó et al. Citation2004a, Sioud and Sørensen Citation2003). These off-target effects as potential adverse markers, the specificity of RNAi was more readily questionable. In fact, following the activation of IFNs by siRNA in culture cells (Persengiev et al. Citation2004, Sledz et al. Citation2003) limited the uses of siRNA as suppressors of gene expression.
This review provides a brief overview of the mechanisms surrounding the non-specific activation of the immune system by siRNA and shRNA and also suggests strategies to minimize the consequence of off-target effects.
Recognition of siRNA by immune system
The induction of IFNs and inflammatory cytokines by siRNA has prevented their wider application as gene suppressors. The induction of tumor necrosis factor (TNF)-α and interleukin (IL)-6 production by adherent peripheral blood mononuclear cells (PBMC) was not limited to ds-siRNAs, but also with single-stranded siRNA (ss-siRNA) with both the sense and antisense strands (Uchida et al. Citation2006). While the immunostimulatory off-target effects were most often sequence-dependent (Jackson and Linsley Citation2010), specific activation of IL-6 and TNFα as well as of IFNα formation was more likely dependent on endosomal Toll-like receptor (TLR) pathways. Transfection of immature dendritic cells (DC) with both ds-siRNA and ss-siRNA can trigger IL-12 production and stimulate CD83 (activation marker) expression. The stimulation of inflammatory cytokine and IFNα formation by both ds-siRNA and ss-siRNA by the adherent PBMC was sequence-associated and required endosomal intracellular signaling pathway. Endosomal self-RNA (sense strands) could stimulate TLR signaling in adherent human PBMC and show a molecular schema capable of inducing innate immunity. siRNAs are identified by mammalian immune cells. The pathways responsible for the recognition of siRNAs by mammalian immune cells were primarily not clear (Karikó et al. Citation2004a). siRNAs induce nonspecific activation of the immune system through two pathways including a: endosomal and b: cytoplasmic recognition pathways. The family of TLR expressed by mammalian immune cells distinguishes pathogen-associated molecular patterns (PAMPs) including viral ds-RNA and unmethylated CpG DNA (Medzhitov Citation2001). TLR3, TLR7, TLR8, and TLR9 are the four TLRs participating in immunity activation originated from off-target effects of siRNA therapy (). TLR3, the receptor for ds-RNAs is a candidate for the identification of siRNAs on the basis of immunostimulation. The stimulation of immune cells via siRNAs is sequence-associated and either sense or antisense strands can stimulate cytokine production as effectively as ds-siRNAs (Hornung et al. Citation2005, Judge et al. Citation2005, Sioud Citation2005). Since TLR3 neither distinguishes ss-RNA nor needs sequence specificity (Medzhitov Citation2001), it is unlikely involved in stimulation of the immune cells via siRNAs. TLR7 and TLR8 mediate in a sequence dependent recognition of siRNAs. Researchers represented that, TLR7 is totally needed for stimulation of cytokines in response to siRNAs in immune cells of knockout mice (Hornung et al. Citation2005, Judge et al. Citation2005, Sioud Citation2005). Preferentially, U- and G-rich ss-RNA molecules are able to recognize and activate TLR7 and TLR8 (Diebold et al. Citation2004, Heil et al. Citation2004). Furthermore, human plasmacytoid dendritic cells (pDCs) can recognize siRNAs through TLR7 (Iwasaki and Medzhitov Citation2004, Medzhitov Citation2001) while human monocytes are recognized via TLR8 (Iwasaki and Medzhitov Citation2004, Liu Citation2005). Unmethylated CpG DNAs are recognized by TLR9 and could regulate different responses. Bacterial lipopolysaccharides (LPS) are recognized by TLR4. Therefore, TLR4 is important in shRNA-mediated therapy. Signaling pathways via TLRs need the MyD88 adaptor molecule and advance through multiple intracellular pathways to affect mitogen-activated protein kinase (MAPK), nuclear factor kappa B (NF-κB), and IFN regulatory factor 7 (IRF-7) mobilizations (; Takeda and Akira Citation2005). Activation of these pathways depends on the particular TLR and its ligand, which differs in various immune cells, leading to the production of several cytokines, including TNF, IL-6, IL-12, and IFNα (Iwasaki and Medzhitov Citation2004, Ito et al. Citation2002, Liu Citation2005). Activation of TLRs by their ligands also up regulate the co-stimulatory molecules such as CD40 in DCs, a turning point in the shift from innate to acquired immunity response (Iwasaki and Medzhitov Citation2004, Rossi and Young Citation2005). The intracellular RNA-sensing receptors include the 2′-5′-oligoadenylate synthetases and the ds-RNA-activated protein kinase (PKR) that can activate general protein synthesis blockage (Williams Citation2001) and pathways that induce transcription factors including IFN regulatory factor (IRF)-3 and NF-κB, which lead to activation of genes, such as IFNs (; Sato et al. Citation2000). Other cytoplasmic receptors such as melanoma differentiation-associated gene-5 (MDA-5) and retinoic acid-inducible gene I (RIG-I) contain caspase recruitment domain (CARD) including helicases, which distinguish intracellular ds-RNA.
Figure 1. Pathways activated in the distinction of siRNAs by the mammalian immune system and mammalian cells. siRNA are distinguished by cells of the mammalian immune system and mammalian cells, through extracellular receptors that include TLR3, TLR7 and TLR8 and cytoplasmic receptors such as PKR, OAS, RIG-I, and MDA-5. In endosomal responses, the ligand-binding domain receptors distinguish the siRNAs before they enter into the cytoplasm. This distinction needs time-dependent endosomal maturation to induce downstream signaling through cytoplasmically located Toll-interleukin-1 receptor (TIR)-domains inducing the MAPK, IRF, and NF-κB pathways leading to the expression of pro-inflammatory cytokines and IFN. The cytoplasmic pathways induce MAPK, IRF-3, and NF-κB pathways leading to the expression of pro-inflammatory cytokines and IFN. Activation of PKR and OAS pathways cause in general protein synthesis inhibition. The key shows the size, base compound, functional and structural varieties between oligodeoxynucleotides (ODNs), double-stranded RNA (ds-RNA), small interfering RNAs (siRNAs), and short hairpin RNAs (shRNAs). IFN, interferon; RISC, RNA-induced silencing complex; IRF, interferon regulatory factor; TLR, toll-like receptor; TIR, Toll-interleukin-1 receptor domain; TRIF, TIR-containing adapter inducing IFN-β; PKR, ds-RNA-dependent protein kinase; RIG-I, retinoic acid-inducible protein; MDA-5, melanoma differentiation-associated protein 5; OAS, 2′5′-oligoadenylate synthetase.
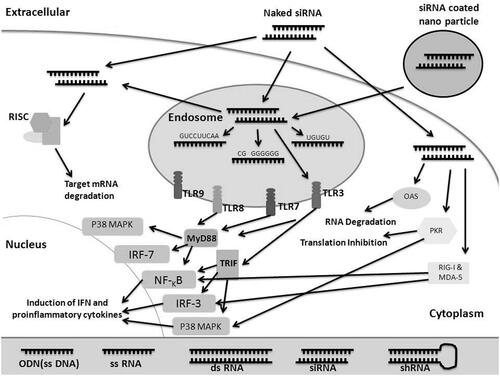
Table I. Pattern recognition receptors (PRRs) distinguish uniquely response to RNA (Bridge et al. Citation2003, Diebold et al. Citation2004, García-Sastre and Biron Citation2006, Hornung et al. Citation2006, Kato et al. Citation2006, Li et al. Citation2006, Schlee et al. Citation2006, Verthelyi and Zeuner Citation2003).
Mechanism of siRNAs recognition by endosome and cytoplasm
TLR7, TLR8, and TLR9 all distinguish nucleic acids including exogenous RNA and DNA. They are expressed in endosomes and needed for endosomal signaling. Endosomal distinguish by TLR9 does not occur by incorporating the nucleic acid with polymers or cationic lipids. Indeed, this has been represented to increase the time that CpG oligodeoxynucleotides (ODN) pass through the endosomes (Honda et al. Citation2005). Many studies have proved that the form of delivery is acute for the immunostimulatory activity of siRNA (Hornung et al. Citation2005, Judge et al. Citation2005, Sioud Citation2005). Transfection of siRNAs in combination with cationic lipids into mice stimulates a cytokine response that weakens the organism (Hornung et al. Citation2005, Judge et al. Citation2005, Sioud Citation2005). Besides, transfection of unmodified and naked siRNAs has no serious effect on immune system stimulation (Heidel et al. Citation2004, Soutschek et al. Citation2004). Two possible elucidations for the lack of immunostimulation by naked siRNAs are as following: first, unmodified or naked siRNA has a brief half-life in serum and may be cleaved before being distinguished by specific receptors. Second, siRNA-lipids complex, like a viral particle, might be more easily distinguished by immune cells than they would by unmodified or naked siRNA, Sioud (Citation2005) showed that naked nuclease-resistant siRNA including a phosphorothioate structure was not immunostimulatory. Distinction of siRNA by TLR is accomplished in the endosome before the siRNA enter into the cytoplasm. Hence, it would be inferred that if siRNA could enter into the cytoplasm avoiding the endosome, they should reserve the induction of immune cells, but still intercede gene silencing. However, electroporation should prepare true cytoplasmic delivery of siRNAs without migrating via the endosome whereas contradictory results have been gained using this method. Sioud (Citation2005) did not apperceive cytokine production after siRNA delivery via electroporation of monocytes, but Hornung et al. showed that, when pDC were electro-porated with naked siRNA, IFNα production was stimulated. Hornung et al. (Citation2005) also showed stimulation of the IFNα formation after electroporation-mediated introduction of siRNAs into the cells (Hornung et al. Citation2005). IL-6 and TNFα stimulation in PBMC are more significant when siRNA are conjugated with poly-L-lysine than when they are complex with polyethylenimine or cationic lipids () (Judge et al. Citation2005). On the contrary, more IFNα is stimulated by siRNA-cationic lipids or siRNA-polyethylenimine complex compared with the same sequence conjugated with poly-Lysine (Judge et al. Citation2005). TNFα stimulation by siRNA is abolished in PBMCs depleted of monocytes, whereas IFNα stimulation is abolished just in pDC-depleted PBMC (Judge et al. Citation2005). There is a distinction in the cytokine profile stimulated in myeloid dendritic cells (mDC) and pDC by the ligand of TLR7 and TLR8(R-848), with IL-12 being stimulated only in mDC and IFNα being stimulated only in pDC (; Ito et al. Citation2002).
The TLRs are not the only class of pattern recognition receptors (PRR) that distinguish among and respond to siRNAs. In addition to TLRs which defend the endosomal area against infectious pathogens, different additional proteins intricate immune response to siRNA in the cytoplasm (Meurs et al. Citation1990). Cytoplasmic distinguish of siRNA via protein kinase receptor (PKR), RIG-I, and MDA-5 pathways () is considered in the letters to play significant roles for siRNA-mediated immune activation. Each of these distinct responses to sequence-independent specifications of RNA. Plenty of the IFN responses are caused by the induction of the ds-RNA-dependent protein kinase R (PKR), a 68 kDa protein that phosphorylates serine/threonine residues of target proteins that are expressed in most mammalian immune cells. However, the particular aspect of siRNA that it recognizes remains unclear. PKR can respond to as small as 11 bp of ds-RNA (Zhang et al. Citation2006) mediated via the α subunit phosphorylation of the eukaryotic translation initiation factor (eIF2a), which involves in the sequestration of the recycling eukaryotic translation initiation factor eIF-2b in an inactive, complex simultaneous manner with eIF2a-GDP. The phosphorylation of elF-2α leads to global suppression of protein expression and cell death (Saunders and Barber Citation2003, Yoneyama et al. Citation2004). PKR also can phosphorylate IκB kinase and induce the NF-κB signaling pathway. Most human cells fundamentally express a slight level of PKR that leaves inactive. In the presence of IFN, PKR expression is boosted while its induction occurred by exogenous ds-RNA (Citation2006, Meurs et al. Citation1990, Williams Citation2001). Both normal siRNA and blunt siRNAs have been shown to stimulate low levels of PKR activation (Marques et al. Citation2006, Meurs et al. Citation1990, Puthenveetil et al. Citation2006). Later studies indicated that cytoplasmic ds-RNA was also recognized by RIG-I and MDA-5 (Kato et al. Citation2006, Kawai and Akira Citation2010, Yoneyama et al. Citation2004). RIG-I (aka DDX58) is a mitochondrial outer membrane binding protein that has two N-terminal caspase and one C-terminal with RNA helicase activity that interacts with exogenous ds-RNA. RIG-I distinguishes the uncapped 5′-triphosphate backbone of ds-RNA or ssRNA (Hornung et al. Citation2006, Kato et al. Citation2006, Pichlmair et al. Citation2006, Yoneyama et al. Citation2004). The RNA helicase domain requires ATPase and accountable for the distinct ds-RNA binding, causing dimerization and activation of downstream signaling. This type of signaling led to induction of NF-κB and IRF-3 that stimulate IFN-β production (Kato et al. Citation2006). Remarkably, RIG-I can distinguish among siRNA with or without 2 bp 3′-overhang. However, only those with blunt terminals could stimulate its ATPase activity and downstream signaling pathways.
There are data to support the concept that the activation of RIG-I was not sequence-associated but rather a result of the physical construction of the siRNA terminal sequence. In addition, a new study has displayed that intracellular expressed shRNAs are not immunostimulatory in human cells. Blunt-ended siRNA can also activate a RIG-I immune response, free from siRNA sequence (Marques et al. Citation2006). This is a warning for synthetic siRNAs transcribed in vitro or by phage polymerases, which often progress blunt terminals (Kim et al. Citation2004). RIG-I is represented in fibroblasts and mDC (Kato et al. Citation2005). By the activation of RIG-I in these cells immunological cascade that generates a potent IFN response is started (; García-Sastre and Biron Citation2006, Marques et al. Citation2006). The cytoplasmic pathway occurs in an extensive variety of mammalian cells, but the endosomal pathway mediated via TLR is restricted to hematopoietic cells.
Sequence, size, and structure-dependent stimulation of immune system by siRNA
It has been demonstrated that ds-RNA longer than 30 bp are strong stimulators of responses in immune system cells (Minks et al. Citation1979). The rate of immunity activation depends on several factors such as the sequence, length, RNA delivery method and the type of immune cell involved in immune responses. For this reason, the use of RNAi in mammalian cells was prevented until it was observed that 21-mer siRNA duplexes were capable of exertion of strong and particular gene silencing in mammalian cells without imposing any specific immunostimulations like those of longer ds-RNAs (Elbashir et al. Citation2001). However, the immunogenic activity of short ds-RNA is more important than it was originally expected. The increasing number of new studies reveals off-target effects originated from immunostimulation caused by ds-RNAs with less than 30 bp (Holen et al. Citation2002, Hornung et al. Citation2005, Judge et al. Citation2005, Kim et al. Citation2004, Marques and Williams Citation2005, Morrissey et al. Citation2005, Reynolds et al. Citation2006, Schlee et al. Citation2006). A total comprehension of the sequence requirements for activation of immune system via siRNA and gene knockdown is necessary before efforts can be made to engineer siRNAs that suppress gene expression without inducing adverse off-target effects. G- and U-rich zone seems to be a general common feature of the immunostimulatory motifs. 5′-GUCCUUCAA-3′ and 5′-UGUGU-3′ are available in the immunostimulatory ss-RNAs (; Hornung et al. Citation2005, Judge et al. Citation2005). Although, many immunostimulatory siRNAs do not include either of motifs mentioned above, others are not stimulatory despite being G- and U-rich zone. Although, many immunostimulatory siRNAs do not include either of motifs mentioned above, others are not stimulatory despite being G- and U-rich zone (Sioud Citation2005). Thus, other specifications, like the situation or ratio of UG dinucleotides inside the molecule or the combination of siding sequences, may act a key role in specifying the nature and power of the cytokine response stimulated by ss-RNA. As well as sequence combination, the size of ss-RNAs may be needed for activation of the immune system cells. Hornung et al. (Citation2005) proved 12-nt ss-RNAs including the immunostimulatory motifs (GUCCUUCAA) were weak stimulators of IFNα in pDC, but by enhancing their size to 16 or 19 nt, cytokine stimulation was recovered (Hornung et al. Citation2005). Sioud (Citation2005) indicated that the single-stranded molecules individually could be potentially more stimulating than the double-stranded ones. Showing that the attendance of single strands is crucial. Replacement of U with A in the siRNA in human immunostimulatory sequence prohibited stimulation of IL-6 and TNFα production by PBMCs, but the replacement of G with A arrests only the stimulation of IFNα in pDC without effecting the stimulation of IL-6, TNFα, and IL-12 formation (Heil et al. Citation2004). Furthermore, siRNA with a UGUGU motif embedded in diverse sequences in human PBMC stimulated qualitatively different cytokine profiles (Judge et al. Citation2005). Every U-rich RNA may be capable for distinction by TLR7 (Diebold et al. Citation2006). The attendance of a 5′-triphosphate or blunt terminal can start RIG-I-mediated immunostimulation (Hornung et al. Citation2006, Kim et al. Citation2004, Marques et al. Citation2006). Double-stranded siRNA with lengths between 23 and 30 bp could stimulate the IFN response, depending on cell type (Reynolds et al. Citation2006). Chemical modifications can reduce the immunostimulatory features of siRNA (Judge 2008). For instance, double-stranded siRNA >90% modified with 2′F-RNA, 2′-O-Me and DNA residues did not exhibit any considerable effect on IFN levels or cytokines, while unmodified double-stranded siRNA led to a significant activation (Morrissey et al. Citation2005). Recently, it has been shown that, a far shorter 2′-O-Me modification is adequate to abolish immunostimulatory properties (Judge et al. Citation2006). Approximately, every degree of modification was sufficient for this purpose; however, 2′-O-methylation of cytidine residues was ineffectual in decreasing immunostimulatory (Judge et al. Citation2006). Moreover, 2′F-RNA and 2′-O-Me modification of uridine prevents immune off-target effects (Hornung et al. Citation2006), such as TLR-dependent and independent immune effects (Cekaite et al. Citation2007). Modification with pseudouracil or 2-thiouracil prevents immunostimulation via RIG-I due to a 5′-triphosphate (Hornung et al. Citation2006). Various types of chemical modifications can decrease the immune effects from different receptors. For instance, demonstrated that just 2-thiouridine was capable of decreasing TLR3-mediated immunostimulation, but others could only decrease TLR7- or TLR8-mediated effects (Karikó et al. Citation2005). An ss-RNA including a CpG motif and a 6 nt poly-(G) at the 3′-terminal has been shown to be able to activate monocytes. However, the receptors that are responsible for distinction of this molecule remain unknown. It is noteworthy that for distinction of CpG ODNs by TLR9, the sequences adjacent to the CpG motif that stimulate a powerful immunostimulatory activity vary among mice and humans (Verthelyi and Zeuner Citation2003). The recombinant TLR9 binds CpG ODN directly in a sequence-specific and pH-associated manner. This means that all requirements for distinction of unmethylated CpG DNA in vivo are made by the receptor itself (Rutz et al. Citation2004). Sequence of an siRNA guarantees not only its gene-specific knockdown potential, but also potency to stimulate immune responses. For this reason, a great deal of interest has been placed on introducing chemical modifications that be promising for gene knockdown while by-passing immune system stimulation (Hornung et al. Citation2005, Koshkin et al. Citation1998). Locked nucleic acid (LNA) contains a methylene linkage among the 2′-oxygen and the 4′-carbon in the ribose ring of the ribonucleotide which can abolish their immunostimulatory activity (Koshkin et al. Citation1998, Koski et al. Citation2004). But, depending on the expanse and position of the LNA modifications, they can block not only the stimulation of IFN but also the knockdown activity of the siRNA (Hornung et al. Citation2005). The presence of DNA nucleotides in siRNAs is acceptable and may even enhance knockdown activity (Chiu and Rana Citation2002). These DNA-RNA hybrids could strongly induce TLR7, TLR8, and TLR9 in vivo, if they include CpG motifs (Sen et al. Citation2004). Even though siRNAs including modifications such as 2′F, 2′O-methyl, and 2′H do not stimulate cytokines when injected into mice, they maintain their knockdowning activity (Morrissey et al. Citation2005). Considering these points it is concluded that immunostimulatory features are not completely understood and more comprehensive studies are needed to securely use the modified siRNAs for therapeutic applications.
Distinction of self and non-self-RNA by immune cells
Exact and effective discrimination between self and non-self-molecules is crucial for effectively activation of the immune system (Medzhitov and Janeway Citation2002). TLRs were primarily supposed to distinguish PAMPs such as bacterial LPS that do not exist on eukaryotic cells (Medzhitov and Janeway Citation2002). Despite, nucleic acids, the ligands for TLR3, TLR7, TLR8, and TLR9 are available in all living organisms and their capability to induce immune cells exposes a pathogenic mechanism in some autoimmune diseases. In spite of this strong threat, many motifs of immunostimulatory nucleic acids are identified (Ishii and Akira Citation2005). An example for self/non-self-distinction for immunostimulatory activity is unmethylated CpG DNA. CpG dinucleotides do not exist in the vertebrate genome; when present, the cytosine is generally methylated (Kariin and Burge Citation1995). In contrast, bacterial DNA has a large incidence of CpG dinucleotides in non-methylated situation (Kariin and Burge Citation1995). In addition, the DNA of vertebrate cells is packed in the nucleus or mitochondria and scarcely gets represented to the cytoplasm or endosomal structure (Ishii and Akira Citation2005). These numerous levels of control indicate the significance of distinction between self and non-self-molecules. Immune complexes including IgG binding to mouse chromatin have been found to induce murine DC at least through TLR9. As a result, inhibition of the CpG dinucleotide and its methylation are essential, but not adequate, to avoid immunostimulation (Boulé et al. Citation2004). Distinction of self- vs. non-self ssRNA is even more intricate because ssRNA like mRNA are frequent in the cytoplasm of all cells. Interestingly, mDCs seem to discriminate structural features between bacterial and eukaryotic RNAs and long poly(A) tails may be accountable for the absence of immunostimulatory activity of mRNA (Koski et al. Citation2004). A transcribed mRNA encoding green fluorescent protein (GFP), irrespective of the existence of the two representatives of eukaryotic mRNA (a short 3′-end poly-(A) and the 7-methyl-guanosine cap of the 5′-end) has been shown to induce production of IFNα by pDC in vitro (Diebold et al. Citation2004). Significantly, human mRNA, when assembled with protamine (Scheel et al. Citation2005) or a cationic lipid (Karikó et al. Citation2004b), are also immunostimulatory to DC. Modifications in eukaryotic RNA, such as 7′G-cap methylation of mRNA, 2′O-methylation of ribosomal RNA, and a conjunction of such modifications into ssRNA, abolish their immunostimulatory activity (Karikó et al. Citation2005).
Conclusion
Similar to any new agent, problem of delivery and specificity are main barriers before siRNAs, in therapeutic applications. Like antisense oligonucleotides, certain synthetic siRNAs stimulate innate immunity through TLRs, particularly TLR7 and TLR8 can lead to important immuno-toxicities by the secretion of IFN s and proinflammatory cytokines. Thus, there is a need for assessment of probable immunostimulatory effects of therapeutic siRNA before clinical applications. The recent studies clearly represent that RNAs with modified uridines can escape the distinction by TLR7 and TLR8. In fact, replacement of 2′-hydroxyl uridines with 2′-deoxy or 2′-fluoro or 2′-O-methyl-modified cohorts abolished immunostimulation by ss-siRNA and ds-siRNA. Unlike TLR7 and TLR8, the distinction of RNA by RIG-I is not sequence dependent. On the other hand, stimulation of innate immunity by either ss-siRNA or ds-siRNA might be useful for treatment of different diseases including cancer. Recent studies have proved that the unmodified siRNA therapeutic anti-angiogenesis activity presently in clinical trials may really be due to TLR3-mediated immune effects (Kleinman et al. Citation2008). Moreover, immunostimulatory siRNAs may be applied as drug to face cancer and chronic viral infections on two aspects: causing gene knockdown and stimulation of immune responses (Schlee et al. Citation2006).
The involvement of immunological signaling pathways is dependent on structure, sequence, and quality of the siRNA and also the siRNA delivery system. The concept of activating an immune response via siRNA-based therapeutics run importantly above what is much considered as “off-target” effects on gene expression mediated with the stimulation of an IFN response. There are major concerns about systemic applications of RNAi where dose-limiting immuno-toxicities may be observed at pre-therapeutic siRNA doses. Inflammatory response is also a relevant concern for drug injection location, due to administration sites and the ability for these to expanse systemically. Even in the lack of clear inflammatory responses, low-grade induction of the immune system by siRNA mainly increases the probability of an antibody reaction against components of any siRNA delivery carrier that is used in vivo. Antibody reactions against the drug carrier can strongly damage multidose therapy regimens because of lack of tissue targeting, quick drug clearance, and their potential to induce hypersensitive reactions in patients when they are exposed to the drug. Engineered siRNAs evade recognition by TLRs and other nucleic acid sensing receptors. In particular, this can be attained by introducing chemically modified nucleotides into the ds-siRNA in a manner that does not mainly RNAi activity. The development in siRNA design demonstrates how chemical modification strategies can conquer many, if not all, of the challenges associated with the immunostimulatory activity of siRNA.
Declaration of interest
The authors report no declarations of interest. The authors alone are responsible for the content and writing of the paper.
References
- Boulé MW, Broughton C, Mackay F, Akira S, Marshak-Rothstein A, Rifkin IR. 2004. Toll-like receptor 9-dependent and -independent dendritic cell activation by chromatin-immunoglobulin G complexes. J Exp Med. 12:9.
- Bridge AJ, Pebernard S, Ducraux A, Nicoulaz AL, Iggo R. 2003. Induction of an interferon response by RNAi vectors in mammalian cells. Nat Genet. 34:263–264.
- Cekaite L, Furset G, Hovig E, Sioud M. 2007. Gene expression analysis in blood cells in response to unmodified and 2′-modified siRNAs reveals TLR-dependent and independent effects. J Mol Biol. 1:90–108.
- Chiu YL, Rana TM. 2002. RNAi in human cells: basic structural and functional features of small interfering RNA. Mol Cell. 10:549–561.
- Czauderna F, Fechtner M, Dames S, Ayguèn H, Klippel A, Pronk GJ, Giese K, Kaufmann J. 2003. Structural variations and stabilising modifications of synthetic siRNAs in mammalian cells. Nucleic Acids Res. 31:2705–2716.
- Diebold SS, Kaisho T, Hemmi H, Akira S, Reis e Sousa C. 2004. Innate antiviral responses by means of TLR7-mediated recognition of single-stranded RNA. Science. 303:1529–1531.
- Diebold SS, Massacrier C, Akira S, Paturel C, Morel Y, Reis e Sousa C. 2006. Nucleic acid agonists for Toll‐like receptor 7 are defined by the presence of uridine ribonucleotides. Eur J Immunol. 36:3256–3267.
- Elbashir SM, Harborth J, Lendeckel W, Yalcin A, Weber K, Tuschl T. 2001. Duplexes of 21-nucleotide RNAs mediate RNA interference in cultured mammalian cells. Nature. 411:494–498.
- García-Sastre A, Biron CA. 2006. Type 1 interferons and the virus-host relationship: a lesson in detente. Science. 312:879–882.
- Heidel JD, Hu S, Liu XF, Triche TJ, Davis ME. 2004. Lack of interferon response in animals to naked siRNAs. Nat Biotechnol. 22:1579–1582.
- Heil F, Hemmi H, Hochrein H, Ampenberger F, Kirschning C, Akira S, et al. 2004. Species-specific recognition of single-stranded RNA via toll-like receptor 7 and 8. Science. 303:1526–1529.
- Holen T, Amarzguioui M, Wiiger MT, Babaie E, Prydz H. 2002. Positional effects of short interfering RNAs targeting the human coagulation trigger tissue factor. Nucleic Acids Res. 30:1757–1766.
- Honda K, Ohba Y, Yanai H, Negishi H, Mizutani T, Takaoka A, Taya C, Taniguchi T. 2005. Spatiotemporal regulation of MyD88–IRF-7 signalling for robust type-I interferon induction. Nature. 434:1035–1040.
- Hornung V, Ellegast J, Kim S, Brzózka K, Jung A, Kato H, et al. 2006. 5′-Triphosphate RNA is the ligand for RIG-I. Science. 314:994–997.
- Hornung V, Guenthner-Biller M, Bourquin C, Ablasser A, Schlee M, Uematsu S, et al. 2005. Sequence-specific potent induction of IFN-α by short interfering RNA in plasmacytoid dendritic cells through TLR7. Nat Med. 11:263–270.
- Ishii KJ, Akira S. 2005. Innate immune recognition of nucleic acids: beyond toll‐like receptors. Int J Cancer. 117:517–523.
- Ito T, Amakawa R, Kaisho T, Hemmi H, Tajima K, Uehira K, et al. 2002. Interferon-alpha and interleukin-12 are induced differentially by Toll-like receptor 7 ligands in human blood dendritic cell subsets. J Exp Med. 11:1507–1512.
- Iwasaki A, Medzhitov R. 2004. Toll-like receptor control of the adaptive immune responses. Nat Immunol. 5:987–995.
- Jackson AL, Bartz SR, Schelter J, Kobayashi SV, Burchard J, Mao M, et al. 2003. Expression profiling reveals off-target gene regulation by RNAi. Nat Biotechnol. 21:635–637.
- Jackson AL, Burchard J, Schelter J, Chau BN, Cleary M, Lim L, Linsley PS. 2006. Widespread siRNA “off-target” transcript silencing mediated by seed region sequence complementarity. RNA. 12:1179–1187.
- Jackson AL, Linsley PS. 2010. Recognizing and avoiding siRNA off-target effects for target identification and therapeutic application. Nat Rev Drug Discov. 9:57–67.
- Judge A, MacLachlan I. 2008. Overcoming the innate immune response to small interfering RNA. Hum Gene Ther. 2:13.
- Judge AD, Bola G, Lee AC, Maclachlan I. 2006. Design of noninflammatory synthetic siRNA mediating potent gene silencing in vivo. Mol Ther. 13:494–505.
- Judge AD, Sood V, Shaw JR, Fang D, Mcclintock K, Maclachlan I. 2005. Sequence-dependent stimulation of the mammalian innate immune response by synthetic siRNA. Nat Biotechnol. 23:457–462.
- Kariin S, Burge C. 1995. Dinucleotide relative abundance extremes: a genomic signature. Trends Genet. 11:283–290.
- Karikó K, Bhuyan P, Capodici J, Weissman D. 2004a. Small interfering RNAs mediate sequence-independent gene suppression and induce immune activation by signaling through toll-like receptor 3. J Immunol. 172:6545–6549.
- Karikó K, Buckstein M, Ni H, Weissman D. 2005. Suppression of RNA recognition by Toll-like receptors: the impact of nucleoside modification and the evolutionary origin of RNA. Immunity. 23:165–175.
- Karikó K, Ni H, Capodici J, Lamphier M, Weissman D. 2004b. mRNA is an endogenous ligand for Toll-like receptor 3. J Biol Chem. 279: 12542–12550.
- Kato H, Sato S, Yoneyama M, Yamamoto M, Uematsu S, Matsui K, et al. 2005. Cell type-specific involvement of RIG-I in antiviral response. Immunity. 23:19–28.
- Kato H, Takeuchi O, Sato S, Yoneyama M, Yamamoto M, Matsui K, et al. 2006. Differential roles of MDA5 and RIG-I helicases in the recognition of RNA viruses. Nature. 7089:101–105.
- Kawai T, Akira S. 2010. The role of pattern-recognition receptors in innate immunity: update on Toll-like receptors. Nat Immunol. 11:373–384.
- Kim DH, Longo M, Han Y, Lundberg P, Cantin E, Rossi JJ. 2004. Interferon induction by siRNAs and ssRNAs synthesized by phage polymerase. Nat Biotechnol. 22:321–325.
- Kleinman ME, Yamada K, Takeda A, Chandrasekaran V, Nozaki M, Baffi JZ, et al. 2008. Sequence-and target-independent angiogenesis suppression by siRNA via TLR3. Nature. 452:591–597.
- Koshkin AA, Singh SK, Nielsen P, Rajwanshi VK, Kumar R, Meldgaard M, Olsen CE, Wengel J. 1998. LNA (Locked Nucleic Acids): synthesis of the adenine, cytosine, guanine, 5-methylcytosine, thymine and uracil bicyclonucleoside monomers, oligomerisation, and unprecedented nucleic acid recognition. Tetrahedron. 54:3607–3630.
- Koski GK, Karikó K, Xu S, Weissman D, Cohen PA, Czerniecki BJ. 2004. Cutting edge: innate immune system discriminates between RNA containing bacterial versus eukaryotic structural features that prime for high-level IL-12 secretion by dendritic cells. J Immunol. 172:3989–3993.
- Li S, Peters GA, Ding K, Zhang X, Qin J, Sen GC. 2006. Molecular basis for PKR activation by PACT or dsRNA. Proc Natl Acad Sci USA 103:10005–10010.
- Liu J, Carmell MA, Rivas FV, Marsden CG, Thomson JM, Song JJ, et al. 2004. Argonaute2 is the catalytic engine of mammalian RNAi. Science. 305:1437–1441.
- Liu YJ. 2005. IPC: professional type 1 interferon-producing cells and plasmacytoid dendritic cell precursors. Annu Rev Immunol. 23:275–306.
- Mansoori B, Shotorbani SS, Baradaran, B. 2014. RNA interference and its role in cancer therapy. Adv Pharm Bull. 4:313–321.
- Marques JT, Devosse T, Wang D, Zamanian-Daryoush M, Serbinowski P, Hartmann R, et al. 2006. A structural basis for discriminating between self and nonself double-stranded RNAs in mammalian cells. Nat Biotechnol. 24:559–565.
- Marques JT, Williams BR. 2005. Activation of the mammalian immune system by siRNAs. Nat Biotechnol. 23:1399–1405.
- Medzhitov R. 2001. Toll-like receptors and innate immunity. Nat Rev Immunol. 1:135–145.
- Medzhitov R, Janeway CA. 2002. Decoding the patterns of self and nonself by the innate immune system. Science. 296:298–300.
- Meister G, Tuschl T. 2004. Mechanisms of gene silencing by double-stranded RNA. Nature. 431:343–349.
- Meurs E, Chong K, Galabru J, Thomas NSB, Kerr IM, Williams BR, Hovanessian AG. 1990. Molecular cloning and characterization of the human double-stranded RNA-activated protein kinase induced by interferon. Cell. 62:379–390.
- Minks MA, West DK, Benvin S, Baglioni C. 1979. Structural requirements of double-stranded RNA for the activation of 2′,5′-oligo(A) polymerase and protein kinase of interferon-treated HeLa cells. J Biol Chem. 254:10180–10183.
- Morrissey DV, Lockridge JA, Shaw L, Blanchard K, Jensen K, Breen W, et al. 2005. Potent and persistent in vivo anti-HBV activity of chemically modified siRNAs. Nat Biotechnol. 23:1002–1007.
- Persengiev SP, Zhu X, Green MR. 2004. Nonspecific, concentration-dependent stimulation and repression of mammalian gene expression by small interfering RNAs (siRNAs). RNA. 10:12–18.
- Pichlmair A, Schulz O, Tan CP, Näslund TI, Liljeström P, Weber F, Reis e Sousa C. 2006. RIG-I-mediated antiviral responses to single-stranded RNA bearing 5′-phosphates. Science. 314:997–1001.
- Puthenveetil S, Whitby L, Ren J, Kelnar K, Krebs JF, Beal PA. 2006. Controlling activation of the RNA-dependent protein kinase by siRNAs using site-specific chemical modification. Nucleic Acids Res. 34:4900–4911.
- Reynolds A, Anderson EM, Vermeulen A, Fedorov Y, Robinson K, Leake D, et al. 2006. Induction of the interferon response by siRNA is cell type- and duplex length-dependent. RNA. 12:988–993.
- Rossi M, Young JW. 2005. Human dendritic cells: potent antigen-presenting cells at the crossroads of innate and adaptive immunity. J Immunol. 175:1373–1381.
- Rutz M, Metzger J, Gellert T, Luppa P, Lipford GB, Wagner H, Bauer S. 2004. Toll‐like receptor 9 binds single‐stranded CpG‐DNA in a sequence‐and pH‐dependent manner. Eur J Immunol. 34:2541–2550.
- Sato M, Suemori H, Hata N, Asagiri M, Ogasawara K, Nakao K, et al. 2000. Distinct and essential roles of transcription factors IRF-3 and IRF-7 in response to viruses for IFN-alpha/beta gene induction. Immunity. 4:9.
- Saunders LR, Barber GN. 2003. The dsRNA binding protein family: critical roles, diverse cellular functions. FASEB J. 17:961–983.
- Scheel B, Teufel R, Probst J, Carralot JP, Geginat J, Radsak M, et al. 2005. Toll‐like receptor‐dependent activation of several human blood cell types by protamine‐condensed mRNA. Eur J Immunol. 35:1557–1566.
- Schlee M, Hornung V, Hartmann G. 2006. siRNA and isRNA: two edges of one sword. Mol Ther. 14:463–470.
- Sen G, Flora M, Chattopadhyay G, Klinman DM, Lees A, Mond JJ, Snapper CM. 2004. The critical DNA flanking sequences of a CpG oligodeoxynucleotide, but not the 6 base CpG motif, can be replaced with RNA without quantitative or qualitative changes in Toll-like receptor 9-mediated activity. Cell Immunol. 232:64–74.
- Sioud M. 2005. Induction of inflammatory cytokines and interferon responses by double-stranded and single-stranded siRNAs is sequence-dependent and requires endosomal localization. J Mol Biol. 348:1079–1090.
- Sioud M, Sørensen DR. 2003. Cationic liposome-mediated delivery of siRNAs in adult mice. Biochem Biophys Res Commun. 312:1220–1225.
- Sledz CA, Holko M, de Veer MJ, Silverman RH, Williams BR. 2003. Activation of the interferon system by short-interfering RNAs. Nat Cell Biol. 5:834–839.
- Soutschek J, Akinc A, Bramlage B, Charisse K, Constien R, Donoghue M, et al. 2004. Therapeutic silencing of an endogenous gene by systemic administration of modified siRNAs. Nature. 432:173–178.
- Takeda K, Akira S. 2005. Toll-like receptors in innate immunity. Int Immunol. 17:1–14.
- Tuschl T. 2001. RNA interference and small interfering RNAs. Chembiochem. 2:239–245.
- Tuschl T, Borkhardt A. 2002. Small interfering RNAs: a revolutionary tool for the analysis of gene function and gene therapy. Mol Intervent. 2:158.
- Uchida T, Scumpia P, Moldawer L. 2006. Variable requirement of dendritic cells for recruitment, but not activation, of NK and T cells to different TLR agonists. Shock. 25:80.
- Verthelyi D, Zeuner RA. 2003. Differential signaling by CpG DNA in DCs and B cells: not just TLR9. Trends Immunol. 24:519–522.
- Williams BR. 2001. Signal integration via PKR. Sci STKE. 2001:re2.
- Yoneyama M, Kikuchi M, Natsukawa T, Shinobu N, Imaizumi T, Miyagishi M, et al. 2004. The RNA helicase RIG-I has an essential function in double-stranded RNA-induced innate antiviral responses. Nat Immunol. 5:730–737.
- Zhang Z, Weinschenk T, Guo K, Schluesener HJ. 2006. siRNA binding proteins of microglial cells: PKR is an unanticipated ligand. J Cell Biochem. 97:1217–1229.