Abstract
Nanotechnology and its applications in biomedical sciences principally in molecular nanodiagnostics are known as nanomolecular diagnostics, which provides new options for clinical nanodiagnostic techniques. Molecular nanodiagnostics are a critical role in the development of personalized medicine, which features point-of care performance of diagnostic procedure. This can to check patients at point-of-care facilities or in remote or resource-poor locations, therefore reducing checking time from days to minutes. In this review, applications of nanotechnology suited to biomedicine are discussed in two main class: biomedical applications for use inside (such as drugs, diagnostic techniques, prostheses, and implants) and outside the body (such as “lab-on-a-chip” techniques). A lab-on-a-chip (LOC) is a tool that incorporates numerous laboratory tasks onto a small device, usually only millimeters or centimeters in size. Finally, are discussed the applications of biomedical nanotechnology in improving “lab-on-a-chip” techniques.
Background
Nanotechnology is not a single discipline or technology, but it include several technologies that cross sectors, such medicine, nanomaterials (NMs), fabrication, devices, communications, electronics, and energy. Nowadays, scientists focused on two main class of applications of nanotechnology particularly suited to biomedicine: biomedical applications for use inside the body (such as drugs, diagnostic techniques, prostheses, and implants) and biomedical applications for use outside the body (such as “lab-on-a-chip” techniques, which are appropriate for testing blood and other samples, and for inclusion in analytical instruments for research and development or R&D on new drugs). In brief, both class intend to study and clarify eight topics for research in the coming years: (1) applications of nanotechnology in therapy, (2) biomimetic nanostructures, which are synthetic products developed from an understanding of biological systems, (3) synthesis and use of nanostructures, (4) devices for early detection of disease, (5) instruments for studying individual molecules, (6) biological nanostructures, (7) nanotechnology for tissue engineering, and (8) the electronic–biological interface (Malsch Citation2002).
Biomedical nanotechnology applications for use inside the body
The aim of many companies for biomedical nanotechnology applications for use inside the body are developing nanotechnology applications for important problems such as anticancer drugs, implanted insulin pumps, and gene therapy as well as prostheses and implants that include nanostructured materials.
Biomedical nanotechnology in nanodiagnostic techniques
Nanotechnology is the formation and employment of nanomaterials, nanodevices, and nanosystems through the control of matter on the nanometer length scale and its usage in life sciences, which is known as “nanobiotechnology” (Jain Citation2005a). Scientists are occupied in the synthesis of organic, inorganic, and hybrid nanomaterials for the use in nanotechnology applications, such as the development of novel nanoanalytical techniques. Nanodiagnostics technologies are growingly popular in the field of medical diagnostics. Some example of nanodiagnostics technologies are: nanoparticle biolabels, nanoscale visualization, biochips/microarrays, nanoproteomic-based diagnostics, nanoparticle-based nucleic acid diagnostics, nanoparticle-based immunoassays, DNA nanomachines, nanobiosensors, biobarcode assays, and nanopore technology (Jain Citation2007).
Nanofluidic arrays and protein nanobiochips are examples of devices that incorporate nanotechnology-based biochips and microarrays. The aim of the design of these chips is to interact with cellular constituents with higher specificity (Saini et al. Citation2010). Nanofluidic devices are more promising for isolation and analysis of individual biomolecules, such as DNA, which can lead to new detection schemes for cancer (Jain Citation2005a). In general, research in the coming years on nanofluidic technologies will focus of applications in personalized medicine, systems biology, drug development, clinical research, and pathogen detection.
Nanotechnology has been used for detection of biomarkers. The high surface areas and physicochemical characteristics of nanoparticles make them promising candidates for generating biomarker-harvesting systems (selectively bind to a subset of biomarkers and sequester them for afterward analyzing using high-sensitivity proteomic tests) (Geho et al. Citation2006, Jain Citation2003).
There are many nanoparticles which widely have been used for diagnostics, such as gold nanoparticles (assemble onto a sensor surface only in the presence of a complementary target, which can detect millions of different DNA sequences simultaneously) (Jain Citation2003), QuantumDots (inorganic fluorophores which can emit fluorescent light when irradiated with low energy light) (Kubik et al. Citation2005), and magnetic nanoparticles (such as iron nanoparticles) (Maeda et al. Citation2006).
In situ pathogen quantification can detect a single bacterium within 20-min using a bioconjugated nanoparticle-based bioassay (Zhao et al. Citation2004). Silver nanorods (which significantly amplify the signal) by applying a spectroscopic assay based on SERS, has been used for rapid detection of viruses with a high degree of sensitivity and specificity (Shanmukh et al. Citation2006). This method makes available rapid diagnostics (about 60 s) for detection and characterization of viruses (that generate reproducible spectra) without viral manipulation.
Biomedical nanotechnology in drug-based approaches
Some of traditional drugs (used for the treatment and management of chronic diseases such as cancer, asthma, hypertension, HIV, and diabetes) can be toxic in vivo or in vitro but biomedical nanotechnology approaches and nano-sized carriers can deliver them out of healthy tissue and keep old drugs to new places. The ability to produce nanodrug carrier particles to exact specifications, and to organize their shape at the nanoscale with great exactness, empowers researchers to organize their function. Nanoparticles with carefully controlled size, chemistry, surface charge, and other important properties can deliver drugs to place of interest and provide new functions. Nano-engineered drug delivery systems can give selectively into place of interest such as cancerous tissue, or keep the drugs (which they carry) from being destroyed before they reach their destination, without causing side effects or inducing resistance (Bourzac Citation2012). It is also producing therapies that were before believed impossible, for example drugs that can change their properties according on that target proteins once deemed undruggable, or where they are in the body. Nanoparticulate drug delivery system, such as micelles and liposomes, are frequently applied to rise the efficiency of drug and DNA delivery and targeting (Torchilin Citation2005a, Citation2005b).Some peptides and proteins have also been applied for the intracellular delivery of nanoparticulates (quantum dots (Eatemadi et al. Citation2014a, Citation2014b), iron oxide nanoparticles (Eatemadi et al. Citation2015, Seidi et al. Citation2014), and liposomes (Daraee et al. Citation2014a,Citationb)), small drug molecules, and large molecules (enzymes and DNA) (Tachibana et al. Citation1998, Torchilin et al. Citation2003, Vives et al. Citation1997, Zhao et al. Citation2002). Another class of therapeutics polymers are polymer–drug conjugates that consists of a water-soluble polymer that is chemically conjugated to a drug through a biodegradable linker (Mellatyar et al. Citation2014). Due to a low aqueous solubility and a broad tissue distribution profile of small molecule drugs, especially hydrophobic compounds, administration of the free drug may result in serious side effects. Therefore, to increase their aqueous solubility, modify their tissue distribution profile, enhance their plasma circulation half-life, and retarding degradation/metabolism/excretion rates of these compounds, they must conjugate to hydrophilic, biocompatible polymers. In 1994, the introduction of PEG-l-asparaginase (used for the treatment of acute lymphoblastic leukemia) as a FDA-approved anti-cancer treatment was the first practical use of polymer therapeutics (Veronese and Pasut Citation2005).
In one study, Lee et al. (Torchilin Citation2012) synthesized the biotin-tagged pH-sensitive polymeric micelles based on a mixture of PLA-b-PEG-b-PHis-biotin [PLA = poly (l-lactic acid)] and PEG-b-PHis block copolymers that targeting moiety, biotin, was internalized to cancer cells by ligand–receptor interactions, lowered pH (<6.5) destabilized the carrier resulting in a burst release of the loaded drug.
Lukyanov et al. (Lukyanov et al. Citation2004), produced a pH-degradable PEG-b-phosphatidylethanolamine (PE) liposome had anti-myosin monoclonal antibody as well as TAT or biotin attached on its surface.
There are different nanoparticulate drug delivery systems which have widely been used such as liposomes, microemulsions, nanoparticles and etc. these systems may be synthesized by natural polymers (Starch, Chitosan, and Gelatin), synthetic polymer (poly ethylene glycol), or combination.
Biomedical nanotechnology in prostheses and implants-based approaches
Nanotechnology provides people the ability to overcome impairments, through better prosthesis, implants materials, therapies, and so on.
Nanotechnology has been used to improve a broad range of implants and prosthesis (Chaudhry et al. Citation2006, Loomis Citation2002), because it is have potential to making them biocompatible (Bullis Citation2006), cleaner (i.e., antimicrobial coatings), stronger, to have more efficient (i.e., consuming less power) and better performance (i.e., increase capacity and speed processor by allowing a greater number of electrodes in current implants/prostheses).
Some of the problems of existing materials, for example preventing the protein of cells from assemble on sensors, which can lead to the prosthesis to not work properly, stop working or needing replacement, can be overcome using nanotechnology (Lee et al. Citation2008).
Using nanotechnology, scientists create nanofibers that are being used to allow growth of tissue (Bullis Citation2006) and the nanocircuits that are being used to improve advanced wheelchairs (able to know its environment, sense where they are and where they must go) (Benson and Barrett Citation2005).
Nanotechnology will help in the generation of new prosthetics, such as emotional social intelligence prosthesis (Lombardi Citation2006), memory implants (Radford Citation2003), and molecular prosthetics – nano-scale components that can repair or replace defective cellular components (Cabrera Citation2009).
“Lab-on-a-chip” techniques
A lab-on-a-chip (LOC) is a tool that incorporates numerous laboratory tasks onto a small device, usually only millimeters or centimeters in size. It is fundamentally a network of wells and channels that are scraped onto polymer or silicon supports, coupled with nanostructured, biofunctionalized surfaces to detect target analytes from biological serum. Microfluidics is a broader term used for LOC. In the microfluidic systems, electrokinetic or pressure forces move small volumes of liquid over a stationary capture/detection region (e.g., arrays of microcantilevers (McKendry et al. Citation2002), carbon nanotubes (Claussen et al. Citation2009), or graphene petals (Claussen et al. Citation2012)) in an accurately controlled approach through the wells and channels. Frequently employed fluids and liquids include bacterial cell suspensions, whole blood samples, antibody or protein solutions, and various reagents and buffers (Phurimsak et al. Citation2014). Same systems have been grown or fabricated onto the microchip. Biorecognition agents (e.g., single-stranded DNA, antibodies, enzymes) physically/chemically immobilized on the sensor detection region (detector) bind with target analyte within the moving media to initiate an electrical/optical signal transduction (using transducer).
The LOC enables sample dilution, mixing, handling, electrophoresis, and chromatographic detection, staining, and separation on a single, integrated system. The main advantages of the LOC are speed of analysis, ease of use, low reagent and sample consumption, high-throughput processing, and high reproducibility due to automation and standardization (Hong and Quake Citation2003, Janasek et al. Citation2006, Whitesides Citation2006).
For the most part, “lab-on-a-chip” systems consist of elements that present physical ways of directing liquid, most frequently in form of microfluidic channel networks. The LOC consisted of many part such as: (1) sample input, (2) reagent supply, (3) pumps, (4) sensor readout, (5) drain, (6) waste output, (7) septum, (8) mixing channels, and (9) liquid reagent storage (). The design of these structures should be permanent during improvement of the LOC system and is usually not necessary to be variable.
Figure 1. Lab-on-a-chip devices for point of care applications (reprinted from (Castillo-León and Svendsen Citation2014)).
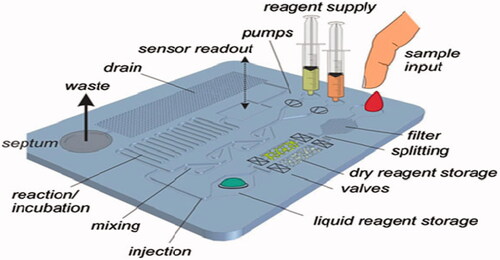
illustrates a common structure of a LOC device with diverse passive and active structures. LOC-based techniques will carry on to be the most probable technological driver to convert the point-of-care diagnostic industry. The employ of LOC spans a variety of potentials: adapting LOC components into an existing non-LOC POC technology, developing completely new LOC concepts, or re-working current LOC concepts into a practical device. There are unofficial list of companies working on microfluidics-based point-of-care diagnostic test, such as Abaxis, Advanced Liquid Logic, Alere (formerly Inverness), Atonomics, Biosite (Alere), Biosurfit, and many other companies. The commercially microfluidic point-of-care diagnostic devices are well reviewed in reference (Chin et al. Citation2012). illustrates some examples of commercially available lab-on-a-chip.
Biomedical nanotechnology in “lab-on-a-chip” techniques
The state-of-the-art in LOC technology presents a standard shift for medical diagnostics. Instead of sending test samples to other laboratories for analysis, healthcare services can use LOC technology to check patients at point-of-care facilities or in remote or resource-poor locations, therefore reducing checking time from days to minutes. This low diagnostic time is importantly essential for time-consumption medical care and treatments such as pinpointing biological warfare agents from an exposed solider, diagnosing a viral infection in an elderly immune-compromised patient or detection early the initiation (start) of treatment and preventing complications related to the infection or disease.
Synthetic nanomotors provide a unique solution to organize and test biological samples on LOC tools (Wang and Gao Citation2012). Receptor-functionalized nanomotors (which moved using catalytic decomposition of hydrogen peroxide), have potential to capturing and isolating biological targets (such as pancreatic cancer cells and E. coli) from untreated biological samples (Balasubramanian et al. Citation2011, Campuzano et al. Citation2011, Sanchez et al. Citation2011). These micro/nanomotors could be immobilized on micro fluidic-based tools to cut off target analyte or fracture cellular tissue from unprocessed biological media such as a piece of tissue biopsy. To eliminate the need for separate nanostructured, biodetection regions grown or immobilized within the structure, Incorporating Förster Resonance Energy Transfer (FRET)-based nanosensors may reduce microchip design. Enzyme–substrate interaction, antibody–antigen binding, and DNA hybridization would all occur rapidly in solution, which can cause diffusion-limited kinetics and bound/free reagent separation and washing steps (Varghese et al. Citation2010). Achieving an optofluidic system (FRET-based biodetection scheme) may hold using of luminescent semiconductor Quantum Dots (QDs) that is capable of multiplexed bioanalytical analysis and highly-sensitive. These systems (nanocrystalline materials) have promising properties well-designed to optical biosensing including size-tunable photoluminescence (PL), high quantum yields, enhanced avidity/sensitivity to biomolecular probes, and biosensing and resistance to photobleaching (Algar et al. Citation2011, Gill et al. Citation2008).
Conjugating fluorescent dye-labeled biological probes with QDs can present FRET biosensors that are advanced to traditional biosensors in several ways. The probability of FRET between a QD and dye is significantly enhanced as multiple FRET “lanes” are introduced, when connecting multiple dye-acceptors around the central QD in a centrosymmetrical fashion and using this, can be greatly enhanced The FRET efficiency of these QD-dye bioconjugates (Gill et al. Citation2008, Varghese et al. Citation2010).
A wide range of biomarkers can detect using QD-fluorescent dye bioconjugates through both increases and decreases in FRET (donor/acceptor) efficiencies. Using QD-dye conjugates, monitoring estrogen receptor β, an important diagnostic biomarker for breast cancer, created an enhancement in FRET efficiency (Wei et al. Citation2006), but a decrease in FRET efficiency occurred via the toxins active proteolytic cleavage of the linker between the fluorophores, when detecting botulinum neurotoxins, extremely potent bacterial toxins that contaminate food supplies, monitor (Sapsford et al. Citation2011). Therefore, incorporating Such FRET-based QD-dye conjugates in LOC microfluidic devices could rapidly attach to analyte of interest in solution for a real-time, rapid bioanalytical analysis that is also simply quantifiable.
Lab-on-a-chip (LOC) can be used for detecting and monitoring disease-causing organisms, such as viruses and bacteria (Fernandes et al. Citation2014). LOC has been used for analysis of and nucleic acid immunoassays (the binding of antibodies to antigens to identify and measure certain substances, such as hormones) (Prakash et al. Citation2014).
Lab-on-a-chip (LOC) has also been used for monitoring disease states, clinical diagnostics (the detection of disease) (Millington et al. Citation2014), and biochemical analysis by quantitative (numerical) and qualitative (i.e., determining the nature) analysis of chemical substances or proteins from the sample of interest (e.g., cancer markers in blood) (Mirasoli et al. Citation2014). LOC can be used for analysis of proteins, DNA, etc., as in protein microarrays (Dixit and Aguirre Citation2014) and DNA microarrays (Ohlander Citation2014). A microarray is a collection of miniaturized tests located on a membrane that allows numerous tests to be carrying simultaneously, or with the purpose of achieve higher throughput rate. Gene expression microarrays method has been used to predict patient outcomes, to classify cancerous tumors (growths), and to recognize new genes related with certain diseases (Ohlander Citation2014).
Lab-on-a-chip (LOC) device have been used for protein characterization, genetic analysis of proteins, protein–protein, protein–nucleic acids, protein–enzymes, protein–small molecules, and protein–drugs interactions, as well as comparison of proteins in normal state and disease conditions, finally will result in understanding the production of abnormal proteins and chemicals, the proteins to be targeted for treatment of diseases, and protein impaired pathways (Jain Citation2005b). Another important field that LOC technology has been used in which is to discover novel protein (or nucleic acid) biomarkers, which may help in the detection and/or diagnosis of a disease, the indication of the disease stage or the monitoring the course of a disease (Mohammed and Desmulliez Citation2011), such as protein biomarkers or tumor markers for skin, intestinal, or breast cancers, etc. (Muluneh and Issadore Citation2014).
Potential risks
Existing drawbacks to LOC technology involve inability to multiplex, the inability to identify, and quantify low concentration levels (submicromolar regime), and complex fabrication/biofunctionalization protocols that are difficult and costly to substitute. Most significantly, there is still an important inability to carry out most tests in complex media such as serum or blood which means that most samples need undertake cleanup prior to analysis on a chip device and extensive sample purification. Therefore, fundamental design questions regarding signal quantification, sample preparation, and LOC design still must to be addressed before widely commercialization of LOCs. A novel pattern shift in LOC design is requisite to address the exciting challenges related to the nanotechnology. To address these challenges, scientists launch to reveal bioagent detection/quantification schemes and mobile sample preparation that interact with and move within the sample solution and will also greatly reduce the throughput performance and fabrication complexity of LOC devices. This can be overcome using FRET.
Conclusion and remarks
The LOC has abundant promising for addressing environmental requirements and contains the potential to hugely improve the cost, efficiency, and speed of almost all chemical-based nanodiagnostics. To shift this nanotechnology to commercialization and maturation, some challenges most to be overcome including almost non-existent multiplexed capabilities, complex fabrication protocols, and low target analyte sensitivity. Some of the main advantages of using the LOC systems include reduced consumption of the sample and reagents with minimal waste production, provision of fast and accurate results, simplified handling, and low production cost and instrument size. The two main features such as field portability and rapid sample throughput can improve the LOC to important tool in field of nanodiagnosis using nanotechnology. LOC technology has been broadly studied but still remains rarely commercialized and need further researches.
Acknowledgements
The authors thank Department of Medical Nanotechnology, Faculty of Advanced Medical Science of Tabriz University for all supports provided.
Declaration of interest
The authors declare that they have no competing interests. This work is funded by 2015 Biotechnology Research Center, Tabriz University of Medical Sciences Grant.
References
- Algar WR, Susumu K, Delehanty JB, Medintz IL. 2011. Semiconductor quantum dots in bioanalysis: crossing the valley of death. Analyt Chem. 83:8826–8837.
- Balasubramanian S, Kagan D, Jack Hu CM, Campuzano S, Lobo–Castañon MJ, Lim N, et al. 2011. Micromachine-enabled capture and isolation of cancer cells in complex media. Angew Chem Int Ed. 50:4161–4164.
- Benson J, Barrett S. 2005. Next generation autonomous wheelchair control. Biomed Sci Instrum. 41:283–288.
- Bourzac K. 2012. Nanotechnology: carrying drugs. Nature. 491:S58–S60.
- Bullis K. 2006. Brain-healing nanotechnology. Technol Rev. 14:115–131.
- Cabrera L. 2009. Nanotechnology: changing the disability paradigm. Int J Disabil Commun Rehabil. 8(2):1–8.
- Campuzano S, Orozco J, Kagan D, Guix M, Gao W, Sattayasamitsathit S, et al. 2011. Bacterial isolation by lectin-modified microengines. Nano Lett. 12:396–401.
- Castillo-León J, Svendsen WE. 2014. Lab-on-a-Chip Devices and Micro-Total Analysis Systems: A Practical Guide. Switzerland: Springer.
- Chaudhry Q, Blackburn J, Floyd P, George C, Nwaogu T, Boxall A, et al. 2006. Final report: a scoping study to identify gaps in environmental regulation for the products and applications of nanotechnologies. London: Defra.
- Chin CD, Linder V, Sia SK. 2012. Commercialization of microfluidic point-of-care diagnostic devices. Lab on a Chip. 12:2118–2134.
- Claussen JC, Franklin AD, ul Haque A, Porterfield DM, Fisher TS. 2009. Electrochemical biosensor of nanocube-augmented carbon nanotube networks. ACS Nano. 3:37–44.
- Claussen JC, Kumar A, Jaroch DB, Khawaja MH, Hibbard AB, Porterfield DM, et al. 2012. Nanostructuring platinum nanoparticles on multilayered graphene petal nanosheets for electrochemical biosensing. Adv Funct Mater. 22:3399–3405.
- Daraee H, Eatemadi A, Abbasi E, Fekri Aval S, Kouhi M, Akbarzadeh A. 2014a. Application of gold nanoparticles in biomedical and drug delivery. Artif Cells Nanomedicine Biotechnol. [Epub ahead of print]. DOI: https://doi.org/10.3109/21691401.2014.955107.
- Daraee H, Etemadi A, Kouhi M, Alimirzalu S, Akbarzadeh A. 2014b. Application of liposomes in medicine and drug delivery. Artif Cells Nanomedicine Biotechnol. [Epub ahead of print]. DOI:https://doi.org/10.3109/21691401.2014.953633.
- Dixit CK, Aguirre GR. 2014. Protein microarrays with novel microfluidic methods: current advances. Microarrays. 3:180–202.
- Eatemadi A, Daraee H, Zarghami N, Melat Yar H, Akbarzadeh A. 2014a. Nanofiber: synthesis and biomedical applications. Artif Cells Nanomed Biotechnol. [Epub ahead of print]. DOI:https://doi.org/10.3109/21691401.2014.922568.
- Eatemadi A, Daraee H, Karimkhanloo H, Kouhi M, Zarghami N, Akbarzadeh A, et al. 2014b. Carbon nanotubes: properties, synthesis, purification, and medical applications. Nanoscale Res Lett. 9:1–13.
- Eatemadi A, Darabi M, Afraidooni L, Zarghami N, Daraee H, Eskandari L, et al. 2015. Comparison, synthesis and evaluation of anticancer drug-loaded polymeric nanoparticles on breast cancer cell lines. Artif Cells Nanomedicine Biotechnol. [Epub ahead of print]. DOI: https://doi.org/10.3109/21691401.2015.1008510.
- Fernandes AC, Duarte CM, Cardoso FA, Bexiga R, Cardoso S, Freitas PP. 2014. Lab-on-chip cytometry based on magnetoresistive sensors for bacteria detection in milk. Sensors (Basel). 14:15496–15524.
- Geho DH, Jones CD, Petricoin EF, Liotta LA. 2006. Nanoparticles: potential biomarker harvesters. Curr Opin Chem Biol. 10:56–61.
- Gill R, Zayats M, Willner I. 2008. Semiconductor quantum dots for bioanalysis. Angew Chem Int Ed Engl 47:7602–7625.
- Hong JW, Quake SR. 2003. Integrated nanoliter systems. Nat Biotechnol. 21:1179–1183.
- Jain KK. 2005a. Nanotechnology in clinical laboratory diagnostics. Clin Chim Acta. 358:37–54.
- Jain K. 2007. Nanobiotechnology: Applications, Markets and Companies. Basel: Jain (Ed) Pharma Biotech Publications.
- Jain KK. 2003. Nanodiagnostics: application of nanotechnology in molecular diagnostics. Expert Rev Mol Diagn. 3:153–161.
- Jain K. 2005b. Nanotechnology-based lab-on-a-chip devices. In: Fuchs J, Podda M, Eds. Encyclopedia of Diagnostic Genomics and Proteomics. New York: Marcel Dekkar, pp. 891–895.
- Janasek D, Franzke J, Manz A. 2006. Scaling and the design of miniaturized chemical-analysis systems. Nature. 442:374–380.
- Kubik T, Bogunia-Kubik K, Sugisaka M. 2005. Nanotechnology on duty in medical applications. Curr Pharm Biotechnol. 6:17–33.
- Lee J-H, Galli GA, Grossman JC. 2008. Nanoporous Si as an efficient thermoelectric material. Nano Lett. 8:3750–3754.
- Lombardi C. 2006. MIT Group Develops ‘Mind-Reading’ Device. Cambridge (MA): CNET News.
- Loomis JM. 2002. Sensory replacement and sensory substitution: overview and prospects for the future. In: Roco MC, Bainbridge WS, Eds. Converging Technologies for Improving Human Performance. Boston (MA): Kluwer Academic, pp. 189–198.
- Lukyanov AN, Elbayoumi TA, Chakilam AR, Torchilin VP. 2004. Tumor-targeted liposomes: doxorubicin-loaded long-circulating liposomes modified with anti-cancer antibody. J Control Release. 100:135–144.
- Maeda M, Kuroda C, Shimura T, Tada M, Abe M, Yamamuro S, et al. 2006. Magnetic carriers of iron nanoparticles coated with a functional polymer for high throughput bioscreening. J Appl Phys. 99:08H103-08H-3.
- Malsch I. 2002. Biomedical applications of nanotechnology. Ind Physicist. 8:15–17.
- McKendry R, Zhang J, Arntz Y, Strunz T, Hegner M, Lang HP, et al. 2002. Multiple label-free biodetection and quantitative DNA-binding assays on a nanomechanical cantilever array. Proc Natl Acad Sci USA. 99:9783–9788.
- Mellatyar H, Akbarzadeh A, Rahmati M, Ghalhar MG, Etemadi A, Nejati-Koshki K, et al. 2014. Comparison of inhibitory effect of 17-DMAG nanoparticles and free 17-DMAG in HSP90 gene expression in lung cancer. Asian Pac J Cancer Prev. 15:8693–8698.
- Millington DS, Sista R, Bali D, Eckhardt AE, Pamula V. 2014. Development of biomarker assays for clinical diagnostics using a digital microfluidics platform. In: Li W, Lee MS, Eds. Dried Blood Spots: Applications and Techniques. Hoboken (NJ): John Wiley & Sons, Inc., pp. 325–331.
- Mirasoli M, Guardigli M, Michelini E, Roda A. 2014. Recent advancements in chemical luminescence-based lab-on-chip and microfluidic platforms for bioanalysis. J Pharm Biomed Anal. 87:36–52.
- Mohammed M-I, Desmulliez MP. 2011. Lab-on-a-chip based immunosensor principles and technologies for the detection of cardiac biomarkers: a review. Lab on a Chip. 11:569–595.
- Muluneh M, Issadore D. 2014. Microchip-based detection of magnetically labeled cancer biomarkers. Adv Drug Deliv Rev. 66:101–109.
- Ohlander A, Bauer S, Ramachandraiah H, Russom A, Bock K, editors. 2014. Microfluidic Detection Module for DNA Analysis Using Integrated Microheaters and DNA Microarrays on Plastic Foil. Proceedings of the Sensors and Measuring Systems 2014; 17 ITG/GMA Symposium; Nuremberg, Germany: VDE.
- Phurimsak C, Tarn MD, Peyman SA, Greenman J, Pamme N. 2014. On-chip determination of C-reactive protein using magnetic particles in continuous flow. Analyt Chem. 86:10552–10559.
- Prakash R, Pabbaraju K, Wong S, Wong A, Tellier R, Kaler K. 2014. Droplet microfluidic chip based nucleic acid amplification and real-time detection of influenza viruses. J Electrochem Soc. 161:B3083–B3B93.
- Radford T. 2003 Brain implant may restore memory. The Guardian. Available from: http://www.guardian.co.uk/international/story/ 0.3604(912940):00.
- Saini R, Saini S, Sharma S. 2010. Nanotechnology: the future medicine. J Cutan Aesthet Surg. 3:32–33.
- Sanchez S, Solovev AA, Schulze S, Schmidt OG. 2011. Controlled manipulation of multiple cells using catalytic microbots. Chem Commun. 47:698–700.
- Sapsford KE, Granek J, Deschamps JR, Boeneman K, Blanco-Canosa JB, Dawson PE, et al. 2011. Monitoring botulinum neurotoxin a activity with peptide-functionalized quantum dot resonance energy transfer sensors. ACS Nano. 5:2687–2699.
- Seidi K, Eatemadi A, Mansoori B, Jahanban-Esfahlan R, Farajzadeh D. 2014. Nanomagnet-based detoxifying machine: an alternative/complementary approach in HIV therapy. J AIDS Clin Res. 5:2.
- Shanmukh S, Jones L, Driskell J, Zhao Y, Dluhy R, Tripp RA. 2006. Rapid and sensitive detection of respiratory virus molecular signatures using a silver nanorod array SERS substrate. Nano Lett. 6:2630–2636.
- Tachibana R, Harashima H, Shono M, Azumano M, Niwa M, Futaki S, et al. 1998. Intracellular regulation of macromolecules using pH-sensitive liposomes and nuclear localization signal: qualitative and quantitative evaluation of intracellular trafficking. Biochem Biophys Res Commun. 251:538–544.
- Torchilin VP. 2005a. Lipid-core micelles for targeted drug delivery. Curr Drug Deliv. 2:319–327.
- Torchilin VP. 2005b. Recent advances with liposomes as pharmaceutical carriers. Nat Rev Drug Discov. 4:145–160.
- Torchilin VP, Levchenko TS, Rammohan R, Volodina N, Papahadjopoulos-Sternberg B, D'Souza GG. 2003. Cell transfection in vitro and in vivo with nontoxic TAT peptide-liposome–DNA complexes. Proc Natl Acad Sci USA. 100:1972–1977.
- Torchilin VP. 2012. Multifunctional nanocarriers. Adv Drug Deliv Rev. 64:302–315.
- Varghese SS, Zhu Y, Davis TJ, Trowell SC. 2010. FRET for lab-on-a-chip devices – current trends and future prospects. Lab on a Chip. 10:1355–1364.
- Veronese FM, Pasut G. 2005. PEGylation, successful approach to drug delivery. Drug Discov Today. 10:1451–1458.
- Vives E, Brodin P, Lebleu B. 1997. A truncated HIV-1 Tat protein basic domain rapidly translocates through the plasma membrane and accumulates in the cell nucleus. J Biol Chem. 272:16010–16017.
- Wang J, Gao W. 2012. Nano/microscale motors: biomedical opportunities and challenges. Acs Nano. 6:5745–5751.
- Wei Q, Lee M, Yu X, Lee EK, Seong GH, Choo J, et al. 2006. Development of an open sandwich fluoroimmunoassay based on fluorescence resonance energy transfer. Analyt Biochem. 358:31–37.
- Whitesides GM. 2006. The origins and the future of microfluidics. Nature. 442:368–373.
- Zhao X, Hilliard LR, Mechery SJ, Wang Y, Bagwe RP, Jin S, et al. 2004. A rapid bioassay for single bacterial cell quantitation using bioconjugated nanoparticles. Proc Natl Acad Sci USA. 101:15027–15032.
- Zhao M, Kircher MF, Josephson L, Weissleder R. 2002. Differential conjugation of tat peptide to superparamagnetic nanoparticles and its effect on cellular uptake. Bioconj Chem. 13:840–844.