Abstract
Context The rate of co-infection of HIV/Tuberculosis is increasing alarmingly. This calls for a drug delivery approach targeting both diseases.
Objective The study aims to investigate co-loading of isoniazid, an antitubercular drug and lamivudine an antiretroviral drug, into polymeric microspheres for simultaneous treatment of both diseases.
Materials and methods Microspheres were prepared by o/o emulsion solvent evaporation method by employing ethylcellulose and eudragit RS 100 as polymers. The prepared formulation was suitably characterized for FTIR, DSC, percent yield, loose surface crystals, entrapment efficiency, and in vitro studies. The surface morphology of microspheres was observed using digital microscope and scanning electron microscope. Cell viability study was done on Caco-2 cells.
Results and discussion FTIR and DSC studies demonstrated compatibility and stability of excipients. Microscopy studies revealed that particles were spherical in shape and distributed over a range of 120–270 μm. Percent yield, LSC and %EE have shown promising results. In vitro release showed biphasic release pattern with sustained release up to 12 h. Mechanism of drug release followed Higuchi Kinetics and non-fickian release behaviour. The formulation containing drug/polymer ratio 1:2 and EU/EC of 1:1 showed optimum response in context to achievement of controlled release. The cell viability studies showed that the prepared system had no toxic effect on intestinal epithelial Caco-2 cells.
Conclusion Polymeric microspheres were prepared and suitably characterized for simultaneous delivery of two drugs. This matrix system could be used for better therapeutic outcome in this deadly co-infection.
Introduction
WHO (Citation2015) reported Tuberculosis (TB) as the second most population prevalent disease after HIV in causing death from infectious diseases worldwide (Dye et al. Citation2005, Raviglione and Pio Citation2002) Globally, about one-third of population is infected with Mycobacterium tuberculosis, the causative agent of TB, with approximately eight million new cases and two million deaths every year. Further, according to another report released by WHO it is estimated that one-third of 39.5 million HIV-infected people worldwide are coinfected with TB and are about 26–31 times more susceptible to develop active TB due to this opportunistic infection (WHO Citation2015). Both the diseases affect the progression of each other and lead to co-morbidity. This unfortunate synergy has significantly increased the human death toll and is becoming a severe health hazard posing high economic burden at the same time. Many mathematical models making use of differential equations have been applied to understand the dynamics of this coinfection transmission in population (Sharomi et al. Citation2008).
At present, there is no joint therapy for these two diseases (Jain et al. Citation2007). In chronic treatment for prolonged duration, the traits like safety and efficacy, short and simplified treatment, better management of directly observed treatment, short course and antiretroviral therapy strategy, simplified drug supply management, shipping and distribution, increasing patient compliance, providing new treatments for (multi drug resistant (MDR))-TB/HIV are highly desirable (Burman and Jones Citation2001, Jain et al. Citation2007). It is crucial for the success of AIDS and TB therapy to maintain systemic drug levels consistently above its therapeutic drug concentration throughout the course of the treatment. All the above-desired criteria can be met very well by controlled release microspheres.
Since many years, microencapsulation technique has been employed to convert a solution of drugs and polymers into free flowing microspheres. Microsphere-based drug delivery systems have evolved as one of the most promising means of the sustained and controlled drug delivery. Microspheres with their special characteristics to embed drug in polymer matrix can improve drug bioavailability and reduce the dosing frequency (Balamuralidhara et al. Citation2012), provide sustained release and may resolve the problem of non-adherence to prescribed therapy and improve patient compliance, which is one of the major obstacles in the control of TB and AIDS epidemics.
Microspheres have been into research since decades for the management of TB and HIV (Agnimitra et al. Citation2010, Pandav and Naik Citation2014, Vaghani et al. Citation2011). The fixed-dose drug combination microspheres present themselves as a better alternative for sustained and controlled drug therapy while increasing compliance and being more economic at the same time (Bulut and Dilek Citation2014). Ain et al. (Citation2003) evaluated pharmacokinetic and therapeutic effects of alginate microparticles loaded with antitubercular drugs, i.e., isoniazid (INH), rifampicin and pyrazinamide in guinea pigs. The drug microparticles exhibited sustained release of INH, rifampicin and pyrazinamide for 3–5 d in plasma and up to 9 d in organs (Sharma et al. Citation2003). Thus, the drugs can be administered orally weekly to improve the patient compliance.
Lamivudine (LAM) sulfate is an antiretroviral drug which acts by inhibiting nucleoside reverse transcriptase enzyme (Agnimitra et al. Citation2010). INH is one of the most important “first-line” anti-tubercular drugs used for the treatment of TB. This centerpiece drug acts by getting converted to its active form by an enzyme KatG and subsequently inhibiting the synthesis of mycolic acid needed for cell wall of mycobacteria (Timmins and Deretic Citation2006). Out of various polymers, ethyl cellulose (EC) is the most commonly employed polymer in microencapsulation technique for controlling drug release. In 2007, Sahoo et al. (Citation2007) prepared stavudine-loaded microspheres of EC alone and EC in combination with polyvinyl pyrrolidone which provided controlled release of the drug (Sahoo et al. Citation2007). In the same year, Katakam et al. prepared and characterized antiretroviral drug LAM containing microspheres using various cellulose polymers (cellulose acetate phthalate, cellulose acetate butyrate, EC and hydroxypropylmethylcellulose acetate phthalate). The obtained microspheres had shown zero-order, diffusion-controlled release behavior (Katakam et al. Citation2014). Das and Rao (Citation2006) encapsulated zidovudine in EC microspheres by double emulsion solvent diffusion technique and obtained high entrapment efficiency (EE) with sustained release (Das and Rao Citation2006).
In fact, combination of EC with acrylic polymers such as Eudragit RS (EU) has produced more controlled and sustained release characteristics (Javed et al. Citation2014). Vaghani et al. (Citation2011) studied various EU and EC combination as promising controlled release carriers for 5-fluorouracil (Vaghani et al. Citation2011). Pandav and Naik (Citation2014) observed higher encapsulation efficiencies of the drug with EC and EU and RL 100. Also, in vitro release was prolonged for 12 h (Pandav and Naik Citation2014).
Therefore, the present study was undertaken keeping in view the fact that both TB and AIDS are chronic diseases requiring long-term treatment. The microspheres of two candidate drugs viz. LAM and INH alone and in fixed dose combination were prepared to achieve a controlled drug release profiles of the drug in order to treat this dynamic coinfection with a single formulation having sustained effect suitable for per oral administration. The microspheres were prepared by oil-in-oil (o/o) emulsion solvent evaporation technique using EU100 and EC as a matrix polymer. The combination of INH and LAM was expected to simultaneously treat TB and AIDS with improved efficacy and compliance.
Materials and methods
Materials
LAM and INH were generously gifted by Mylan Laboratories (Nashik, India) and Lupin Pharma Lab (Pune, India), respectively. EC, EU and magnesium stearate procured from HiMedia Laboratories, Mumbai, India. MTT (3-(4,5-dimethylthiazol-2-yl)-2,5-diphenyltetrazolium bromide) dye was from Sigma-Aldrich, St. Louis, MO. All other solvents and chemicals used were of analytical grade and were purchased from SD Fine chemicals (Mumbai, India).
Preparation of microspheres
The microspheres were prepared using modified o/o emulsion solvent evaporation technique as mentioned elsewhere (Kim et al. 2002). The batches were prepared according to the formulation design given in . Briefly, the accurately weighed quantity of drug and polymers were dissolved in a solvent mixture consisting of 10 ml acetone and 15 ml ethanol and stirred to obtain a homogeneous solution. Magnesium stearate (50 mg) was dispersed in this solution. The dispersion was then added in a drop wise manner to 50 ml of liquid paraffin pre-emulsified with 0.02% span 80 under agitation at 1000 rpm for 3 h using mechanical stirrer (Remi Instruments, Mumbai, India) to facilitate complete solvent evaporation. The formed microspheres were collected by decanting the liquid paraffin and washing thrice with 50 ml n-hexane to remove excess of liquid paraffin. They were dried at room temperature for 12 h and stored in desiccators for further evaluation.
Table I. Composition of various microsphere batches.
Characterization of microspheres
Size, shape and surface morphology
The size, shape and surface morphology of the prepared microspheres were observed by a digital microscope (Eclipse E200, Nikon, Melville, NY) and the average size was measured using NIS-Elements F 3.0 software (Nikon, NY). Environmental scanning electron microscope (FEI, Quantum 200E Instrument, OR) equipped with secondary electron detector was also used to study the surface of microspheres. The microspheres were placed on the aluminum stub pre-coated with silver glue to enhance the conductivity and analyzed in low vacuum at various magnifications.
Yield (%)
The prepared microspheres were dried properly and weighed accurately. Yield (%) was calculated from the ratio of weight of microspheres obtained to the total weight of drug and non-volatile excipients added during preparation using following equation:
Loose surface crystal study
Beyond entrapment inside the microspheres, some of the drug present or adsorbed on the surface of microspheres is called loose surface crystal (LSC). For determining LSC, 100 mg of dried microspheres were directly dispersed in 50 ml phosphate buffer pH 7.4 and stirred at 100 rpm for 10 min. The content was then filtered through Whatman filter paper of pore size 0.45 μm. The filtrate was then suitably diluted with buffer and analyzed spectrophotometrically by the method developed by Pandey and Mishra (Citation2013) to calculate LSC. All the determinations were calculated in triplicate.
Entrapment efficiency
Entrapment of drug was calculated by subtracting LSC from the total amount of drug found in microspheres. It is expressed as %EE. Briefly, 100 mg of dried microspheres were crushed properly and dispersed in 50 ml phosphate buffer pH 7.4. The content was stirred for 2 h and filtered through the Whatman filter paper of pore size 0.45 μm. It was then suitably diluted and analyzed spectrophotometrically. The amount of drug was calculated by UV spectrophotometric method as described earlier. All the determinations were done in triplicate. The %EE was evaluated using following equation:
Solid state characterization
Fourier Transform Infrared (FTIR) spectroscopy
To estimate the compatibility between excipients Fourier Transform Infrared (FTIR) spectroscopic study was done. FTIR spectra of pure drugs (INH and LAM), polymers (EC and EU), a physical mixture of drugs and physical mixtures of both drugs with both polymers were obtained by the conventional KBr pellet method using FTIR (Shimadzu, Model 8400, Kyoto, Japan). The samples were scanned in the range of 400 and 4000 cm−1. Further to evaluate interactions between excipients during formulation, FTIR spectra of microspheres was obtained.
Differential scanning calorimetry (DSC)
Thermal curves of LAM, INH, EU, EC and microspheres were obtained using differential scanning calorimeter (Mettler-Toledo, Columbus, OH). Each sample was scanned at a rate of 10°C per minute over the range of 0–300°C maintaining nitrogen flow at 50 ml/min and taking an empty aluminum crucible as reference pan.
In vitro release study
In vitro release study was performed in phosphate buffer pH 7.4 for 12 h using USP (United States Pharmacopoeia) dissolution apparatus type II. The rpm was set at 50 and temperature was maintained at 37 ± 0.5°C. The weight of microspheres equivalent to 100 mg of the drug was placed in the beaker. Five-milliliter aliquot was withdrawn at pre-set time interval (0.5, 1, 2, 3, 4, 5, 6, 7, 8, 10 and 12 h) and replaced by an equal volume of fresh dissolution medium having the same temperature. The samples were suitably diluted and analyzed spectrophotometrically.
The mechanism of drug release from microspheres was calculated from in vitro release data by fitting into various kinetic equations. The kinetic equations used were zero-order, first-order and Higuchi model and Korsmeyer–Peppas equation. The rate constants and regression coefficient were also calculated for the respective models using Microsoft excel 2010. The value of diffusion coefficient “n” indicative of the mechanism of release was determined from Korsmeyer–Peppas equation to determine exact mechanism of drug release from microspheres:
where Mt/M∞ is the fraction of drug released, k is rate constant and t is time.
In vitro cell viability study
Preparation of extract
The microspheres weighing 100 mg were incubated in 50 ml of phosphate buffer pH 7.4 at 37 °C. One milliliter sample was collected and equal volume at same temperature was added to it after 6 h, 12 h and 24 h. It was stored at −20°C until use.
Cell line study
The Caco-2 cells were grown in Dulbecco’s modified Eagle medium supplemented with 10% fetal bovine serum and antibiotic–antimycotic solution. When the cells reached confluency they were trypsinized and plated in a 96-well plate at a number of 10,000cells/well. The plate was then incubated overnight at 37°C. After that 100 μl of the extract and 100 μl of media was added to the culture wells and they were incubated at 37°C for 24 h. After 24 h, the extract and media was removed and 20 μl MTT was added (5 mg/ml) and cells were again incubated for 4 h. The MTT solution was then removed and 200 μl of dimethyl sulphoxide (DMSO) was added to each well to dissolve the formed purple-colored formazan crystal. The plate was then analyzed at 492 nm using a microplate reader. The percent cell viability was calculated taking growth in control well as 100%. Also, the cells were treated with 4′,6-diamidino-2-phenylindole (DAPI) to stain the nucleus and images using fluorescent microscope (Carl Zeiss, Oberkochen, Germany) were taken.
Results and discussion
Formulation of microspheres
Microspheres, possessing a number of advantages over other novel drug delivery systems, are a very suitable means of sustained and controlled drug delivery. An o/o emulsion solvent evaporation method was employed for preparing microspheres which is a simple, economic and efficient method having good batch to batch reproducibility and suitability for laboratory as well as industrial scale. The solvent evaporation method is the most preferred method as it requires less expertise, excipients and is suitable for encapsulation of hydrophilic drugs with wide range of polymers. The method involves removal of the one organic phase by extraction with other organic solvent or oil phase (liquid paraffin). Here, organic solvent (ethanol + acetone) versus liquid paraffin-based emulsion system was used for the preparation of microspheres.
FTIR spectra
shows FTIR spectra of pure drug INH, LAM and their physical mixture, respectively. The FTIR spectrum of INH showed one band at about 3306 cm−1 due to N-H stretching and a weaker band at 3111.28 cm−1 due to the secondary amide group. Two bands overlapped, one is at 1664.62 cm−1, for N-H bending and other is at 1637.62 cm−1 for C = O bending and appeared as doublet. Further, a strong band appeared at 1560.46 cm−1 following combination of a C-N stretching band and an N-H bending band. A band appeared at 1411.94 cm−1 due to C = C stretching of ring carbons and a band at 2862 cm−1 represents C-H[4]n symmetric stretching. Also, a band appeared at 1141.90 cm−1 due to N-X (x = NH2) stretching. The FTIR spectrum of LAM showed characteristic peak of the carbonyl group present in the cytidine nucleus at 1653.06 cm−1, a band of peaks at 3327.32 cm−1 and 3200.01 cm−1 owing to amino and hydroxyl groups and peaks at 1286.56 cm−1 and 1164.32 cm−1 due to asymmetrical and symmetrical stretching of the C-O-C system of oxathiolane ring. The spectrum of EC showed characteristics absorption band for -C-O-C- stretching vibration at 1022.31 cm−1 and C-H stretching bands at 2875.96 cm−1 and 2976.26 cm−1. A band at 1377.22 cm−1 appeared due to C-H bending. The characteristic peaks of both the drugs are retained in the FTIR spectra of physical mixture of both the drugs (). Moreover, ) shows the infrared spectra of EC, EU, physical mixture of both the drugs and polymers and microspheres. The prominent peaks of both the drugs are retained in spectra of physical mixture and microspheres, indicating the drugs and polymers does not exhibit any interaction and are suitable to be formulated as microspheres.
Differential scanning calorimetry
DSC thermograms are shown in LAM (b) INH (c) EU (d) EC (e) microspheres. The sharp melting temperature as exhibited by LAM and INH at 175°C and 180°C, respectively indicates their crystalline nature. However, endotherm of EC and EU show broad peaks confirming amorphous nature of polymers. Additionally, microspheres showed a broad peak at about 170–230°C is due to the presence of amorphous polymer matrix and concluding that the drug is uniformly dispersed in the matrix.
Surface morphology
The prepared microspheres were observed using both optical microscope and scanning electron microscope. From photomicrographs, it was clear that the particles were spherical to slightly oblong in shape ().
Figure 4. (a) Digital microscopic images of microspheres of optimized batch. (b) Digital microscopic images of co-loaded microspheres.
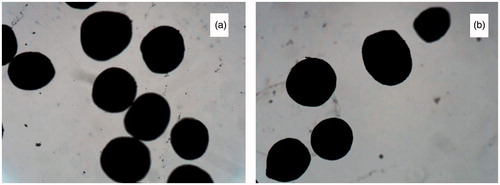
The SEM images at various magnifications were collected for detailed analysis of the shape and surface morphology of the prepared microspheres. The SEM photograph of optimized formulation batches of EC microspheres, EU microspheres and combined microspheres were observed at 200 × magnification (). It shows spherical microspheres with rough surface. The roughness is attributed to deposition of needle shaped drug crystals. This surface-associated drug adsorbed on the surface of the microspheres is responsible for burst release and help to enhance drug concentration at diseased site (Rajinikanth et al. Citation2008).
Figure 5. SEM images of: (a) EC microspheres, (b) EU microspheres, (c) combined microspheres at 200 × magnified surface of combined microspheres at (d) 500 × (e) 1000 × and (f) 5000×.
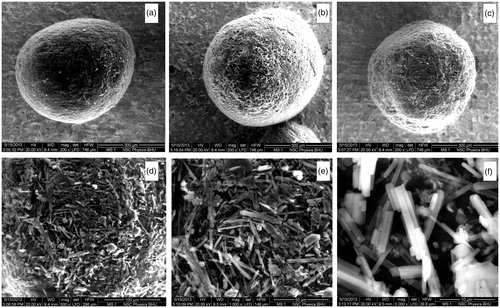
At very high magnification (500×, 1000 × and 5000×,) the needle-shaped crystals of drugs are clearly visible ().
Particle size
The particle size of microspheres is shown in . The particle size of microspheres varied with the type of polymer (EC or EU), drug/polymer ratio and polymer1/polymer 2 ratio (EU/EC). The average particle sizes ranged between 210 and 287 μm for EC microspheres, 220–301 μm for EU microspheres and 510–532 μm for combination of both polymers in different ratio. Earlier studies have also reported the relationship between particle size and polymer concentration (Ghaderi et al. Citation1996). Batches prepared with EC as polymer resulted in smaller microspheres than batches with EU as polymer.
Table II. Particle size and yield of different batches of LAM and INH microspheres (n = 3).
Yield
The percent yield of the microspheres ranged from 60 to 86% with EC, 56–83% with EU and 85–92% with the combination of EC and EU (). Yield determines the amount of final product obtained. Since, in all the cases yield was >56% it indicates that the solvent evaporation method can be considered efficient enough to microencapsulate the selected hydrophilic drugs.
Loose surface crystal study (% LSC)</H2
LSC study is a measure of the amount of drug adsorbed on the surface of the microspheres. The study was conducted to ascertain surface roughness and burst effect. The desired therapeutic action is attained by optimizing LSC and drug entrapped. Thus, burst effect is required for achieving therapeutic effective concentration of drug early. However, high amount of loose crystals on surface indicates lower drug entrapment inside the microspheres and will reduce the duration of sustained effect. The comparison between values of %EE and LSC are shown in . The LSC of microspheres ranged between 10% and 25%.
Entrapment efficiency
The %EE of the prepared microspheres was found to increase with increase in drug/polymer ratio (). The factors affecting particle size also influences %EE. It ranged from 48.30 to 80.38% for EC and 58.6 to 92.40% for EU and 68.41 to 88.33% for combination of both polymers. This could be attributed to more amount of drug present in the internal phase, increased viscosity of the medium, larger particle size and fast solidification of the formed microparticles. The increase in %EE was more prominent for EU than for EC.
In vitro release study
The cumulative percent of drug released from microspheres in phosphate buffer pH 7.4 are shown in . The drug release pattern showed biphasic behavior consisting of initial burst release phase in the first 2 h followed by a slow release phase extended up to 12 h for all the batches studied.
Figure 7. The cumulative percent of drug release from microspheres in phosphate buffer pH 7.4: (a) EC microspheres, (b) EU microspheres, and (c) combined microspheres.
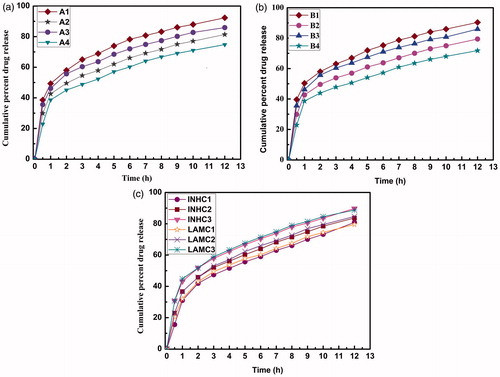
The burst effect followed sequence of A1 > A3 > A2 > A4 and B1 > B3 > B2 > B4. Batches with drug/polymer ratio of 1:1 showed higher burst release of drug compared to batches with drug/polymer ratio of 1:2 due to higher polymer content. This could be attributed to the increased thickness of the polymeric wall and longer diffusion path which may hinder diffusion up to some extent and more entrapment of drug inside microspheres.
Furthermore, release of drugs from EU microspheres (B series) was faster than from EC microspheres (A series). Although both series showed drug release extended up to 12 h, the batches A4 and B4 showed slow and controlled drug release with less than 80% of drug release in 12 h. From the batches A1–A4 and B1–B4 it was found that batches with drug/polymer ratio 1:2 (A2, A4, B2 and B4) showed optimum response in terms of in vitro drug release and EE.
Among the batches containing both drugs and both polymers (C1, C2 and C3) the drug release was solely affected by EC/EU ratio 1:1, 1:2 and 1:3, and LSC. The batch with 1:1 showed slower in vitro drug release than batch with 1:3. The batch C1 showed less cumulative percent drug release with minimized burst effect.
The in vitro release profiles were applied on various kinetic models in order to find out the mechanism of drug release and respective rate constants as given in . The in vitro release profiles from microspheres of different polymer-drug ratios were best fitted to Higuchi model with high correlation coefficient with R2 > 0.99 indicating drug release was proportional to square root of time and microspheres acted as matrix for drug release. The “n” value as obtained from the Korsmeyer–Peppas model was less than 0.5 for both EC and EU microspheres which indicate drug release was governed by non-Fickian mechanism (anamalous release). Similarly, the combined microspheres of both the drugs and polymers followed a non-Fickian mechanism of drug release as the “n” value varied from 0.281 to 0.352.
Table III. Kinetic analysis of in vitro release data.
In vitro cell viability
In vitro cell viability studies on Caco-2 cells showed a very good percent viability as compared to untreated control cells. The samples collected after 6 h showed maximum growth of cells which was 98% as compared to 100% of control. The samples collected after 12 and 24 h also showed cell growth above 95% (). These results indicate that the developed microspheres which are a combination of LAM + INH/EC + EU are totally safe for administration via oral route. The fluorescent microscopic images showed a good number of cells present in all sample treated wells. This implies that the prepared microspheres do not interfere with normal cell division and growth and exert no toxicity effect on gastrointestinal epithelia.
Discussion
Polymeric microspheres of EC and EU 100 containing INH and LAM were prepared by solvent evaporation technique. ICH class 3 organic solvents such as ethanol and acetone were selected because both have less toxic and negative genotoxic effects as compared to chlorinated solvents such as dichloromethane according to ICH Q3C (R4) guidelines. They are volatile with boiling point 78.37°C and 56°C, respectively, and can be eliminated totally with minimum contamination to the formulation. Spherical microparticles can be produced by joint effect of accelerated solvent evaporation depending on solvent volatility and polymer precipitation (Rosca et al. Citation2004). Also, both drugs and polymer could be easily incorporated in these solvents without any potentially toxic interaction.
For fabrication of microspheres, highly viscous saturated solution of polymers and drugs in organic solvents was prepared. When this organic phase was added in external oil phase containing surfactant, polymer–drug matrix immediately starts to precipitate because of insolubility in liquid paraffin and fast evaporation of organic solvents. The emulsion droplets containing drug get transformed into solid state due to hydrophobicity of polymers and evaporation of organic solvents during stirring (Pandav and Naik Citation2014). Due to decrease in solubility of drugs in external oil phase and hydrophobicity of polymers, the drug loss was reduced and enhanced encapsulation efficiency was obtained by this method.
Small amount of magnesium stearate was used as a droplet stabilizer to prevent droplet coalescence and aggregation in a suspension by reducing the interfacial tension between the dispersed phase and the dispersion medium (liquid paraffin). n-hexane was added as a non-solvent to the processing medium to solidify the formed microspheres (Lim and Wan Citation1998, Ueda et al. Citation1993). Span 80 is a better surfactant in terms of encapsulation efficiency, drug content, and particle size. Further, it has greater propensity to migrate toward the surface of polymeric microparticles.
EC and EU polymers are non-toxic, biocompatible, suitable for water-soluble drugs, soluble in wide range of organic solvents and insoluble in water, stable against active interactions, hydrolysis and oxidation, provide controlled and sustained release of the active ingredient, and have generally regarded as safe status (Rowe et al. Citation2009).
The particle size of microspheres prepared using EC alone were found to be smaller in size as compared to the particle size obtained using EU as a polymer. This trend in particle size under similar conditions may be attributed to more hydrophobic nature of EC than EU. The presence of quaternary ammonium groups in the structure of EU contributes toward its hydrophilicity (Rowe et al. Citation2009). Relatively, larger particles were obtained with mixture of EC and EU than single polymers. The effect could be attributed to higher polymer concentration in organic phase which increases the viscosity of feed and in turn leads to a less effective emulsifying process and increases the aggregation of microspheres (Ghaderi et al. Citation1996, Jha et al. Citation2011, Rajinikanth et al. Citation2008). The percent yield was found to be a direct function of concentration of polymer and ratio of drug and polymers. A higher percentage yield expresses the efficiency of processing conditions to obtain the product. Thus, lower yield indicates drug and polymer loss during processing conditions.
The amount of drug adsorbed on the surface depends on the polymer concentration. As the amount of polymer increases, more drugs get entrapped and hence less is available for adsorption. In fact, the LSC is a direct function of particle size and yield of microspheres. Smaller microspheres have more surface area available for drug adsorption. Further, higher yield also increases the surface adsorbed drug as more microsphere amount was obtained. The presence of loose drug crystals on the surface of microspheres was observed in different resolutions using SEM.
The findings of this research work indicate that higher the concentration of polymers available to encapsulate the drug and lesser the shearing stress to breakdown the formed particles, higher is the %EE. The results were in accordance with other reports (Cheu et al. Citation2001, Ghorab et al. Citation1990) Moreover, as the polymer concentration increased, the matrix for drug also increased and more drugs gets entrapped in the matrix hence higher encapsulation was observed in batches with higher drug polymer ratio than lower ratio. Batches with large particles showed increased %EE. However, EU-based microsphere batched exhibited higher % drug encapsulation than EC-based microspheres.
The prepared microspheres showed biphasic release pattern. The initial burst release is associated with the LSC as revealed by SEM that is adsorbed on to the surface of microspheres. The burst effect and drug release pattern of both the drugs was similar indicating that the drug release was unaffected by type of drug entrapped. Consequently, the drug release is affected by drug/polymer ratio and type of polymer (EC and EU). Both EU and EC are insoluble and non-swellable in aqueous environment. Therefore, batches prepared with EC have shown less burst effect than batches with EU due to its relatively higher hydrophobic nature. On increasing concentration of EU, the permeability of membrane increases and faster diffusion of drugs occur. However, EC forms hydrophobic and impermeable membrane. So, higher EC concentration slows the release of drug and provides desirable sustained and controlled release. Hence, the drug release can be manipulated by mixing the both polymers, and the intended release of both the drugs from the microspheres may be accommodated. The slow release characteristic shown for 12 h could reduce the dose and frequency of administration of both the drugs simultaneously. Combination batches (C1, C2 and C3) of both the drugs (LAM and INH) and polymers (EC and EU) were prepared by maintaining drug/polymer ratio (LAM + INH/EC + EU) of 1:2. Since, both drugs exhibited almost similar release profile from the microspheres for all batches (A, B and C series). This may be due to the reason that both LAM and INH have high degree of solubility in water and phosphate buffer pH 7.4. It could be inferred that the drug release from microspheres was independent of type of drug.
The in vitro cell viability study using MTT showed good biocompatibility of prepared microspheres. The results were supported by microscopic images showing a good number of cells present in all culture well. This suggests that the combination of both drugs and polymers do not react to give rise to a potentially harmful and toxic product which may harm gastric mucosa. So this combination could be safely used via oral route.
Conclusion
From the above results, it was concluded that low molecular weight and highly water-soluble drugs can be successfully encapsulated into EC and EU microparticles using o/o hydrophobic emulsion solvent evaporation method. The polymeric microspheres of combination of LAM and INH were successfully prepared, optimized and evaluated and were found to be promising for treatment of HIV-TB coinfection. The formulation containing drug/polymer ratio 1:2 and EU/EC of 1:1 showed optimum response with respect to in vitro release and drug EE. The microspheres exhibited Higuchi model release kinetics and maintained drug level for prolonged time which will minimize the side effects and minimize the frequency of dose which improves patient compliance. The in vitro cell viability studies established biocompatibility of the microspheres. In future, in vivo studies and various bacteriological studies are needed to support this research work.
Acknowledgements
GP acknowledges University Grants Commission (UGC) (New Delhi, India) for providing research fellowship.
Declaration of interest
The authors declare no conflicts of interest.
References
- Agnimitra D, Ipsita B, Sumit M, Moonmun D, Durga M, Si S, Barik B. 2010. Effect of chitosan on ethyl cellulose and Eudragit RS 100 microspheres. Asian J Chem. 22:5716.
- Ain Q, Sharma S, Khuller GK, Garg SK. 2003. Alginate based oral drug delivery system for tuberculosis: pharmacokinetics and therapeutic effects. J Antimicrob Chemother. 51:931–938.
- Balamuralidhara V, Kumar TP, Gupta NV, Gangadharappa H. 2012. pH-dependent gradient release microspheres of lercanidipine hydrochloride: in vitro and in vivo evaluation. J Drug Deliv Sci Technol. 22:493–499.
- Bulut E, Dilek M. 2014. Development and characterization of pH-sensitive locust bean gum-alginate microspheres for controlled release of ibuprofen. J Drug Deliv Sci Technol. 24:613–619.
- Burman WJ, Jones BE. 2001. Treatment of HIV-related tuberculosis in the era of effective antiretroviral therapy. Am J Respir Crit Care Med. 164:7–12.
- Cheu SJ, Chen RL, Chen PF, Lin WJ. 2001. In vitro modified release of acyclovir from ethyl cellulose microspheres. J Microencapsul. 18:559–565.
- Das MK, Rao KR. 2006. Evaluation of zidovudine encapsulated ethylcellulose microspheres prepared by water-in-oil-in-oil (w/o/o) double emulsion solvent diffusion technique. Acta Pol Pharm. 63:141–148.
- Dye C, Watt CJ, Bleed DM, Hosseini SM, Raviglione MC. 2005. Evolution of tuberculosis control and prospects for reducing tuberculosis incidence, prevalence, and deaths globally. JAMA. 293:2767–2775.
- Ghaderi R, Sturesson C, Carlfors J. 1996. Effect of preparative parameters on the characteristics of poly D, L-lactide-co-glycolide) microspheres made by the double emulsion method. Int J Pharm. 141:205–216.
- Ghorab MM, Zia H, Luzzi LA. 1990. Preparation of controlled release anticancer agents I: 5-fluorouracil-ethyl cellulose microspheres. J Microencapsul. 7:447–454.
- Jain S, Tiwary AK, Sapra B, Jain NK. 2007. Formulation and evaluation of ethosomes for transdermal delivery of lamivudine. AAPS PharmsSciTech. 8:249–257.
- Javed I, Ranjha N, Mahmood K, Kashif S, Rehman M, Usman F. 2014. Drug release optimization from microparticles of poly (E-caprolactone) and hydroxypropyl methylcellulose polymeric blends: formulation and characterization. J Drug Deliv Sci Technol. 24:607–612.
- Jha RK, Tiwari S, Mishra B. 2011. Bioadhesive microspheres for bioavailability enhancement of raloxifene hydrochloride: formulation and pharmacokinetic evaluation. AAPS PharmsSciTech. 12:650–657.
- Katakam P, Diaf SR, Dey B, Adiki SK, Chandu BR, Chowdary K. 2014. A comparative evaluation of drug release and permeability of ethylcellulose, cellulose acetate and Eudragit RS100 microspheres. Int J Sci Res Knowl. 2:75–82.
- Kim B, Hwang SJ, Park JB, Park HJ. 2002. Preparation and characterization of drug-loaded polymethacrylate microspheres by an emulsion solvent evaporation method. J Microencapsul. 19:811–822.
- Lim L, Wan L. 1998. Effect of magnesium stearate on chitosan microspheres prepared by an emulsification-coacervation technique. J Microencapsul. 15:319–333.
- Pandav S, Naik J. 2014. Preparation and in vitro evaluation of ethylcellulose and polymethacrylate resins loaded microparticles containing hydrophilic drug. J Pharma. 2014:904036. DOI: 10.1155/2014/904036.
- Pandey G, Mishra B. 2013. A new analytical Q-absorbance ratio method development and validation for simultaneous estimation of lamivudine and isoniazid. ISRN Spectroscopy. 2013:912376. DOI: 10.1155/2013/912376.
- Rajinikanth PS, Karunagaran LN, Balasubramaniam J, Mishra B. 2008. Formulation and evaluation of clarithromycin microspheres for eradication of Helicobacter pylori. Chem Pharm Bull (Tokyo). 56:1658–1664.
- Raviglione MC, Pio A. 2002. Evolution of WHO policies for tuberculosis control, 1948–2001. The Lancet. 359:775–780.
- Rosca ID, Watari F, Uo M. 2004. Microparticle formation and its mechanism in single and double emulsion solvent evaporation. J Control Release. 99:271–280.
- Rowe RC, Sheskey PJ, Quinn ME. 2009. Handbook of Pharmaceutical Excipients. London: Pharmaceutical Press.
- Sahoo S, Mallick A, Barik B, Senapati P. 2007. Preparation and in vitro evaluation of ethyl cellulose microspheres containing stavudine by the double emulsion method. Pharmazie. 62:117–121.
- Sharma S, Khuller G, Garg S. 2003. Alginate-based oral drug delivery system for tuberculosis: pharmacokinetics and therapeutic effects. J Antimicrob Chemother. 51:931–938.
- Sharomi O, Podder CN, Gumel AB, Song B. 2008. Mathematical analysis of the transmission dynamics of HIV/TB coinfection in the presence of treatment. Math Biosci Eng. 5:145.
- Timmins GS, Deretic V. 2006. Mechanisms of action of isoniazid. Mol Microbiol. 62:1220–1227.
- Ueda M, Nakamura Y, Makita H, Kawashima Y. 1993. Preparation of microcapsules masking the bitter taste of enoxacin by using one continuous process technique of agglomeration and microencapsulation. J Microencapsul. 10:461–473.
- Vaghani S, Jivani N, Serasia T, Vasanti S, Satish C, Patel, M. 2011. Preparation and characterization of 5-fu loaded microspheres of Eudragit and ethylcellulose. Ars Pharm. 2011;52:23–30.
- WHO. 2015. Global tuberculosis report. Geneva, Switzerland: WHO Press. http://www.who.int/tb/publications/global_report/en/.