Abstract
Objective: The present study was designed to develop PEGylated solid lipid nanoparticles (SLNs) of pentoxifylline (with increased t½) for the management of cardiac hypertrophy. Materials and methods: PEGylated SLNs were prepared by melt emulsification method and characterised by zeta potential, polydispersity index, entrapment efficiency, in vitro release and pharmacokinetic profile. Hypertrophy was assessed as increase in tumour necrosis factor-alpha, mean arterial blood pressure, LV protein content and left ventricular end diastolic pressure. Result: PEGylated SLNs (F19) with increased t½ were developed and characterised. F19 attenuates hypertrophy assessed in terms of parameters employed. Conclusion: Thus, PEGylated SLNs (F19) of pentoxifylline have enhanced t½ and consequently more significantly preclude cardiac hypertrophy as compared to pentoxifylline.
Introduction
Pathological cardiac hypertrophy is one of the major causes of congestive heart failure and is characterised by alteration in molecular, cellular and interstitial changes, i.e. changes in size, shape and function of the heart resulting from physiologic or pathologic stimuli (Lloyd-Jones Citation2010). Both pressure and volume overload can lead to structure adaptation, cardiac remodelling and cardiac dysfunction. Tumour necrosis factor-alpha (TNF-α), a proinflammatory cytokine has been implicated in pathogenesis of cardiovascular disorders such as myocarditis, ischemic heart disease and cardiac dysfunction. The persistent over expression of TNF-α has been suggested to be involved in cardiac remodelling and left ventricular dysfunction (Zhang et al. Citation2005b).
Pentoxifylline has been documented to inhibit TNF-α (Zhang et al. Citation2005a) decrease fibrosis and inhibit PDEs that prevent cell necrosis and hypertrophy in cardiac remodelling (Balakumar and Singh Citation2005, Champion et al. Citation2014). Beside TNF-α inhibition, pentoxifylline also modulates the endothelium via the down-regulation of cell surface adhesion molecule expression and chemokine production, and also via moderation of apoptotic pathways (Champion et al. Citation2014). Pentoxifylline is also reported to inhibit peripheral blood mononuclear cell proliferation (Moller et al. Citation1997). However, due to its short half-life (t½: 0.4–0.8 h) and less circulation time, pentoxifylline perfusion into hypertrophied heart is reduced. Pentoxifylline is metabolised in liver and erythrocytes and excreted by urine and faeces (Smith et al. 1996).
PEGylated solid lipid nanoparticles (SLNs) have the potential to increase circulation time and enhance permeation retention effect of drug. The addition of polyethylene glycol (PEG) to the surface of lipid nanoparticles increases hydrophilicity of the carrier system, meanwhile also reduces the recognition by MPS (mononuclear phagocyte system also called the reticuloendothelial system), which will ultimately reduce the clearance of drug from the bloodstream and hence increases half-life of the drug (Nunes and Barros Citation2015). Lipid nanoparticles have a size of 100–200 nm in diameter; apparently, this size range is favourable for extravasation to achieve so-called enhanced permeation retention effect. Lipid nanoparticles also have potential to target inflammation (Wang et al. Citation2014) and reduce the macrophage particle phagocytosis. Furthermore, lipid nanoparticles have a slower rate of dissociation, allowing retention of loaded drugs for a longer period of time and, eventually, achieving higher accumulation of drug at the target site, further allow drug to act specifically for long time.
PEGylation of nanoparticles may overcome the problem as reported with PEGylated microparticle of VEGF (vascular endothelial growth factor) which significantly decreased uptake of the nanocarriers by macrophages, compared with non-PEGylated microparticles (Simón-Yarza et al. Citation2013). PEGylation was also reported to increase efficacy of bacteriophage therapy by increasing half-life of the bacteriophage (Kim et al. Citation2008). Furthermore, specifically DSPE-PEG was also reported to increase circulation time of SLNs of Praziquantel (Zou et al. Citation2012). Moreover, PEGylation was also used for enhancing circulation half-life of recombinant human granulocyte colony stimulating factor (Zhai et al. Citation2009). These studies indicate the potential of PEGylation in enhancing circulation time of particles in body. Thus, the aim of the present study is to develop, characterise and investigate the potential of PEGylated SLNs of pentoxifylline in experimental cardiac hypertrophy.
Materials and methods
Pentoxifylline was obtained as a gift sample from Bakul Pharma (Mumbai, India), glycerol monostearate was purchased from Sigma-Aldrich (St. Louis, MO). Soybean lecithin (S75) was purchased from Lipoid GmbH (Ludwigshafen, Germany) and N-(carbonyl-methoxypolyethyl-eneglycol-2000)-1,2-distearoyl-sn-glycero-3-phosphoethanola-mine (mPEG2000-DSPE) was purchased from Genzyme Corporation (Cambridge, MA). All other reagents were of analytical grade.
Preparation of the PEGylated SLNs
PEGylated SLNs were prepared by melt-emulsification process with little modification as reported by Zhao et al. (Citation2012). Briefly, glycerol monostearate (55.3%), S75 (17.7%), mPEG2000-DSPE (15.6%) and pentoxifylline (11.4%) were mixed and dissolved in ethanol at 65 °C and solvent is evaporated slowly. Five percent glucose solution is used for dispersing the melted mixture under mechanical agitation. Emulsion was further homogenized for 10 min and sonicated for 5 min using ultrasonicator. Then, prepared lipid nanoparticles were separated by centrifuging at 2000 rpm for 10 min (3K-30 Sigma) (Zhao et al. Citation2012).
Optimisation of pentoxifylline-loaded SLNs
Optimisation of parameters including time length of homogenisation, sonication time, concentration of DSPE-PEG, S75 (as surfactant):lipid (GMS) ratio and drug (pentoxifylline) is done for obtaining desired particle size, polydispersity index (PDI) and entrapment efficiency of nanoparticles.
Characterisation of SLNs
Measurement of particle size and zeta potential
The mean particle size, PDI and zeta potential of the SLNs were determined in purified water at 23 °C by Zeta sizer (Beckman Coulter Counter, New Delhi, India). Particle size distribution studies were performed at a fixed refractive index of the respective formulation and size was measured in triplicate.
Determination of entrapment efficiency
Entrapment of pentoxifylline was determined by measuring the concentration of free drug (unentrapped drug). SLNs were centrifuged at 20,000 rpm for 20 min and supernatant was measured for the unentrapped drug with the help of UV spectroscopy (Wang et al. Citation2014). Entrapment efficiency of developed nanoparticles was calculated by using formula as given below:
Determination of the incorporated ratio of mPEG 2000-DSPE in SLNs
Free mPEG 2000-DSPE is separated by ultrafitration method. The amount of mPEG2000-DSPE was determined. Briefly, 10 ml of sodium nitrate–picrate solution (sodium nitrate 3.33 mol l−1, picric acid 0.02 mol l−1 and sodium hydroxide 0.1 mol l−1) and 5 ml of dichloromethane were added one by one to 5 ml of filtered sample solution. The mixture was vortexed for 1 min and then centrifuged at 3500 rpm for 15 min. After this organic layer was collected and measured spectrophotometrically at 365 nm. Total amount of mPEG 2000-DSPE in the formulation was determined using the same analytical procedure. The amount of mPEG2000-DSPE in the sample solution was calculated from the calibration curve of the standard mPEG 2000-DSPE solution (Zhao et al. Citation2012).
In vitro drug release
In vitro release of pentoxifylline from PEGylated SLNs was performed using the dialysis bag method reported by Venkateswarlu and Manjunath (Citation2004). The study was carried out using phosphate buffer pH 7.4 as a release medium at 37 °C. Briefly, 2 ml of formulation was taken in cellophane membrane sac in conical flask containing 100 ml of phosphate buffer pH 7.4. The temperature was maintained at about 37 °C in the shaking incubator with 100 rpm, conical flask was closed with the aluminium foil to prevent any loss during the experimental run. For sampling, 2 ml of liquid was withdrawn from the receptors compartment and were equally replaced with fresh release medium. Quantitative analysis was carried out immediately after withdrawal of samples using UV–Vis spectrophotometer under validated conditions. Percentage cumulative drug released was plotted against time (Venkateswarlu and Manjunath Citation2004).
Animals
Young male Wistar albino rats weighing 150–200 g were obtained from central animal house of ISF College of Pharmacy, Moga, Punjab, India and maintained on normal chow diet (Kisan Feeds Ltd., Chandigarh, India) and tap water ad libitum The animal protocol was approved and approved by the Institutional Animal Ethics Committee/(Approval no. 240) and the animal experiments were carried out in accordance with the Indian National Science Academy Guidelines for use and care of animals.
Pharmacokinetic study of pentoxifylline-loaded SLNs and pure pentoxifylline (HPLC)
Pharmacokinetic studies were carried out in male Wistar rats (150–200 g). The animals were divided into two groups, pure drug was administered to one group and other group received PEGylated nanoparticles. Both groups were injected intravenously with a dose of 3 mg kg−1, i.v. The blood samples were collected at predetermined time intervals into tubes with heparin for avoiding blood clotting. The collected blood samples were immediately centrifuged by cold centrifugation at 10,000 rpm for 5 min and the obtained plasma was subjected to analysis. Pentoxifylline plasma concentration was analysed using reversed-phase high-performance liquid chromatographic (RP-HPLC) method as reported by Chmielewska et al. (Citation2006). Briefly, the analyte was extracted from the plasma with dichloromethane after addition of 0.2 ml 1 M NaOH. C18 analytical column (250 mm × 4 mm i.d.) is used for HPLC separation with acetonitrile–water, 45:55 (v/v), as a mobile phase. Spectrophotometrically detection was performed at 275 nm. Calibration graphs were prepared by spiking blank plasma with known amount of drug (pentoxifylline) and linear plot was obtained from 50 to 1000 ng ml−1. Phenacetin was used as an internal standard. Recovery of the drug from plasma was 92.1%, and the detection limit was 50 ng ml−1 (Chmielewska et al. Citation2006).
Animal experimental protocol
Partial abdominal aortic constriction
Pathological cardiac hypertrophy was produced by partial abdominal aortic constriction (PAAC). Rats were anaesthetised with thiopentone sodium (35 mg kg−1 i.p.) and abdomen was exposed 3–4 cm. Aorta was identified between diaphragm and celiac artery. After that 4–0 silk suture was placed around the middle of aorta and tightened along with a 0.7-mm diameter needle. Needle was withdrawn to leave the vessel partially constricted and midline incision was sutured in layers. Neosporin antibiotic powder was applied locally on the sutured wound. Rats were allowed to recover and were kept under observation for 4 weeks. Sham operated animals were subjected to same surgical procedures except PAAC (Balakumar and Singh Citation2005; Singh et al. Citation2015).
Experimental design
Rats were divided into five groups and each group consisted of six animals. Group 1 (Sham control): surgery was performed to expose the abdominal aorta but it was not constricted. Group 2 (PAAC control): Abdominal aorta was exposed and partially constricted. Group 3 (PAAC–pentoxifylline): Rats were subjected to PAAC and were treated with pentoxifylline (3 mg kg−1 i.v.), which was started after 1 day of surgery and continued for 4 weeks. Group 4 (PAAC-PTX-SLN): Rats were subjected to PAAC and were treated with nanoparticles of pentoxifylline (3mg kg−1 i.v.) from the next day of surgery to 4 weeks. Group 5 (PAAC-ENAL): Rats were subjected to PAAC and were treated with standard drug enalapril (2mg kg−1 i.v) for 4 weeks ().
Pharmacological assessments
Hemodynamic assessments
For the measurement of cardiac function, a double-distilled water filled latex balloon was inserted through the mitral valve into the left ventricle, and left ventricular end diastolic pressure (LVEDP) was measured using pressure transducer. For measuring mean arterial blood pressure (MABP) (mmHg) rat tail was heated and MABP was measured by tail cuff method (BIOPAC System, Goleta, CA).
Morphological assessments
Left ventricle with interventricular septum and right ventricle weight were measured separately and expressed as mg g−1 of body weight. Left ventricle thickness was measured by dividing ventricle into three equal slices and wall thickness (mm) of each slice was noted at eight different points using an ocular micrometer. The mean value of all three slices was calculated (Balakumar and Singh Citation2005).
Biochemical assessments
Protein estimation. The left ventricle was stored at −80 °C in liquid nitrogen for quantitative estimation of biochemical parameters. The left ventricle was homogenised and protein content was determined spectrophotometrically at 750 nm by Lowry’s method and expressed as mg g−1 of left ventricular weight (Waterborg Citation2009).
TNF-estimation. TNF-α is estimated in left ventricular homogenate using the ELISA method (Krishgen Elisa kit of TNF-α determination).
Statistical analysis
Results were expressed as mean ± SD. Heart rate, MABP, LVEDP, left ventricular wall thickness (LVWT), left ventricular weight to body weight ratio (LVW/BW), RVW/BW and left ventricular TNF-α and protein levels were statistically analysed using one-way ANOVA followed by Tukey’s multiple range test. The p value <0.05 was considered to be statistically significant.
Results
Optimisation and characterisation of PEGylated SLNs
Many factors including composition, particle size, and zeta potential may influence in vivo characteristics of nanocarriers (Alexis et al. Citation2008). The mean particle size of final optimised filtered SLNs was between 200 and 400 nm, with a zeta potential of approximately −20 mV. Encapsulation efficiency of formulation F19 was found to be 91.9 ± 5.1%. First, surfactant:lipid ratio is optimised. After optimising the value of surfactant and lipid final concentration ratio is taken approximately (33:100 mg). Furthermore, other parameters are optimised for getting desired characteristics of formulated nanoparticles. All the parameters are optimised on the bases of particle size and PDI as shown in .
Table I. Optimisation of formulation.
Assessment of incorporation of mPEG2000-DSPE in SLNs
Measurement of DSPE-PEG incorporated in nanoparticles is important for ensuring long circulation of the SLNs in body as PEGylation prevents phagocytosis of nanoparticles (Chaudhari et al. Citation2012). Hence, incorporation of mPEG2000-DSPE in SLNs was determined by the picrate method. The calibration curve for the standard PEG2000-DSPE solution was A = 0.009x + 0.083 mL−1 (r2 = 0.997, where A is absorbance and x is mPEG2000-DSPE concentration), and the incorporation of mPEG2000-DSPE in final optimised formulation was 86.4 ± 4.48%. These results indicate that most of the mPEG2000-DSPE was incorporated into the SLNs.
In vitro studies
The in vitro drug release study of the pentoxifylline-loaded PEGylated SLNs F19 was done in PBS pH 7.4 using dialysis bag. The release study of the formulation was done thrice to ensure results. Obtained results show that 99.36 ± 3.6% of total pentoxifylline was released from PEGylated SLNs F19 in 9 h ().
In vivo pharmacokinetic study
PEGylation of nanocarriers has been reported to increase circulation time of nanoparticles in blood which ultimately increases time of the drug in body (Muller et al. Citation2000). Pharmacokinetic analysis of optimised formulation F19 was compared with pure drug. Final optimised formulation F-19 shows significant increase in circulation time as compared to pure drug. In case of formulation F19 pentoxifylline was detectable in plasma up to 24 h which shows an increase in circulation time of drug (). It also correlates well with obtained in vitro release data. The results show that nanoparticles had significantly improved the exposure, reduced the clearance, and raised the half-life of pentoxifylline ().
Figure 3. Pharmacokinetic profile of pure drug and PEGylated SLNs of pentoxifylline. NANO-PTX, PEGylated nanoparticles of pentoxifylline; PTX, pentoxifylline.
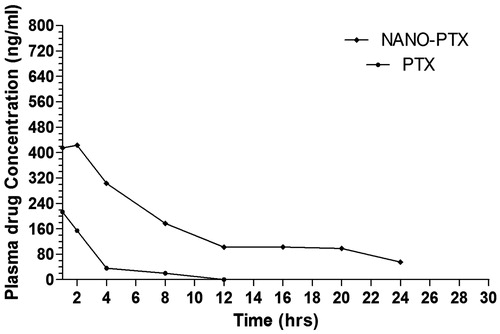
Table II. Pharmacokinetic parameters of pentoxifylline-loaded SLNs compared to pure drug.
Effect of PEGylated SLN’s of pentoxifylline on hemodynamic parameters
PAAC significantly increases LVEDP as compared to sham control. Pentoxifylline and PEGylated SLN’s F19 significantly reduce LVEDP as compared to PAAC control. However, PEGylated SLN’s F19 shows much significant reduction as compared to pure pentoxifylline ().
Figure 4. Effect of pharmacological interventions on LVEDP. @p < 0.05 versus Sham control, *p < 0.05 versus PAAC control, #p < 0.05 versus PAAC + PTX (3 mg kg−1). PAAC, partial abdominal aortic constriction; LVEDP, left ventricular end diastolic pressure; PTX, pentoxifylline, ENAL, enalapril; PTX + SLNs, nanoparticles of PTX.
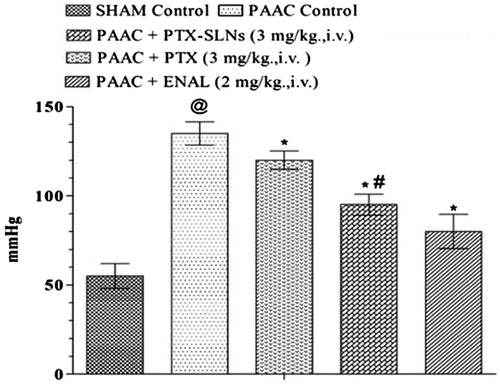
PAAC also shows significant increase in MABP, which was significantly attenuated by both pentoxifylline and PEGylated SLN’s F19. However, PEGylated SLN’s F19 shows much significant attenuation in MABP as compared to pure pentoxifylline ().
Figure 5. Effect of pharmacological interventions on MABP. @p < 0.05 versus Sham control, *p < 0.05 versus PAAC control, #p < 0.05 versus PAAC + PTX (3 mg/kg). PAAC, partial abdominal aortic constriction; MABP, mean arterial blood pressure; PTX, pentoxifylline; ENAL, enalapril; PTX + SLNs, nanoparticles of pentoxifylline.
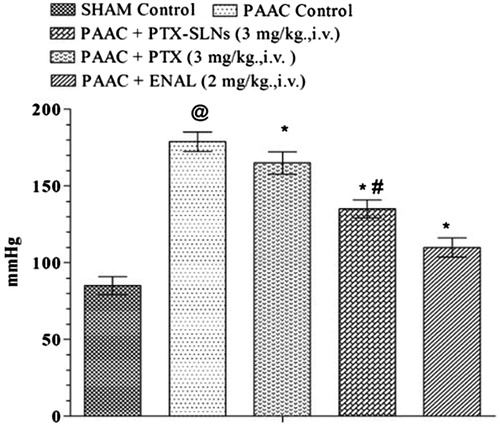
Effect of PEGylated SLN’s of pentoxifylline on morphological parameters
PAAC significantly increases left ventricular weight to body weight ratio (LVW/BW) (mg g−1) () and LVWT () as compared to sham control. Both pentoxifylline and PEGylated SLN’S F19 shows significant attenuation in LVW/BW (mg g−1) and LVWT; however, PEGylated SLN’s F19 shows much significant attenuation as compared to pentoxifylline.
Figure 6. Effect of pharmacological interventions on LVW/BW in rats. @p < 0.05 versus Sham control, *p < 0.05 versus PAAC control, #p < 0.05 versus PAAC + PTX (3 mg kg−1). PAAC, partial abdominal aortic constriction; LVW/BW, left ventricular weight/body weight; PTX, pentoxifylline; ENAL, enalapril; PTX + SLNs, nanoparticles of pentoxifylline.
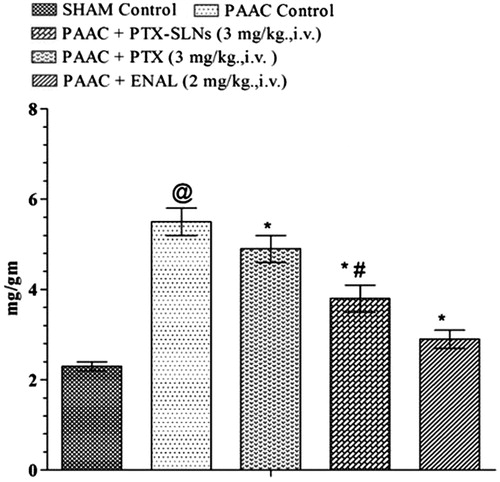
Figure 7. Effect of pharmacological interventions on LVWT. @p < 0.05 versus Sham control, *p < 0.05 versus PAAC control, #p < 0.05 versus PAAC + PTX (3 mg kg−1). PAAC, partial abdominal aortic constriction; LVWT, left ventricular wall thickness; PTX, pentoxifylline; ENAL, enalapril; PTX + SLNs, nanoparticles of Pentoxifylline.
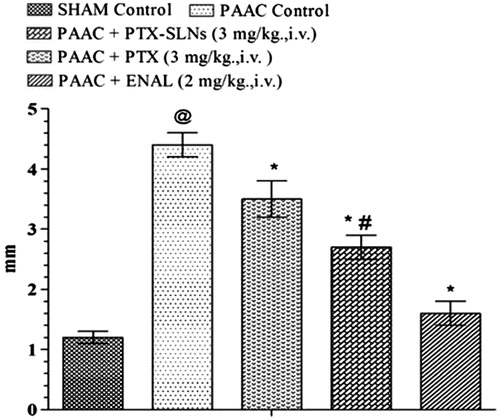
Effect of PEGylated SLN’s of pentoxifylline on biochemical parameters
Left ventricular protein content
Significant increase in left ventricular protein content was observed in PAAC control as compared to sham control. Both pentoxifylline and PEGylated SLN’s F19 show significant reduction in left ventricular protein content (). However, PEGylated SLN’s F19 shows much significant attenuation as compared to pure pentoxifylline.
Figure 8. Effect of Pharmacological interventions on left ventricular protein content. @p < 0.05 versus Sham control, *p < 0.05 versus PAAC control, #p < 0.05 versus PAAC + PTX (3 mg kg−1). PAAC, partial abdominal aortic constriction, protein content expressed as mg protein in left ventricle to left ventricular weight in gram; PTX, pentoxifylline; ENAL, enalapril; PTX + SLNs, nanoparticles of pentoxifylline.
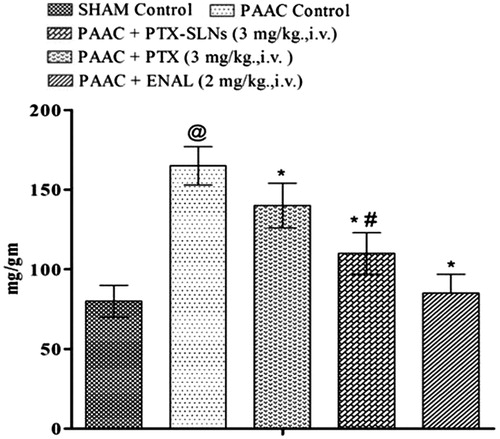
Left ventricular TNF-α level
TNF-α level was also increased in PAAC control and was shown to be significantly decreased by both pentoxifylline and PEGylated SLN’s F19. PEGylated SLN’s F19 shows much significant decrease in left ventricular TNF-α level as compared to pentoxifylline as represented in . Beneficial effect of PTX and PEGylated SLN’s of PTX may be due to inhibition of serine 32 in IKB factor that prevents the arrival into the nucleus of NF-KB and consequently inhibiting TNF-α production.
Figure 9. Effect of pharmacological interventions on left ventricular TNF-α level @p < 0.05 versus Sham control, *p < 0.05 versus PAAC control, #p < 0.05 versus PAAC + PTX (3 mg kg−1). PAAC, partial abdominal aortic constriction; PTX, pentoxifylline; ENAL, enalapril; PTX + SLNs, nanoparticles of pentoxifylline.
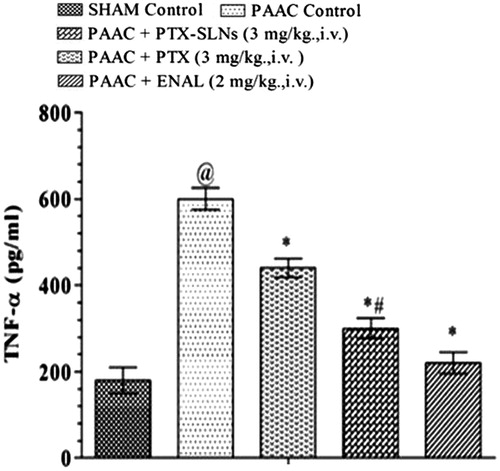
Discussion
Deficient perfusion to hypertrophied tissue triggers inflammatory cascade and further aggravates cardiomyocyte death. Inflammatory cascade stimulate various cytokines involved in the necrosis and apoptosis of cardiac myocyte. TNF-α is one of the cytokines, which is documented to be involved in cardiomyocyte necrosis. Pentoxifylline has been documented to inhibit proinflammatory cytokines, namely TNF-α, IL-1, IL-6, IL-12 decreases oxidative stress mediated fibrosis and inhibit PDEs that prevent cell necrosis and hypertrophy in cardiac remodelling (Champion et al. Citation2014, Moller et al. Citation1997). However, due to its short half-life (t½: 0.4–0.8 hours) and less circulation time, pentoxifylline perfusion into hypertrophied heart is reduced. PEGylated SLNs have been reported to have high circulation time in body. Thus, in the present PEGylated SLNs of pentoxifylline are proposed to have long circulation time in blood which will increase half-life of drug and may have high retention to hypertrophied and inflamed cardiomyocyte that ultimately enhances the action of pentoxifylline in attenuating cardiac remodelling. PEGylated nanoparticles were prepared by melt emulsification method. Optimisation of formulation was done by variating surfactant:lipid ratio, homogenisation time and speed, sonication time and concentration of DSPE-PEG and drug (pentoxifylline) for getting desired particle size and PDI as shown in . First, surfactant:lipid ratio was optimised from F1 to F3 in which formulation F2 with ratio of 1:3 was best emulsified formulation. However, further increase in fraction of lipid leads to increase in particle size, which could be related to a viscosity increase in the dispersion, leading to higher surface tension and thus larger particle size. Moreover, this increase in particle size might occur due to increased collision and aggregation of the nanoparticles (Zhang et al. Citation2013). Furthermore, homogenisation time was optimised from F4 to F8. Formulation F7 with homogenisation time of 10 min was found to be the most adept formulation. However, in case of other formulations increase in homogenisation time increases particle size which may be due to increased temperature and accumulation of particles. Homogenisation speed also plays an important role in formulation which was optimised from F8 to F11, in which F10 with speed of 8000 rpm was found to be the best formulation with best values as shown in further increase in homogenisation speed leads to increase in size which may be due to aggregation of nanoparticles (Parhi and Suresh Citation2010). Sonication, an important step of preparation was also optimised from F11 to F14 in which F13 with sonication time of 5 min was found to be with smallest particle size and further sonication increases the particle size which might be a cause of breakage of lipid nanoparticles by over sonication. After that concentration of drug and DSPE-PEG was optimised on the basis of particle size and entrapment of drug as shown in . Among these formulations, F16 was found to be the best formulation without drug and F19 was the finally optimised formulation with desired size, PDI and entrapment efficiency of drug.
SLNs have been reported to have sustained in vitro release and enhance the oral bioavailability of pentoxifylline (Varshosaz et al. Citation2010). In the present study, in vitro release of formulation (F19) shows the controlled drug release with release time of 10 h. Furthermore, various pharmacokinetic studies of PEGylated nanoparticles had been reported for increasing circulation time of drugs as reported in case of PEGylated SLNs of siRNA where circulation of nanoparticles was increased by PEGylation (Lobovkina et al. Citation2011). Pharmacokinetic study of finalised formulation F19 in rats shows an increase in half-life of pentoxifylline which may be due to increased circulation of PEGylated nanoparticles (Lima et al. Citation2004).
Furthermore, the effect of final formulation F19 was investigated in PAAC-induced cardiac hypertrophy in Wistar rats. PAAC increases left ventricular (LV) weight to body weight ratio, left ventricular wall thickness and left ventricular protein content which have been observed to increase in pressure induce cardiac remodelling (Li et al. Citation2003). MABP has been noted to increase gradually and attain peak level after 4 weeks of PAAC. The marked increase in MABP in PAAC model may be due to pathological cardiac hypertrophy as reported recently (Kuwahara et al. Citation2004). LVEDP was also increased in PAAC-induced hypertrophy which may be due to reduced left ventricular function (Mielniczuk et al. Citation2007). PAAC was shown to increase left ventricular TNF-α levels as reported recently in case of pathological cardiac hypertrophy (Sun et al. Citation2007). PEGylated SLN (F19) treatment shows much significant reduction in left ventricular weight to body weight ratio, left ventricular wall thickness, MABP, LVEDP, left ventricular protein content and left ventricular TNF-α levels. These results indicate increased beneficial effect of PEGylated SLNs F19 as compared to pure drug. However, enalapril has more significant effect on parameters employed than the PTX and PSLN-PTX. Enalapril also reduces TNF-α level more significantly as compared to PTX and PSLN-PTX possibly due to inhibition of ACE and ANG II mediated stimulation of TNF-α Production. Pentoxifylline is not the drug-of-choice for cardiac hypertrophy; however, it is proposed that it may be used as adjuvant treatment in combination with enalapril and other recommended standard drug therapy. Thus, investigation of combined effect of enalapril with PTX and PSLN-PTX may be done in further studies.
The present study entails that nanoparticles of pentoxifylline show much significant attenuation of cardiac remodelling as compared to pure drug which may be due to increased circulation time of PEGylation SLNs which ultimately increases the half-life of drug in the body. Furthermore, increased retention and permeation of PEGylated SLNs of pentoxifylline to the hypertrophied and inflamed myocyte may also be the reason of increased beneficial effect of PEGylated SLNs of pentoxifylline.
Conclusion
Thus, it may be concluded that PEGylated SLNs of pentoxifylline increases the circulation time and half-life of drug and significantly preclude pathological cardiac remodelling as compared to pentoxifylline.
Acknowledgements
We express sincere thanks to Mr. Praveen Garg honourable chairman, ISF College of Pharmacy, Moga, Punjab, India, for providing needed facilities for the study.
Declaration of interest
The authors report no declarations of interest. The authors alone are responsible for the content and writing of the paper.
References
- Alexis F, Pridgen E, Molnar LK, Farokhzad OC. 2008. Factors affecting the clearance and biodistribution of polymeric nanoparticles. Mol Pharm. 5:505–515.
- Balakumar P, Singh M. 2005. The possible role of TNF-alpha in physiological and pathophysiological cardiac hypertrophy in rats. Iran J Pharmacol Ther. 4:138–142.
- Champion S, Lapidus N, Cherié G, Spagnoli V, Oliary J, Solal AC. 2014. Pentoxifylline in heart failure: a meta‐analysis of clinical trials. Cardiovasc Ther. 32:159–162.
- Chaudhari KR, Ukawala M, Manjappa AS, Kumar A, Mundada PK, Mishra AK, et al. 2012. Opsonization, biodistribution, cellular uptake and apoptosis study of PEGylated PBCA nanoparticle as potential drug delivery carrier. Pharm Res. 29:53–68.
- Chmielewska A, Konieczna L, Plenis A, Lamparczyk H. 2006. Quantitative determination of pentoxifylline in human plasma. Acta Chromatogr. 16:70–79.
- Kim KP, Cha JD, Jang EH, Klumpp J, Hagens S, Hardt WD, et al. 2008. PEGylation of bacteriophages increases blood circulation time and reduces T‐helper type 1 immune response. Microbial Biotechnol. 1:247–257.
- Kuwahara F, Kai H, Tokuda K, Takeya M, Takeshita A, Egashira K, Imaizumi T. 2004. Hypertensive myocardial fibrosis and diastolic dysfunction another model of inflammation? Hypertension. 43:739–745.
- Li J, Li P, Feng X, Li Z, Hou R, Han C, Zhang Y. 2003. Effects of losartan on pressure overload‐induced cardiac gene expression profiling in rats. Clin Exp Pharmacol Physiol. 30:827–832.
- Lima V, Vidal FD, Rocha FAC, Brito GAC, Ribeiro RA. 2004. Effects of tumor necrosis factor-α inhibitors pentoxifylline and thalidomide on alveolar bone loss in short-term experimental periodontal disease in rats. J Periodontol. 75:162–168.
- Lloyd-Jones D. 2010. Heart disease and stroke statistics—update: a report from the American Heart Association. Circulation. 121:e46–e215.
- Lobovkina T, Jacobson GB, Gonzalez-Gonzalez E, Hickerson RP, Leake D, Kaspar RL, et al. 2011. In vivo sustained release of siRNA from solid lipid nanoparticles. ACS Nano. 5:9977–9983.
- Mielniczuk LM, Lamas GA, Flaker GC, Mitchell G, Smith SC, Gersh BJ, et al. 2007. Left ventricular end‐diastolic pressure and risk of subsequent heart failure in patients following an acute myocardial infarction. Congest Heart Fail. 13:209–214.
- Moller DR, Wysocka M, Greenlee BM, Ma X, Wahl L, Trinchieri G, Karp CL. 1997. Inhibition of human interleukin‐12 production by pentoxifylline. Immunology. 91:197–203.
- Muller RH, Mader K, Gohla S. 2000. Solid lipid nanoparticles (SLN) for controlled drug delivery – a review of the state of the art. Eur J Pharm Biopharm. 50:161–177.
- Nunes S, Barros A. 2015. The use of coating agents to enhance liposomes blood circulation time. J Mol Pharm Org Process Res. 3:e120.
- Parhi R, Suresh P. 2010. Production of solid lipid nanoparticles—drug loading and release mechanism. J Chem Pharm Res. 2:211–227.
- Simón-Yarza T, Formiga FR, Tamayo E, Pelacho B, Prosper F, Blanco-Prieto MJ. 2013. PEGylated-PLGA microparticles containing VEGF for long term drug delivery. Int J Pharm. 440:13–18.
- Singh AP, Singh R, Krishan P. 2015. Ameliorative role of gemfibrozil against partial abdominal aortic constriction-induced cardiac hypertrophy in rats. Cardiol Young. 25:725–730.
- Smith RV, Waller ES, Doluisio JT, Bauza MT, Puri SK, Ho I, Lassman HB. 1986. Pharmacokinetics of orally administered pentoxifylline in humans. J Pharm Sci. 75:47–52.
- Sun M, Chen M, Dawood F, Zurawska U, Li JY, Parker T, et al. 2007. Tumor necrosis factor-α mediates cardiac remodeling and ventricular dysfunction after pressure overload state. Circulation. 115:1398–1407.
- Varshosaz J, Minayian M, Moazen E. 2010. Enhancement of oral bioavailability of pentoxifylline by solid lipid nanoparticles. J Liposome Res. 20: 115–123.
- Venkateswarlu V, Manjunath K. 2004. Preparation, characterization and in vitro release kinetics of clozapine solid lipid nanoparticles. J Control Release. 95: 627–638.
- Wang Z, Li J, Cho J, Malik AB. 2014. Prevention of vascular inflammation by nanoparticle targeting of adherent neutrophils. Nat Nanotechnol. 9:204–210.
- Waterborg JH. 2009. The Lowry Method for Protein Quantitation. The Protein Protocols Handbook. New York: Humana Press, pp. 7–10.
- Zhai Y, Zhao Y, Lei J, Su Z, Ma G. 2009. Enhanced circulation half-life of site-specific PEGylated rhG-CSF: optimization of PEG molecular weight. J Biotechnol. 142:259–266.
- Zhang C, Gu C, Peng F, Liu W, Wan J, Xu H, et al. 2013. Preparation and optimization of triptolide-loaded solid lipid nanoparticles for oral delivery with reduced gastric irritation. Molecules. 18:13340–13356.
- Zhang M, Xu YJ, Saini HK, Turan B, Liu PP, Dhalla NS. 2005a. Pentoxifylline attenuates cardiac dysfunction and reduces TNF-∝ level in ischemic-reperfused heart. Am J Physiol Heart Circ Physiol. 289:H832–H839.
- Zhang M, Xu YJ, Saini HK, Turan B, Liu PP, Dhalla NS. 2005b. TNF-α as a potential mediator of cardiac dysfunction due to intracellular Ca2+-overload. Biochem Biophys Res Commun. 327:57–63.
- Zhao Y, Wang L, Yan M, Ma Y, Zang G, She Z, Deng Y. 2012. Repeated injection of PEGylated solid lipid nanoparticles induces accelerated blood clearance in mice and beagles. Int J Nanomed. 7:2891–2900.
- Zou Y, Ding J, Zheng Z, Zhang L, Ma N. 2012. Preparation and in vitro–in vivo evaluation of long-circulating solid lipid nanoparticles loaded with praziquantel. Chin J Pharm. 43:438–442.