Abstract
The main aim of the present investigation was to explore the effect of functionalization on drug delivery potential of carbon nanotubes (CNTs) and to compare the in vitro and in vivo cancer targeting potential of doxorubicin HCL (DOX)-loaded ox-/multi-walled CNTs (MWCNTs), DOX-loaded PEG-MWCNTs and DOX-loaded FA-PEG-MWCNTs. The DOX/PEG-FA-MWCNTs showed enhanced cytotoxicity and were most preferentially taken up by the cancerous cells. The obtained results also support the extended resistance time and sustained release profile of drug-loaded surface-engineered MWCNTs. Overall, we concluded that the developed MWCNTs nanoformulations have higher cancer targeting potential.
Introduction
Surface-engineered multifunctional carbon nanotubes (CNTs) mediated targeted and controlled drug delivery represent as valuable, promising nano-architecture due to unique physicochemical properties. CNT were discovered by Sumio Iijima in 1991 during his transmission electron microscope (TEM) experiment (Iijima Citation1991). CNTs have ability to easily cross the cell membranes devoid of any obvious toxicity (Mehra et al. Citation2015a). CNTs are the third allotrope and belong to the fullerene family member. CNTs comprises of thin graphite sheets of condensed benzene rings rolled up into hollow tubular cylinder with tiny nano-needle shape which attracted great interest in biomedical as well as healthcare applications. CNTs gain the popularity over other nano-carriers due to nano-size diameter and length, ultra lightweight, high aspect ratio, rich surface chemistry, photoluminescence, non-immunogenicity, biocompatibility, biliary excretion pathway, high drug cargo ability, etc. (Kayat et al. Citation2015, Mehra et al. Citation2015a, Sharma et al. Citation2015). CNTs have been classified into the main four classes based on the presence of walls: (i) Single-walled CNTs (SWCNTs) consists of only single graphitic sheet wrapped into cylindrical tube structure with diameter ranges between 0.4 and 2.5 cm and length varies from 20 to 1000 nm. (ii) Double-walled CNTs (DWCNTs) are coaxial nanostructures, with two concentric graphene cylinders. It is a synthetic blend of SWCNTs and MWCNTs. (iii) Triple-walled CNTs (TWCNTs) are made up of three concentric graphene layers. (iv) Multi-walled CNTs (MWCNTs) are made up of several concentric layers of graphene and tend to form the unordered clumps. Their diameter varies from 1.4 to 100 nm and lengths vary from 1 to several micrometers (Mehra and Jain Citation2015a,Citationb).
The generation of functional groups creation onto the surface of CNTs is termed to be functionalization. The pristine CNTs are found to be unsuitable for interaction with biomolecules because they have a tendency to agglomerate or bundling and lack of solubility in an aqueous milieu. These major hurdles can improve upon by functionalization through chemical modifications on CNTs, which enhances its aqueous solubility. The chemical modification can do by adsorption, interactions (covalent and non-covalent interactions), carboxylation, amidation, acylation, esterification, PEGylation and polymer coatings (Datsyuk et al. Citation2008, Mehra et al. Citation2014, Mittal et al. Citation2010).
In the present investigation, our main aim was to investigate the effect of functionalization (degree of functionalization) of CNTs on targeting potential by employing MCF-7 cells. We also compared the PEGylated and non-PEGylated MWCNTs bearing DOX.
Materials and methods
The pristine multi-walled CNTs and Polyethylene-bis amine 3000 were purchased from Sigma-Aldrich Pvt. Ltd. (St. Louis, MO) for present studies. Doxorubicin hydrochloride (DOX) was taken from Sun Pharmaceutical Advanced Research Centre (Vadodara, Gujarat, India). Acetone, folic acid, N-N dimethyl formamide, sulfuric acid, methanol and Triton-X-100 were purchased from Loba Chemie Pvt Ltd. (Mumbai, India).
Purification of carbon nanotubes (CNTs)
Oven purification
MWCNTs (100 mg) were put into a vacuum oven and the temperature was maintained up to 250 ± 2 °C for 30 min (Lodhi et al. Citation2013).
Microwave treatment
MWCNTs (100 mg) were put into a microwave oven and the temperature was maintained at 100 °C for 15 min. Microwave-treated MWCNTs were characterized by Fourier transform infrared (FTIR) spectroscopy and zeta potential (Jain et al. Citation2009).
Piranha treatment
The piranha method was investigated for dispersing and oxidizing 5 mg of MWCNTs using the mixture of concentrated HNO3:concentrated H2SO4 in 1:3 in which MWCNTs were dispersed in an acid mixture for 2 h at 123 °C. After that, MWCNT dispersion was sonicated for 3 h. After sonication, this dispersion was washed with de-ionized water to neutralize the pH filtered. Then, filtered dispersion was again re-dispersed in NH4OH (69–72%): H2O2 and magnetically stirred for 1 h and 30 min then dried at 50 °C for 24 h and further characterized (Datsyuk et al. Citation2008, Li et al. Citation2007, Mehra and Jain Citation2013, Mehra et al., Citation2014).
Dispersion analyses of carbon nanotubes
MWCNTs were dispersed in different surfactants i.e., sodium lauryl sulfate (SLS), Triton-X-100 and Tween-80. Twenty milligrams of SLS was added into 1 ml dispersion of acid-treated MWCNTs, which leads to purification of MWCNTs and reduced the size of CNTs (Jain et al. Citation2009, Kayat et al. Citation2015, Mittal et al. Citation2010).
Synthesis of activated folate-conjugated PEG-bis-amine 3000
Step 1: Preparation of N-Hydroxy succinimide (NHS) ester of folic acid
Folic acid (FA) (1.0 g) was dissolved in dimethyl sulfoxide (40 ml) and triethylamine (500 μl). Then, NHS (520 mg) and dicyclohexyl carbodimide (500 mg) was added and stirred in dark for 18 h under nitrogen atmosphere. The mixture was filtered to remove precipitated side product dicyclohexyl urea, triethyl amine (TEA) was removed by evaporation under reduced pressure and remaining solution was stored at −20 °C (Scheme 1). The collected product was characterized by FTIR spectroscopy (Mittal et al. Citation2010).
Step 2: Conjugation of FA with PEG-bis-amine 3000
FA-NHS was coupled to PEG-bis-amine 3000 following previously reported method (Mehra and Jain Citation2013, Mittal et al. Citation2010). The 2.6 ml of FA-NHS stock solution was added to PEG-bis-amine (500 mg) in DMSO (5.0 ml) in the presence of TEA (0.4 μl) and the continuous mixture was stirred overnight (Scheme 2). Then, the product was dialyzed against using dialysis membrane to remove the un-conjugated folic acid
.Step 3: Conjugation of FA-PEG-bis-amine with MWCNTs
Carboxylated MWCNTs (10 mg) were dispersed in DMSO and 1-Ethyl-3-(3-dimethylaminopro-pyl) carbodiimide dissolved in DMSO (6.4 mg/ml) was added to it with constant magnetic stirring for 6 h at 100 rpm followed by an addition of FA-PEG-bis-amine conjugate (4.6 mg/ml). The reaction was continued under vigorous stirring up to 5 d and remaining un-conjugated FA-PEG-NH2 was removed by dialysis and the product was collected, dried and characterized by using FTIR spectroscopy (Mehra and Jain Citation2013).
Step 4: Preparation of PEG/MWCNTs
Cut and purified MWCNTs (3 mg) was dispersed in ultrapure water (50 ml) and pre-sonicated for 30 min. PEG or PEG-FA aqueous solution (3 mg/ml) was added into the suspension dropwise, and keeps sonication for another 10 min and then magnetically stirring for 24 h at room temperature (RT). The blank sample was collected by centrifugation at 11,000 rpm for 15 min. The obtained centrifugations were further purified five times by dispersing in 50 ml ultrapure water and centrifugation (Niu et al. Citation2013).
Step 5: Doxorubicin drug loading in PEG-FA-MWCNTs
DOX loading was performed by simply mixing DOX (2 mg/ml) with the PEG-FA-MWCNTs dispersions (0.5 mg/ml) in phosphate buffered saline (PBS) pH 7.4 and stirred for 24 h. The formed complex (DOX/PEG-FA-MWCNTs, DOX/PEG-MWCNTs and ODX/MWCNTs) DOX were collected by repeating ultracentrifugation (11,000 rpm, 30 min) with PBS until the supernatant became free of reddish color (corresponding to free DOX). Unbounded DOX in solutions is calculated by measuring the absorbance at 490 nm (characteristic absorbance of DOX) and referring to calibration curve recorded at the same condition. Therefore, DOX-loaded nanotubes can be obtained after subtracting the dosage of unbounded DOX by the initial dosage of DOX. The drug loading efficiency (%) here is defined as the ratio of the dosage of DOX-loaded onto the nanotubes and the dosage of the nanotubes (Niu et al. Citation2013). Thereafter, DOX laden MWCNTs were separated by the centrifugation to remove free/unbound DOX until the solution became color-free, and measured at 480.2 nm spectrophotometerically (UV-Visible spectrophotometer) using a calibration curve prepared under the same condition. The doxorubicin loading efficiency was calculated spectrophotometerically using the following formula:
The product was collected, dried and lyophilized and stored at 5 ± 3 °C for further studies (Das et al. Citation2013, Mehra et al. Citation2014).
Characterization of pristine and surface-engineered MWCNTs
FTIR spectroscopy
The FTIR spectroscopy were performed using compressed KBr pellet method in PerkinElmer FTIR spectrophotometer (Perkin Elmer 783, Pyrogen 1000 spectrophotometer, Shelton, CT) and scanned in the range from 4000 to 500 cm−1 (Mehra et al. Citation2014).
Average particle size and particle size distribution (PSD) measurement
The average particle size and particle size distribution (PSD) of pristine and surface-engineered MWCNTs were determined by photon correlation spectroscopy in a Malvern Zeta-Sizer Nano ZS90 (Malvern Instruments, Ltd., Malvern, UK) at RT (Mehra and Jain Citation2013).
In vitro release study
The in vitro DOX release from DOX/ox-MWCNTs, DOX/PEG-FA-MWCNTs and DOX/PEG-MWCNTs nano-formulations were studied in sodium acetate buffer saline (pH 5.3) and PBS (pH 7.4) as recipient media in a modified dissolution method maintaining the physiological temperature (37 ± 0.5 °C) throughout the study. The dialysis membrane (MWCO 5–6 kDa, Hi-Media, Mumbai, India) filled with the developed optimized nanotube formulations separately, hermetically tied at both ends and immediately placed into the receptor media maintaining strict sink conditions with constant stirring using magnetic stirrer at RT adjusted to 37 ± 0.5 °C at 100 rpm. The aliquots were withdrawn at different time points and volume of recipient compartment was maintained by replenishing with fresh sink solution. The DOX concentration was determined in triplicate at different time points after appropriate dilutions by UV/Visible spectrophotometer at λ max 480.2 nm (Huang et al. Citation2011, Mehra et al. Citation2014, Ren et al. Citation2012).
Cell culture studies
Michigan cancer foundation 7 (MCF-7) (human breast cancer cells), was cultured in Dulbecco’s modified Eagle medium (DMEM) supplemented with 10% heat-inactivated fetal calf serum, 2 mm glutamine, 1% penicillin and streptomycin mix to discourage growth of microorganisms and maintained in humidified atmosphere at 5% CO2 at 37 ± 0.5 °C grown to 80% confluence in tissue culture humidified environment (Liu et al. Citation2007).
Cell viability assay
The cytotoxicity study was performed by cleavage of tetrazolium salt [{3-(4, 5dimethylthiazole-2yl)-2, 5-diphenyl tetrazolium bromide (MTT). MCF-7 cells were incubated in 96-well transparent plates of 2 × 105cells/well. The cells were incubated for 24 h in media containing DOX-loaded nanoformulations at the equivalent drug concentration of 0, 0.01, 0.1, 1, 10 and 100 μg/ml. The nano-formulations were sterilized with UV irradiation for 1 d prior to use. MTT assay was used to measure the cell viability at given time intervals. The absorbance of the wells was measured by microplate reader (ELISA) with wave length 480 nm. The absorbance given by untreated cells was taken as 100%. The percent cell viability defined as the percentage of absorbance of wells containing the cells incubated with nano-formulations (Liu et al. Citation2007).
Procedure for cell uptake studies
Folate receptors over expressed MCF-7 cells were seeded on glass cover-slips (18 × 18 mm) placed into 35 mm tissue at a density of 2 × 105 cells (in 2 ml, growth medium). After overnight growth, supernatants from the culture emulsion of DOX-loaded nanoformulations at 37 °C were added. Upon incubation for 4 h, cells were washed with PBS and observed under fluorescence microscopy after excitation at 480 nm (Liu et al. Citation2007, Shi et al. Citation2009).
Analysis of pharmacokinetic parameters after an intravenous (i.v.) administration
The different pharmacokinetic parameters were determined after an intravenous (i.v.) administration of free DOX, DOX/ox-MWCNTs, DOX/PEG-FA-MWCNTs and DOX/PEG-MWCNTs with the same i.v. dose (5 mg/kg body weight) in Wistar rats DOX content in liver, lungs, kidney and spleen were measured at different time intervals (1, 4, 8, 12 and 24 h). The blood samples were collected from the retro-orbital plexus of the eye with diethyl-ether. The tube was flushed with 0.3 ml of heparinized normal saline solution to prevent blood clotting. The samples were centrifuged at 13,000 rpm for 10 min to obtain plasma supernatant for further analysis. Plasma DOX concentration in plasma was determined by high performance liquid chromatography (Mehra et al. Citation2014).
In vivo biodistribution studies
The in vivo biodistribution study of free DOX, DOX/MWCNTs and DOX/PEG-FA-MWCNTs was measured using Wistar rats weighing 200–250 kg. Twenty-four rats were divided into four groups: Group A (Free DOX), Group B (DOX/MWCNTs), Group C (DOX/PEG-MWCNTs) and Group D (DOX/PEG-FA-MWCNTs). An i.v. dose (5.0 mg/kg body weight) was injected to rats. At the end of each time point, animals were sacrificed and organs (kidney, liver, lungs and spleen) were removed carefully, washed and weighed. Weighed tissue samples were suspended in 2 ml ethyl acetate and homogenized for 5 min. The homogenate was transferred into eppendorf tubes and centrifuged at 3000 rpm for 10 min at 4 °C. The organic layer was subsequently transferred into the second tube and evaporated to dryness under vacuum. The compound was re-suspended in 1 ml of the mobile phase, vortexed and then stored at deep-freeze conditions (−70 ± 2 °C) until analyzed. Supernatant was spiked with the drug (DOX) to prepare a stock solution containing concentration (100 μg/ml). Further dilutions were made for 10, 20, 30, 40 and 50 μg/ml from the stock solution by appropriately diluting with homogenate media. Drug concentration in different organs was analyzed by high-performance liquid chromatography (Mehra et al. Citation2014).
Results and discussion
The surface-engineered CNTs played an important role in research and development, including drug targeting arena. Pristine CNTs are purified by hot air oven treatment (heated at 250 °C), microwave treatment (120 °C for 15 min), piranha treatment (HNO3:H2SO4:NH4OH:H2O2), refluxing method (H2SO4:HNO3 in 3:1) for 8 h. Oxidation is one of the most common and prerequisite techniques for grating hydrophilic functional groups (carboxylic, amino groups, etc.) at the ends and sidewalls of nanotubes through strong oxidizing acid treatment, which increases the aqueous dispensability of nanotubes.
The acid-treated MWCNTs shows characteristic peaks at 3447.17 cm−1 and 2925.59 cm−1 due to OH and CH-stretching of CH2 functional groups, respectively. The characteristic peak at 1633.58 cm−1 was found for asymmetric stretching of C = O bond and at 3062.11 cm−1 was due to COOH group. shows the FTIR spectrum of acid-treated MWCNTs and shows the characteristic peak interpretation of acid-treated MWCNTs. The FTIR spectrum of conjugated PEG-FA-MWCNTs showed various characteristic peaks of an aromatic compound at 3423.64, 2917.33, 1654.36, 1437.34 and 1316.39 cm−1. The characteristic peak at 1020.47 cm−1 was due to C-O stretch of ether linkage (). The average particle size and PSD with a polydispersity index (PDI) was determined by photon correlation spectroscopy. The particles of procured MWCNTs were observed to be 1776.2 nm with 0.733 nm. The particle size of MWCNT after purification by different methods was given below in .
Table I. The characteristic peaks interpretation of functionalized MWCNTs.
Table II. The particle size of MWCNTs after purification.
The dispersion analysis of MWCNTs was performed in different surfactants and found to have maximum dispersion order in the range: SLS > TritonX-100 > Tween-80. The dispersion of MWCNTs in different surfactants is shown in and .
Figure 3. Dispersion analysis of MWCNTs in different surfactants (Triton X-100, Tween-80 and sodium lauryl sulfate).
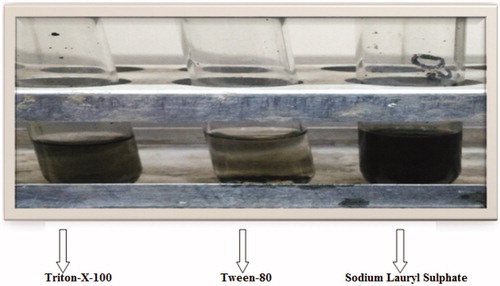
Table III. The dispersion of MWCNTs in different surfactants.
The drug loading efficiency and in vitro release behavior are most important, prerequisite characteristics in the development and characterization of targeted drug delivery system. A significant improvement in entrapment efficiency was observed from 95.4% (DOX/MWCNTs) to 99.5% (DOX/PEG-FA-MWCNTs). Due to its aromatic nature, DOX tends to strongly interact with sidewall and ends of nanotubes through π–π stacking and hydrophobic interactions.
The scanning electron microscope (SEM) of the functionalized MWCNTs at 50,000 × and 30,000 × magnification shown in and show the motic microscopic image of DOX-loaded functionalized MWCNTs. The microscopic image clearly revealed the tiny nano-needle shape structure of the CNTs. As literature suggest that the CNTs able to perforate and cross the plasma membrane.
The in vitro release profile of any targeted drug delivery system is a crucial parameter for determining their overall clinical pharmaceutical efficacy. We investigated the cumulative DOX/PEG-FA-MWCNTs and DOX/MWCNTs nano-formulations at temperature 37 °C for 48 h in PBS (pH 7.4) and sodium acetate buffer solution (pH 5.3) corresponding to physiological and endosomal pH of cancerous cells, respectively. The pH of cytosol is neutral to mildly alkaline (pH 7.4–7.8) while lysosomal pH is acidic (4–5.5). The cumulative DOX release DOX pattern exhibited a non-linear release profile characterized by relatively faster release followed by sustained and slower release. shows the in vitro release pattern of various nanoformulations (Das et al. Citation2013, Mehra and Jain Citation2015a,c, Mo et al., Citation2015).
The efficacy of the formulations to defeat cancer cells is reflected by their cytotoxicity. For the cancer cells incubated with 0, 0.01, 0.1, 1, 10 and 100 μg/ml drug concentration, percentage cell viability after 24 h treatment was measured for free DOX and DOX-loaded MWCNTs nanoformulations. After 24 h treatment, the % cell viability was found to be higher in DOX/PEG-FA-MWCNTs nanoformulations as compared to free DOX. It is clear that the DOX/PEG-FA-MWCNTs formulation demonstrate lower cytotoxicity and higher cell viability than the free DOX formulation at same drug concentration and exposure time, which means for the same therapeutic effect, the drug needed for the nanoformulations, could be less than that for the free DOX. The development of nanoformulations thus can enhance the therapeutic effect as well as to increase the targeting of DOX (Liu et al. Citation2007). Percent cell viability of MCF-7 cell after treated with free DOX, DOX/MWCNTs, DOX/PEG-MWCNTs and DOX/PEG-FA-MWCNTs is shown in .
Figure 7. Percent cell viability of MCF-7 cell after treated with free DOX, DOX/MWCNTs, DOX/PEG-MWCNTs and DOX/PEG-FA-MWCNTs.
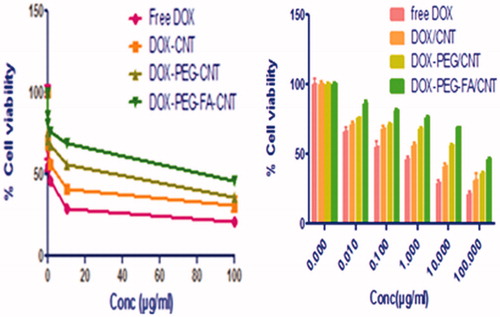
The cell uptake study of the formulation was performed for quantitative estimation of nano-formulations by cancerous cells. Cell uptake study of drug-loaded CNTs was performed on MCF-7 cells. The cell culture was freshly prepared by subculturing the cells using DMEM. MWCNTs formulations were incubated with cells using six-well culture plates for 2 h. Images of cells were taken after 2 h of incubation with plain DOX, DOX/MWCNTs, DOX/PEG-MWCNTs and DOX/PEG-FA-MWCNTs final formulation on Olympus CKX41 fluorescence microscope. We were able to see the fluorescence B excitation filter microscope and images were taken at 20 × magnification. DOX/PEG-FA-MWCNTs showed higher fluorescence intensity as compared to other nanoformulations and free DOX. The observed higher fluorescence intensity clearly suggests the higher uptake for DOX/PEG-FA-MWCNTs formulations possibly due to receptor-mediated endocytosis and nano-needle specific mechanism (Mehra et al. Citation2014). shows the cellular uptake of DOX in MCF-7 cell lines.
The PEGylated MWCNTs significantly increase the blood circulation time of the anticancer drug. The obtained results also support extended residence time and sustained release profile of DOX-loaded surface-engineered nanotubes formulation in the body as compared to DOX solution. Pharmacokinetic parameters like t1/2 and MRT of DOX/PEG-FA-MWCNTs was found to be 13.87 h and 19.43 h which were higher as compared to DOX solution. The various pharmacokinetic parameters of DOX obtained from free DOX, DOX/MWCNTs, DOX/PEG/MWCNTs and DOX-PEG-FA/MWCNTs shown in .
Table IV. The various pharmacokinetic parameters.
In biodistribution study, the amount of DOX (DOX/PEG-FA- MWCNTs) was found to be in order: Liver > Kidney > Spleen > Lungs. The biodistribution study of nanoformulations in different organs is given below in .
Conclusion
To the best of our knowledge, this is the complete study report with evidence of improved selective treatment of tumors using a degree of functionalization of MWCNTs, which are most suitable as sustained and targeted drug delivery. The above finding results suggested that the functionalized MWCNTs suggest that the better targeting response using MCF-7 breast cancer cell line with higher % cell viability and lower cytotoxicity as compared to free DOX. From the above outcomes of our present research studies, it can be concluded that DOX-loaded surface-engineered MWCNTs showed better in vivo, ex vivo efficacy as compared to other nanocarriers depicting higher drug loading (99.5%) and sustained release profile especially at acidic microenvironment corresponding to conditions existing at cancerous tissues/sites. In vitro release studies about 96.72% of DOX in DOX/PEG-FA-MWCNTs was released at pH 5.3 suggested that DOX release pattern exhibited non-linear release profile characterized by relatively initial faster release followed by sustained or slower release in the later period. The improved kinetics of nanotubes formulation upon PEGylation such as t1/2 and MRT, 13.87 and 19.43 h, respectively for DOX/PEG-FA-MWCNTs as compared to free DOX may be considered significantly effective for intravenous administration and improved the biocompatibility of DOX in DOX/PEG-FA-MWCNTs. The organ biodistribution study in the case of DOX/PEG-FA-MWCNTs was observed to be higher in liver, kidneys, spleen and lungs and rapid decline in overall formulation thereafter indicated that most of the MWCNTs were eliminated through renal excretion pathway. In cellular uptake studies, the quantitative estimation of DOX was determined to be higher in DOX/PEG-FA-MWCNTs with high fluorescence intensity. However, folate conjugation makes it more targetable approach for precluding the non-targeting sites. Thus, optimal therapeutic response and improved biocompatibility may be achieved with minimal side effects associated with the carrier and an anti-cancer drug.
Declaration of interest
The authors report no conflict of interest related to manuscript.
References
- Das M, Datir SR, Singh RP, Jain S. 2013. Augmented anticancer activity of a targeted, intracellularly activatable, theranostic nanomedicine based on fluorescent and radiolabeled, methotrexate-folic acid-multiwalled carbon nanotube conjugate. Mol Pharm. 10:2543–2557.
- Datsyuk V, Kalyva M, Papagelis K, Parthenios J, Tasis D, Siokou A, et al. 2008. Chemical oxidation of multiwalled carbon nanotubes. Carbon. 46:833–840.
- Huang H, Yuan Q, Shah JS, Misra RD. 2011. A new family of folate-decorated and carbon nanotube-mediated drug delivery system: synthesis and drug delivery response. Adv Drug Deliv Rev. 63:1332–1339.
- Iijima S. 1991. Helical microtubules of graphitic carbon. Nature. 354:56–58.
- Jain AK, Dubey V, Mehra NK, Lodhi N, Nahar M, Mishra DK, et al. 2009. Carbohydrate-conjugated multiwalled carbon nanotubes: development and characterization. Nanomedicine. 5:432–442.
- Kayat J, Mehra NK, Gajbhiye V, Jain NK. 2015. Drug targeting to arthritic region via folic acid appended surface engineered multi-walled carbon nanotubes. J Drug Target. [Epub ahead of print]. doi:10.3109/1061186X.2015.1077846.
- Li CC, Lin JL, Huang SJ, Lee JT, Chen CH. 2007. A new and acid-exclusive method for dispersing carbon multi-walled nanotubes in aqueous suspensions. Colloids Surf A Physicochem Eng Asp. 297:275–281.
- Liu Z, Sun X, Nakayama-Ratchford N, Dai H. 2007. Supramolecular chemistry on on water-soluble carbon nanotubes for drug loading and delivery. ACS Nano. 1:50–56.
- Lodhi N, Mehra NK, Jain NK. 2013. Development and characterization of dexamethasone mesylate anchored on multi walled carbon nanotubes. J Drug Target. 21:67–76.
- Mehra NK, Jain K, Jain NK. 2015a. Pharmaceutical and biomedical application of surface engineered carbon nanotubes. Drug Discov Today. 20:750–756.
- Mehra NK, Jain NK. 2013. Development, characterization and cancer targeting potential of surface engineered carbon nanotubes. J Drug Target.21:745–758.
- Mehra NK, Jain NK. 2015a. Cancer targeting propensity of folate conjugated surface engineered multi-walled carbon nanotubes. Colloids Surf B Biointerfaces. 132:17–26.
- Mehra NK, Jain NK. 2015b. Multifunctional hybrid-carbon nanotubes: new horizon in drug delivery and targeting. J Drug Target. [Epub ahead of print]. doi:10.3109/1061186X.2015.1055571.
- Mehra NK, Jain NK. 2015c. One platform comparison of estrone and folic acid anchored surface engineered MWCNTs for doxorubicin delivery. Mol Pharm. 12:630–643.
- Mehra NK, Verma AK, Mishra PR, Jain NK. 2014. The cancer targeting potential of D-α-tocopheryl polyethylene glycol 1000 succinate tethered multi walled carbon nanotubes. Biomaterials. 35:4573–4588.
- Mittal V, Patel S, Sheth S. 2010. Development and characterization of folate targeted nanoparticle drug delivery system. Int J Pharm Bio Sci. 1:113.
- Mo Y, Wang H, Liu J, Lan Y, Guo R, Zhang Y, et al. 2015. Controlled release and targeted delivery to cancer cells of doxorubicin from polysaccharide-functionalized single-walled carbon nanotubes. J Mater Chem B. 3:1846–1855.
- Niu L, Meng L, Lu Q. 2013. Folate conjugated PEG on single walled carbon nanotubes for targeting delivery of doxorubicin to cancer cells. Macromol Biosci. 13:735–744.
- Ren J, Shen S, Wang D, Xi Z, Guo L, Pang Z, et al. 2012. The targeted delivery of anticancer drugs to brain glioma by PEGylated oxidized multi-walled carbon nanotubes modified with angiopep-2. Biomaterials. 33:3324–3333.
- Sharma P, Mehra NK, Jain K, Jain NK. 2015. Biomedical applications of carbon nanotubes: a critical review. Curr Drug Deliv. 12. PMID:26201344.
- Shi X, Wang SH, Shen M, Antwerp ME, Chen X, Li C, et al. 2009. Multifunctional dendrimer-modified multiwalled carbon nanotubes: synthesis, characterization, and in vitro cancer cell targeting and imaging. Biomacromolecules. 10:1744–1750.