Abstract
We herein first report a method for the synthesis of chitosan (CHI)-folate conjugated colloidal ZnO–Mn+2 quantum dots (QDs) bearing doxorubicin through chemical method (DOX/FA-CHI-QDs) for cancer therapy as well imaging purpose. The entrapment efficiency was determined to be 99.98 ± 0.012% (DOX/FA-CHI-QDs) and 92.0 ± 2.62% (DOX-QDs). The developed DOX/FA-CHI-QDs formulations depict the sustained release pattern at the lysosomal pH (pH 5.0). The DOX/FA-CHI-QDs showed enhanced cytotoxicity, cellular uptake and were most preferentially taken up by the cancerous cells via receptor-mediated endocytosis (RME) mechanism. Hence, the QD-based formulation is capable of targeting drug delivery and imaging the delivery process.
Introduction
Development of nanotechnological tools have now been an area of great interest in the field of drug delivery and targeting. The importance of nano-diagnostic and therapeutic aspects of nanotechnology will be assessed as a new weapon in the arsenal to fight cancer, exploring the advances in areas such as improved drug delivery, new therapies, and bio-imaging. Quantum dots (QDs) made from inorganic materials such as cadmium selenide and zinc oxides and sulphides. QDs are very stable in the body and have been shown to exhibit cytotoxicity including apoptosis. Quantum dots are nanoparticles of certain semiconductor crystals with the novel property of having an extremely narrow emission spectrum (Gaussian distribution) that is directly proportional to the particle's size. QDs have attracted great attention in targeted and imaging due to their unique advantages (Bajwa et al. Citation2015, Ghaderi et al. Citation2011).
In current scenario, QDs is considered as safe and effective drug delivery systems in targeting due to their physicochemical properties such as physical stability (track cell process for longer period of time), photo-stability (fluorescence intensity do not diminish with time), longer fluorescence life time, shape flexibility, blinking property of QDs do not harm quantitative evaluation, luminescent cell marker, and optical contrast agents. The ZnO:Mn+2 QDs have recently gained increasingly interest in targeting and drug delivery, because of ease of synthesis, ability to alter biodistribution and lower toxicity (Bilan et al. Citation2015, Qi and Gao, Citation2008, Zhao and Zeng Citation2015). In QDs, small molecule, hydrophobic drugs can be embedded between the inorganic core and the amphiphilic polymer coating layer. Small size QDs can be easily cleared from body by renal filtration, thus avoiding reticulo-endothelial uptake before reaching the target sites (Bajwa et al. Citation2015, Zhao and Zeng Citation2015).
Doxorubicin (DOX), an anthracycline antibiotic, is a DNA-interacting drug for treatment of various cancers especially breast, ovarian, prostate, brain, cervix and lung cancers. Clinical application of doxorubicin is limited because of its short half-life and severe toxicity to normal tissues, especially gastrointestinal toxicity and heart failure (Mehra and Jain Citation2015, Mehra et al. Citation2014). The cardio-toxicity confines the cumulative dose of DOX to 500–600 mg/m2, which still can be increased for tumor but not for heart disease (Courvreur et al. Citation1971, Sharma et al. Citation2014).
We conjugate the folic acid (FA)-chitosan (CHI) conjugation for enhancing stability, solubility and sustained release of doxorubicin from the QDs based formulation. CHI is biocompatible, biodegradable and responsible for diffusion mechanism in releasing of doxorubicin. Folic acid (FA), targeting ligand was used to target cancerous cells over expressing folate receptors (FRs) (Mehra et al. Citation2014). Chitosan (CHI) enhances transport of polar drug across epithelium and is biocompatible and biodegradable polymer (Gayathri et al. Citation2015, Pal et al. Citation2015). It is a hybrid formulation having propensity to target cancer cells. It is a debut study research report on QDs mediated delivery of doxorubicin. Safety aspects can be improved by reducing exposure of anticancer drug to normal cells. Targeted delivery to cancer cells will improve chemotherapeutic efficacy of anticancer drug. Polymer drug conjugate can provide better control over disposition of anticancer drug in cancerous tissue.
In the present study, the DOX loaded chitosan encapsulated folate conjugated QDs represent a potential platform to deliver tumor targeted drug and document the delivery process simultaneously. The ZnO doped Mn+2 QDS is expected to increase the band gap of ZnO with consequent increase in luminance. Low molecular weight (LMW) fully deacetylated chitosan (CHI) can be conjugated with folic acid for insitu encapsulation of water dispersible ZnO: Mn+2 QDs ().
Materials and methods
Materials
The zinc acetate, manganese acetate, diethanolamine, oleic acid, ethanol, isopropanol, zinc oxide, zinc chloride, manganese chloride, 0.1 N NaOH, n-Heptane, butanol, cetyltrimethyl ammonium bromide, Sol-gel process: zinc acetate, manganese acetate, acetic acid, diethanolamine, isopropanol were purchased from local suppliers as received. Folic acid (FA) was purchased from the HiMedia Laboratory, Mumbai, India. The Doxorubicin HCl was obtained as benevolent gift sample from Sun Pharma Advanced Research Centre, Vadodara, Gujarat, India.
Methods
Chemical method at basic pH (Final formulation)
In chemical method 0.1 M of zinc acetate and 0.2 mM of manganese acetate water solution was taken and it is mixed with 0.2 M DEA water solution. All three solutions were mixed with vigorous stirring at 600 rpm. Now add 14 mmol oleic acid was added to it with vigorous stirring at 800 rpm (20 °C) for 30 min and basic pH 6.2 was adjusted. The temperature and stirring speed was considered during nucleation step. After 30 min of this nucleation, step the solution was refluxed and continuously stirred for 2 h (100 °C and 1221 rpm). The unreacted molecules are filtered and redispersed in ethanol and isopropanol followed by centrifugation at 1600 rpm for 30 min. Mn+2 ions are removed from QDs surface by heating the solution in DEA at 150 °C for 30 min. Obtained nanocrystals were precipitated with ethanol and again centrifuged for 10 min at 1600 rpm which are then dispersed in water (final formulation) (Misra Citation2008a, Citation2008b, Volkov Citation2015).
Folic acid (FA) activation
Folic acid (1.0 g) was dissolved in DMSO (40 mL) and triethylamine (0.5 mL). After addition of N-hydroxysuccinimide (NHS) (0.52 g, 2.2 equivalent), and dicyclohexylcarbodiimide (DCC) (0.50 g, 1.1 equivalent), the mixture was stirred in the dark for 18 h under nitrogen atmosphere. The mixture was filtered to remove the precipitated side-product dicyclohexylurea (DCU), triethylamine was removed by evaporation under reduced pressure, and the remaining solution (FA-NHS) was stored at −20 °C. The collected product was characterized by FT-IR spectroscopy (Lee and Low Citation1994, Mehra and Jain Citation2013).
DOX-chitosan conjugation
Chitosan solution [M.W. 2000, 1 mg/mL in PBS (pH 7.4; 1.05 mM KH2PO4, 155 mM NaCl and 2.9 mM Na2HPO4.7H2O)] was allowed to react with N-succinimidyl S-acetylthiopropionate (SATP, 50 mM in PBS) and was then reacted with 50 mM hydroxylamine solution (10 mM EDTA in PBS) to generate the sulfhydryl group in chitosan. The unreacted SATP was removed by ultrafiltration using a 1.0-K molecular weight cutoff membrane. The chitosan/DOX conjugate was generated by linking the sulfhydryl groups in chitosan and DOX with a heterobifunctional cross linker, succinimidyl 4-(N-maleimidomethyl) cyclohexane-1-carboxylate (SMCC (Sulfosuccinimidyl 4- (N-maleimidomethyl)- cyclohexane-1-carboxylate); 50 mM in PBS). A volume of 20 mL of triethylamine and 300 mL of SMCC (18 mg/mL in DMSO) were added to the DOX solution (0.2 mg/mL in PBS, pH 8). The mixture was incubated at room temperature for 2 h. After adjusting the pH of the mixture to 5.5, 2 mL of sulfhydryl chitosan solution was added (1.5 mg/mL in PBS). The mixture was incubated at room temperature for 2 h and then kept at 4 °C overnight. The free DOX, SMCC and triethylamine were removed from the chitosan/DOX solution via ultrafiltration using a 1.0-KD molecular weight cut-off membrane (Misra Citation2008).
Chitosan-Dox–Folate Conjugation
The 0.25 g of chitosan was dissolved in 25 mL of water and 2.5 mL of acetic acid. The 0.1 g of activated folic acid (FA) with continuous stirring for 10min followed by addition of 0.006 g EDC and 50 mL of DMSO (pH 4.7) to get folate conjugated chitosan (FA-CHI) (Shen et al. Citation2014).
QD-DOX-chitosan-folate conjugation
Ten milliliters of QD (1 g approximately) was added in to 20 mL of Dox-chitosan-folate conjugate by continuous stirring slowly at 400 rpm. Then 0.006 g of EDC was added with continuous stirring for 1 h (Shen et al. Citation2014). The schematic representation has been depicts in .
Characterization of quantum dots
The optimized formulation was characterized for various parameters like spectroscopy techniques, microscopy, size analysis and in-vitro drug release.
Spectroscopy technique
UV-visible spectra
UV/Vis spectroscopy involves promotion of electrons from ground to higher energy state. To obtain structural information regarding chromophoric part of QDs, UV-visible spectrophotometer method was used. Stock solution of 1 mg/mL of doxorubicin was prepared in water and it was further diluted to obtain a concentration of 10 μg/mL. Samples were taken in 1cm standard cuvettes and scanned in range of 200–700 nm in UV-Visible spectrophotometer (SHIMADZU, UV-1700 series, Japan) to determine absorption maxima ().
FT-IR spectroscopy
FT-IR study was performed by Nicolet -380 for identification and structural analysis of synthesized QDs using KBr disc technique. The DOX mixture was ground into a fine powder using an agate mortar/pestle and compressed into KBr disc under hydraulic pressure at 10,000 psi. Each KBr was scanned 32 times at 4 mm s−1 at a resolution of 2 cm−1 over a wave number of 4000–400 cm−1 and characteristics bands were recorded (Mehra et al. Citation2014).
Particle size and size determination
The mean particle size and poly dispersity index (PDI) of prepared quantum dots were determined by using particle size analyzer. The particle size analyzer (Beckman coulter counter DelsaTM Nano C, Beckman Coulter, Brea, CA, USA) is a new series generation instrument that uses photon correlation spectroscopy (PCS), which determines particle size by measuring the rate of fluctuation in laser light intensity scattered by particles as they diffuse through fluid, for size analysis measurement. Five millilitres of QDs solution was taken and size was determined using zeta sizer (Misra Citation2008).
Zeta potential determination
Zeta potential is the potential difference between the dispersion medium and the stationary layer of fluid attached to dispersed particle. Zeta potential was measured by zeta sizer (using special cuvette for zeta potential), working on principle of electrophoretic light scattering (ELS), which determines electrophoretic movement of charged particle under an applied electric field from Dropper shift of scattered light, for zeta potential determination. The zeta potential of optimized QDs was measured (Bilan et al. Citation2015, Mehra and Jain Citation2013, Mehra et al. Citation2014).
In vitro studies
The in vitro release of DOX from DOX/QDs and DOX/QDs final formulation (nanoformulation) was studied in sodium acetate buffer saline (pH 5.3) and phosphate buffer saline (pH 7.4) as recipient media in a modified dissolution method maintaining the physiological temperature (37 °C) throughout the study. The dialysis membrane (MWCO 5–6 kDa, HiMedia, Mumbai, India) filled with the developed optimized nanotubes formulations separately, hermetically tied at both ends and immediately placed into the receptor media maintaining strict sink conditions with constant stirring using magnetic stirrer at RT adjusted to 37 ± 0.5 °C (100 RPM; Remi, Mumbai, India). The aliquots were withdrawn at different time points and volume of recipient compartment was maintained by replenishing with fresh sink solution. The DOX concentration was determined in triplicate at different time points after appropriate dilutions by UV/Visible spectrophotometer at max 480.2 nm (Shimadzu 1601) (Chakravarthy et al. Citation2011).
Cell line studies
The MCF-7 (Michigan Cancer Foundation-7; an estrogen receptor positive human breast cancer cell line derived from pleural effusion) cell line was procured from National Center for Cell Sciences (NCCS), Pune, India for the present study. The MCF-7 cells were cultured in a humidified atmosphere containing atmosphere at 5% CO2 at 37 °C in Dulbecco’s Modified Eagle Medium (DMEM; HiMedia, Mumbai, India) containing 10% fetal bovine serum (FBS; HiMedia, Mumbai, India) supplemented with 2 mM L-glutamine, 1% penicillin streptomycin mixture (Sigma, St. Louis, Missouri) incubated for 24 h for more than 80% confluence. The medium was changed two to three times in a week (Mehra and Jain Citation2013, Citation2015).
Methylthiazole tetrazolium (MTT) cytotoxicity assay
The methylthiazole tetrazolium (MTT) cytotoxicity assay was performed by cleavage of tetrazolium salt [{3-(4,5 dimethyl thiazole-2 yl)-2,5-diphenyl tetrazolium bromide} (MTT)] to a blue formazan derivative by living cells.
Briefly, MCF-7 cells were seeded in 96-well plates with a density of 1 × 104 cells per well and allowed to adhere for 24 h at 37 °C prior to assay. Then the cells in quadruplet wells were treated with free DOX, DOX/QDs and DOX/FA-CHI-QDs final formulation at concentrations – 0.01, 0.1, 10, 100 μg/mL for 24 h. Thereafter, medium was decanted and 50 mL of methylthiazole tetrazolium (MTT) (1 mg/mL) in DMEM ((10 mL; 5 mg/mL in Hank’s balanced salt solution; without phenol red) was added to each well and incubated at 37 °C for 4 h. MTT is reduced by mitochondrial dehydrogenase activity in metabolically active cells to form insoluble formazan crystals. The formazan crystals were solubilized in 50 mL isopropanol by shaking at room temperature for 10 min. Absorbance was measured at 570 nm. The absorbance given by untreated cells was taken as 100% cell survival and the relative (%) cell viability was calculated (Cai et al. Citation2014).
In vivo studies
The Balb/c mice of either sex (200–250 g) were used for present in vivo studies in accordance with the guidelines by Committee for the Purpose of Control and Supervision of Experiments on Animals (CPCSEA) Registration No. 266/2014 CPCSEA I.S.F.C.P. Moga. India. All the experimental animal protocols were approved by the Institutional Animal Ethics Committee and animals were acclimatized at room temperature by maintaining the relative humidity (RH) 55e60% under natural light/dark condition prior to studies.
Tissue/organ biodistribution study
The in vivo biodistribution of the DOX laden MWCNTs formulations and free DOX were studied on Balb/c mice. The sterilized free DOX, DOX/QDs and DOX/QDs conjugates after dispersion in normal saline (0.9%; w/v) were administered intravenously through caudal tail vein route (equivalent dose of DOX ¼ 5.0 mg/kg body weight) into animals. Each mice was administered the same i.v. dose and carefully sacrificed by decapitation method at time intervals of 1, 4, 8, 12 and 24 h for the collection of visceral organs like liver, spleen, kidney, and lungs immediately. The collected organs were washed with Ringer’s solution to separate any adhered debris and dried with the help of tissue paper, weighed and stored under frozen condition till used. Tissues were homogenized (York Scientific Instrument, New Delhi, India) and vortexed after addition of chloroform (CHCl3) and methanol (CH3OH) mixture and ultracentrifuged at 3000 rpm for 15 min (Z36HK, HERMLE LaborTchnik GmbH, Germany). After centrifugation, obtained supernatant was decanted into another vial and evaporated to dryness under nitrogen gas in a bath at 60 ± 2 °C temperature. The dried residue was collected in vials and injected in to an HPLC and analyzed for DOX content by HPLC (Shimadzu, C18, Japan) method, wherein mobile phase consisted of buffer pH 4.0/acetonitrile/methanol (60:24:16; v/v/v) with 1.2 mL/min flow rate at 102/101 bars pressure with adjusting 20 min runtime and peak at 480.2 nm was considered with its retention time (RT) and area.
Results and discussion
In current scenario various multifunctional nanocarriers have been used in drug delivery and targeting. QDs have been attracted great attention in the drug delivery and targeting owing to their unique properties (Bajwa et al. Citation2015, Misra Citation2008).
Folic acid (FA) has inactivated carboxyl group, which was initially activated and used in final formulation. It was observed that the particle size of final formulation was increased upon conjugation of CHI and FA and confirmed attachment onto QDs ().
The activated FA depicts absorption peak of NH- pteridine ring at 3412.64 cm−1 and asymmetric stretching of -CO at 1643 cm−1 shown in . The FTIR spectrum of synthesized QDs () depicts characteristic peak at 2916, 2974 cm−1 confirming the presence of -CH stretching from CH2 alkenes on the surface of QDs. A clear single peak at 1241 cm−1, which could be ascribed to the stretching of the ROR another important characteristic and the peak at 3447.14 cm−1 was ascribed to the OH stretching vibration.
The final DOX/FA-CHI-QDs formulation shows carboxylic acids 3423.02 cm−1, (CH) Alkenes 3011.03 cm1, (-CO) asymmetric bending vibration 1557 cm -1 (). The surface morphology of the DOX/FA-CHI-QDs was determined using motic microscopic at 10, 40 and 100 × and shown in . The motic microscopic shows that the surface topography and nano-size matter of the DOX/FA-CHI-QDS.
The average particle size (nm) and particle size distribution with polydispersity index (PDI) were determined by Beckman coulter counter) is a new series generation instrument that uses photon correlation spectroscopy (PCS) at room temperature (RT). The average particle size and particle size distribution of the DOX-QDs and DOX/FA-CHI-QDs final formulation were found to be 14.6 ± 0.28 nm and 29.4 ± 0.20 nm with polydispersity index (PDI) of 0.266 ± 0.014 and 0.228 ± 0.002, respectively. The average particle size and PDI clearly suggest that the developed DOX/FA-CHI-QDs formulation have narrow particle size distribution with low polydispersity index (PDI).
The drug loading efficiency and in-vitro release behavior are the most important, prerequisite characteristic in the development and characterization of targeted drug delivery system. The percent DOX entrapment efficiency was studied following a modified dialysis diffusion technique and was found to be significantly higher for DOX/FA-CHI-QDs formulation. The in drug entrapment efficiency the characteristic absorption peak of DOX at 480.2 nm was slightly shifted, due to the indicative interaction between DOX and QDs. A significant improvement in the entrapment efficiency was observed from in DOX/FA-CHI-QDs 99.98 ± 0.012 (n = 3).
Earlier it was reported that the formulation shows burst release. A direct measurement showed that about 35% of bound DOX was released from QD-DOX conjugate after 5 h of incubation in an acidic environment ().
Figure 5. Percent cumulative DOX release from the QDs formulations at pH 5.0 and 7.4. Values represent mean ± S.D. (n=3).
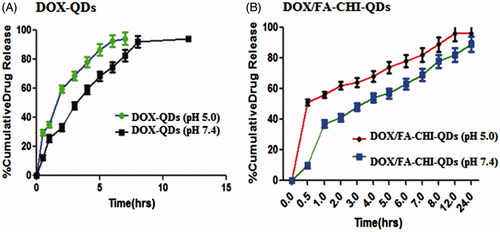
The entrapment efficiency of QD-DOX and DOX/FA-CHI-QDs formulation was found to be 92.2 ± 0.022% and 99.98 ± 0.012%, respectively, which indicates that DOX/FA-CHI-QDs formulation, has more drug entrapment efficiency compared with the formulation.
In-vitro diffusion study of both type of QDs formulation was carried out upto 24 h using cellophane membrane. The data show that the % drug release is more in acidic medium compared with phosphate pH, indicates the targeted anticancer drug delivery at acidic pH of cancer cells. The data shows that more than 50% of the total formulation was released in 15 h while DOX-QDs in 5 h. From the data it is also observed that the QD final formulation shows sustained release compared with DOX-QDs which shows burst drug release. The DOX/FA-CHI-QDs formulation depicts significant sustained release pattern of DOX in acidic microenvironment ().
The cell viability was determined of plain DOX, DOX-QDs, DOX/FA-CHI-QDs final formulation at six different concentration. Cytotoxicity produced by DOX/FA-CHI-QDs formulation showed enhanced cytotoxicity compared with plain DOX. But interestingly, cytotoxicity of DOX/FA-CHI-QDs was found to be much higher than the DOX-QDs formulation. This proves that through final formulation of QDs the drug is better targeted and retained in cancerous cells because of better targeting ability of QDs final formulation. These results indicate that the drug loaded The DOX/FA-CHI-QDs final formulation is based on the multi-functionalities and novel, effective approach towards cancer management with better targeted of highly toxic chemotherapeutic drugs and thus providing fever side effects ().
Images of MCF-7 cells were taken after 2 h of incubation with FITC loaded plain DOX, QD-DOX-QDs and DOX/FA-CHI-QDs formulation on Olympus CKX41 fluorescence microscope. We were able to see the fluorescence on B excitation filter microscope and image were taken at 20 × magnification (). Fluorescence in the cells can be clearly seen in images, which shows that QDs have been uptaken by cells. The DOX/FA-CHI-QDs final formulation shows better uptake compared with plain DOX and DOX-QDs (). The developed DOX/FA-CHI-QDs show similar results with earlier report (Misra Citation2008, Salva et al. Citation2011).
Figure 7. The fluorescence images of the treated MCF-7 cell lines with DOX, DOX-QDs and DOX/FA-CHI-QDs formulations.
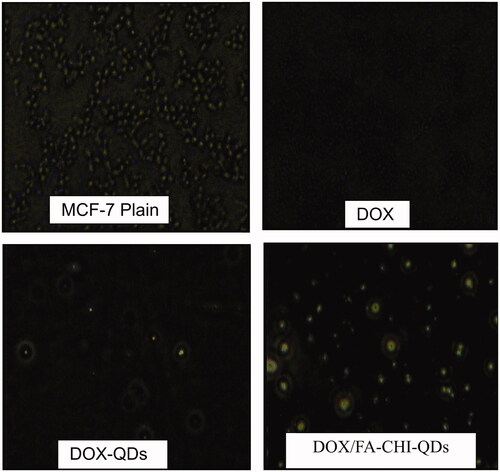
The percentage of drug in different organs was determined by HPLC method by preparing standard plots in different organs homogenates of rats. Dynamic biodistribution () study revealed brief initial peak in lung, liver, kidney, spleen uptake, as expected from transient increase in blood-pool activity. As previously found in in-vivo biodistribution study, liver was the organ which predominantly uptake for QDs formulation followed by kidney, lung, liver and spleen.
Figure 8. Tissue-organ biodistribution study for DOX, DOX-QDs and DOX/FA-CHI-QDs different formulation.
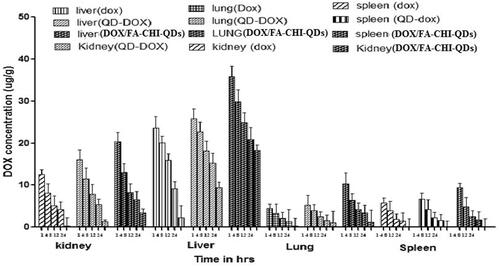
In this study results showed that Balb/C mice, which were treated with DOX-QD and QD final formulation (DOX/FA-CHI-QDs) showed significantly higher accumulation of drug in liver than in other tissues as liver is the primary location of RES and hence primary organ for QDs uptake. The DOX delivered through nanoparticles experienced enhance distribution to organs of RES system. QDs show significantly lower concentration than DOX solution at all the time points studied.
The DOX/FA-CHI-QDs formulation experienced an initial rapid clearance of blood followed by slow clearance after 2 h of injection. The final formulation showed the higher concentration throughout the study. The experimental results revealed that the biodistribution of DOX is greatly altered when delivered through QDs final formulation. The QDs significantly enhanced the circulation half-life of doxorubicin in blood. The QDs final formulation give better sustained release effects compare to DOX-QDs.
Conclusion
Quantum dots (QDs) formulation prepared in this investigation have different semiconductors (ZnO in the core and Mn+2 at the shell). QDs have been characterized by FTIR spectrum, UV Visible Spectroscopy, motic imaging and zeta size analysis. The motic image of QDs showed its fluorescent property. The increase in size of QDs loaded doxorubicin hydrochloride was observed after folate conjugation and chitosan encapsulation. The UV spectrum absorbance was detected at 200 nm. The drug entrapment of the formulation was found to be 99.8% compared with only QD-DOX have been improved. As depicted from the data of in vitro drug release the formulation shows higher release rate in acidic pH compared to normal physiological pH hence the formulation could be successfully explored for drug delivery to cancerous cells. Hence, from the proposed work it is concluded that the drug loaded bifunctionalized chitosan encapsulated quantum dots will enhance cellular apoptosis of tumor cells. Moreover, by using drug loaded, bifunctionalized chitosan encapsulated QDs, the time taken for the tumor cells to go into apoptotic state can also be monitored by confocal microscopic techniques.
Declaration of interest
The authors report no conflicts of interest related to manuscript.
References
- Bajwa N, Mehra NK, Jain K, Jain NK. 2015. Pharmaceutical and biomedical application of quantum dots. Artif Cells Nanomed Biotechnol. 2015, (article in press; 1-11) https://doi.org/10.3109/21691401.2015.1052468.
- Bilan R, Fleury F, Nabiev I, Sukhanova A. 2015. Quantum dot surface chemistry and functionalization for cell targeting and imaging. Bioconj Chem. 26:609–624.
- Cai D, Gao W, He B, Dai W, Zhang H, Wang X. 2014. Hydrophobic penetrating peptide PFVYLI-modified stealth liposomes for doxorubicin delivery in breast cancer therapy. Biomaterials. 35:2283–2294.
- Chakravarthy KV, Davidson BA, Helinski JD, Ding H, Law WC, Yong KT, Prasad PN, Knight PR. (2011). Doxorubicin-conjugated quantum dots to target alveolar macrophages and inflammation. Nanomedicine. 7:88–96.
- Courvreur P, Kante B Gristain L, Roland MS, Peiser P. 1971. Toxicity of polyallylcyanocrylate nanoparticles II: doxorubicin loaded nanoparticles. J. Pharm. Sc. 71:786–789.
- Gayathri S, Ghosh OSN, Sudhakara P, Viswanath AK. 2015. Chitosan conjugation: a facile approach to enhance the cell viability of LaF3: Yb, Er upconverting nanotransdcers in human breast cancer cells. Carbohydr Polym. 12:302–308.
- Ghaderi S, Ramesh B, Seifalian A. 2011. Fluorescence nanoparticles “quantum dots” as drug delivery systems and their toxicity: a review. J Drug Target. 19:475–486.
- Leamon CP, Low PS. 1991. Delivery of macromolecules into living cells- a method that exploits folate receptor endocytosis. Proc Natl Acad Sci. 88:5572–5576.
- Lee RJ, Low PS. 1994. Delivery of liposomes into cultured KB cells via folate receptor-mediated endocytosis. J Biol Chem. 269:198–204.
- Mehra NK, Jain NK. 2013. Development, characterization and cancer targeting potential of surface engineered carbon nanotubes. J Drug Target. 21:745–758.
- Mehra NK, Jain NK. 2015. One platform comparison of estrone and folic acid anchored surface engineered MWCNTs for doxorubicin delivery. Mol Pharm. 12: 630–643.
- Mehra NK, Verma AK, Mishra P, Jain NK. 2014. The cancer targeting potential of D-α-2-tocopheryl polyethylene glycol 1000 tethered multiwalled carbon nanotubes. Biomaterials. 35:4573–4588.
- Misra N, Roy M, Mohanta D, Baruah KK, Choudhury A. (2008a). Photochromism and magneto-optic response of ZnO: Mn semiconductor quantum dots fabricated by microemulsion route. Cent Eur J Phys. 6:109–115.
- Misra RDK. 2008. Quantum dots for tumor-targeted drug delivery and cell imaging. Nanomedicine. 3:271–274.
- Pal A, Taludkar D, Roy A, Ray S, Mallick A, Mandal C, Ray M. 2015. Nanofabrication of methylglyoxal with chitosan biopolymer: a potential tool for enhancement of its anticancer effect. Int J Nanomed. 10:3499–3518.
- Qi L, Gao X. 2008. Emerging application of quantum dots for drug delivery and therapy. Expert Opin Drug Deliv. 5:263–267.
- Salva R, Taratula O, Garbazenko O, Minko T. 2011. Tumor targeted quantum dots-Mucin 1 aptamer-doxorubicin conjugate for imaging and treatment of cancer. J Controll Rel. 153:16–22.
- Sharma H, Kumar K, Choudhary C, Mishra PK, Vaidya B. 2014. Development and characterization of metal oxide nanoparticles for the delivery of anticancer drug. Artif Cell Nanomed Biotechnol. Early online. (Epub ahead of print) https://doi.org/10.1186/1556-276X-9-558.
- Shen M, Jia W, Lin C, Fan G, Jin Y, Chen X, Chen G. 2014. Facile synthesis of folate-conjugated magnetic/fluorescent bifunctional microspheres. Nanoscale Res Lett. 9:558.
- Volkov Y. 2015. Quantum dots in nanomedicine: recent trends, advances and unresolved issues. Biochem Biophys Res Comm. https://doi.org/10.1186/s11671-015-0873-8
- Zhao MX, Zeng EZ. 2015. Application of functional quantum dots nanoparticles as fluorescence probes in cell labeling and tumor diagnostic imaging. Nanoscale Res Lett. 10:171.