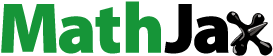
Abstract
Background Curcumin (diferuloylmethane) is a polyphenol derived from the plant Curcuma longa, commonly called turmeric. Extensive research over the last 50 years has demonstrated that these polyphenols play an important role in the maintenance of health and prevention of diseases, in addition to its therapeutic benefits such as anti-tumor, anti-inflammatory, and anti-oxidant activities. Materials and methods This study is devoted to the enhancement of the solubility and bioavailability of curcumin nanoparticles prepared by a process based on a wet-milling technique and then examine in vitro against prostate cancer cell line 3 (PC3), human embryonic kidney cell line (HEK), human erythrocytes (red blood cells (RBCs)), and against fourth different bacterial strains two gram-positive (Micrococcus luteus ATCC 9341, Staphylococcus aureus ATCC 29213), two gram-negative (Escherichia coli ATCC 25922, Pseudomonas aeruginosa ATCC 27853). Results The cell viability curve, the half maximal inhibitory concentration (IC50), and the minimum bactericidal concentration (MBC) were evaluated. Nanocurcumin displayed significant activity against cancer cell line (PC3) and low toxicity against normal cells (HEK) compared with parent curcumin in favor of PC3 (P < 0.05). In addition, it was found that the efficiency of toxicity for nanocurcumin against PC3 (E% = 59.66%) was much better than HEK (E% = 36.07%) compared with parent curcumin. The results also demonstrate that, although nanocurcumin has a little more ability to lays RBCs than parent curcumin after incubated 60 min, but the hemolysis % remained very low and there was no significant difference between hemolysis % of nanocurcumin and parent curcumin (P > 0.05). On the other hand, the results demonstrate that, the MBCs of nanocurcumin were lower than curcumin for all different bacterial strains. Moreover, the selected gram-positive bacteria had higher sensitivity than the selected gram-negative bacteria for both curcumin and nanocurcumin. In conclusion, all these findings not only indicate that nanocurcumin safe compound has a potent ability as anti-cancer and antimicrobial activities, but also well justify the avail of using nanocurcumin as prostate cells PC3 anti-cancer, and antimicrobial agent for nanocurcumin are markedly improved by decreasing particle size to the nano-scale regime.
Introduction
Curcumin [chemical name: (E,E)-1,7-bis(4-hydroxy-3-methoxyphenyl)-1,6-heptadiene-3,5-dione] is the active ingredient in the traditional herbal remedy and dietary spice turmeric. It has a molecular formula C21H20O6, a molecular weight (MW) 368.38, and a melting point of 179–183 °C (Basnet and Skalko-Basnet Citation2011). Curcumin is more soluble in ethanol, dimethyl sulfoxide (DMSO), methanol, and acetone than in water (Sou Citation2012).
Curcumin is brightly yellow-colored compound derived from the popular Indian spice of turmeric, which is a member of the ginger family (Zingiberaceae). Being usually used as a food coloring, curcumin has been used as a food additive, its E number is E100 (Akram et al. Citation2010). Several studies on curcumin as medicinal plant have been reported in literature. It was firstly discovered about 200 years ago by Harvard College laboratory scientists Vogel and Pelletier from the rhizomes of Turmeric (Curcuma longa) (Gupta et al. Citation2013). Curcumin is described as an anti-tumoral, anti-inflammatory, and anti-oxidant agent capable of inducing apoptosis in numerous cellular systems. It has shown also one of the most studied chemopreventive agents (Duvoix et al. Citation2005). Moreover, curcumin has strong therapeutic potential and protective effect against a variety of cancers namely blood, oral cavity, skin, lung, pancreas, intestinal tract, and against human prostate cancer cell line 3 (PC3). Curcumin has also shown ability to suppress proliferation, transformation, and metastasis of tumors (Shishodia et al. Citation2007). Moreover, curcumin has been reported to have ability to decrease the proliferation potential of PC cells (Nakamura et al. Citation2002). Since then, the polyphenol has been found to possess anti-oxidant, anti-inflammatory, hypoglycemic, wound-healing, antimicrobial, and anti-tumor activities (Aggarwal and Sung Citation2009, Akram et al. Citation2010).
So far, most of the cancer treatment regimens focus primarily on chemotherapy as the treatment of choice for advanced or metastatic disease. Recently, treatment by traditional medicinal plants and herbs as alternative medicine has seen a particular interest. In industrialized countries, only few years back, much of what is today considered “alternative medicine” was the only medicine (Bass and Young Citation1996). Indeed, a major part of the world’s population still depends on alternative forms of treatment for most diseases (Miller Citation1998). Most likely, more significant to the progress of pharmacology has been obtained from studies devoted to action mechanisms of naturally occurring chemicals derived from medicinal herbs. The development of new synthetic chemicals that account for a major part of the medicaments used in modern medicine was supported by studies of chemicals derived from plants (Capasso et al. Citation1996). Medicaments such as quinine, morphine, digitalis, and aspirin were all isolated or synthesized from materials derived from plants (Jonas Citation1998).
Extensive preclinical studies for over 30 years ago have indicated curcumin’s therapeutic potential against a wide range of human diseases (Aggarwal and Harikumar Citation2009). Curcumin exerts anti-inflammatory activity by the inhibition of a number of molecules which play an active role in inflammation. In addition, turmeric is used in reducing post-surgical inflammation as well as to prevent atherosclerosis. Curcumin prevents the growth of Helicobacter pylori, which causes gastric ulcers and has been linked with gastric cancers. Curcumin can also lead to reduce the toxicity of heavy metals such as cadmium (Cd) by binding with these heavy metals. This property of curcumin explains its protective action to the brain (Akram et al. Citation2010). Curcumin has been found to exhibit strong anti-oxidant activity, comparable to vitamins E and C, meanwhile demonstrated as a reducing agent and as a radical scavenger (Kunchandy and Rao Citation1989, Rao Citation1993, Tønnesen and Greenhill Citation1992). Curcumin possesses chelating and anti-oxidant properties, which increase the capability of reducing oxidative damage in red blood cells (RBCs) (Tønnesen et al. Citation1994). The clinical trials conducted so far, have indicated the active therapeutic potential of curcumin against many human diseases. It has shown also protection against chronic arsenic exposure, hepatic conditions, and alcohol intoxication (Gupta et al. Citation2013).
Curcumin is a highly pleiotropic molecule, which has shown antibacterial activity in 1949 (Schraufstätter and Bernt Citation1949). So far, several studies research described this natural compound as anti-bacterial agent; and it has ability to inhibit many types of bacteria. Studies devoted to evaluate the synergistic effect of these extracts, the minimum inhibition and bactericidal concentration (MIC and MBC) were carried out, where extraction from Turmeric of (C. longa) was examined against fourth types of bacterial strains. The results showed that the Escherichia coli alone was significantly susceptible to turmeric (Yusra et al. Citation2013). Moreover, study conducted to evaluate the antibacterial activities of curcumin against gram-positive and gram-negative bacteria; revealed that the highest effective was found for Staphylococcus aureus, where the MIC and MBC were 1 mg/ml and 1 mg/ml, respectively (Hamed et al. Citation2013).
On the other hand, curcumin is considered as a safe plant; several studies have shown its non-toxicity toward humans. The safety and non-toxicity of curcumin even at high doses are well established by human clinical trials (Gupta et al. Citation2012, Vogel and Pelletier Citation1815). Several toxicological studies on this compound indicated that curcumin is non-toxic drug even at high concentrations (Ammon and Wahl Citation1991). It has been reported that, at a certain dose of 8 g/d in combination with gemcitabine, was safe and well tolerated in patients with pancreatic cancer (Dhillon et al. Citation2008, Kanai et al. Citation2011). Although curcumin is an excellent safe drug, it has also potential to prevention and/or treatment of cancer and other chronic diseases, however, it could not achieve the optimum therapeutic outcome in the previous clinical studies, generally due to its low solubility and poor bioavailability (Basnet and Skalko-Basnet Citation2011).
So far, several studies have demonstrated that curcumin is very active as anti-tumor and antimicrobial agent, but being water soluble and due its poor bioavailability, it represents some disadvantages for its use. This has encouraged some researchers to use curcumin as nanoparticles (Basniwal et al. Citation2011) in order to enhance its solubility and bioavailability.
The aim of this study consists of the examination of curcumin nanoparticle with sizes in the range of 34–359.4 nm (prepared by a wet-milling technique). Therefore, this study is devoted particularly to enhance the absorption, cellular uptake, bioavailability, and ultimate efficiency of curcumin (enhance curcumin activity as an anti-cancer and antimicrobial combined with fixing its mild cytotoxic profile against mammalian cell line human embryonic kidney cell line (HEK) and human erythrocytes). The synthesis of curcumin nanoparticles was followed by subsequent examination of the parent curcumin then compare with modified curcumin (nanocurcumin) against PC3, mammalian cell line HEK, human erythrocytes, and fourth different bacterial strains.
Materials and methods
Preparation of curcumin nanoparticles
Curcumin, (E,E)-1,7-bis(4-hydroxy-3-methoxyphenyl)-1,6-heptadiene-3,5-dione, was purchased from Sigma Aldrich® (St. Louis, MO) (100 mg, 0.27 mmol) was added to (20 ml) dichloromethane Sigma-Aldrich (St. Louis, MO), then 1 ml from this solution was sprayed into 50 ml boiling water as dropwise using a flow rate of 0.2 ml/min under ultrasonic conditions. After completing 1 ml dropwise, the content was subjected to sonication for 10 min then stirred (200, 400, 600, 800) rpm for 20 min at room temperature to get clear orange colored product which was finally freeze-dried to obtain an orange powder (Basniwal et al. Citation2011).
Cell culture
The following cell lines (HEK 293 Human Embryonic Kidney 293 cell line) and PC3 purchased from ATCC were routinely cultured in RPMI 1640 or Dulbecco’s modified Eagle’s medium containing 10% fetal bovine serum, 1% l-glutamine, 1% sodium pyruvate, 50 U/ml penicillin, and 50 mg/ml streptomycin. The cells were seeded into 150 cm2 culture flasks. Cells were cultured as monolayers in a humidified environment of 5% CO2 95% air at 37 °C.
Cell proliferation assay
The cytotoxicity of nanocurcumin and parent curcumin against cultured cells was determined by the MTT assay. Both of PC3 and HEK cell line were seeded at a density of 5 × 103 cells per well into a 96-well microtitre plate containing 200 μL serum free media. Cells were incubated with six different concentrations of compounds. After incubated 24 h, 20 μL of 5 mg/ml of 3-(4,5-dimethylthiazol-2-yl)-2,5-diphenyltetrazolium bromide (MTT) was added and incubated for 4 h. Conversion of MTT into purple formazan by metabolically active cells indicates the extent of cell viability. The medium was replaced by 200 μL of DMSO and mixed to dissolve the formazan crystals that have been developed. Absorbance was measured using an ELISA Microplate Reader at 550 nm. The data were processed using Kaleidagraph software version 4.02 and presented as the mean ± standard error of the mean.
Erythrocyte hemolysis assay
The determination of the hemolytic activity of nanocurcumin and parents curcumin was performed as reported previously (Almaaytah et al. Citation2013). The nanocurcumin and parent curcumin solutions with different concentrations (50, 100, 200, 400, and 600 μM) were prepared with phosphate-buffered saline. Each drug solution was prepared individually from a single stock solution, where for curcumin solution, 1% DMSO was added to solution; and for nanocurcumin solution, 0% DMSO under sonication conditions for about 1 h. Briefly, a 4% suspension of human erythrocytes was incubated with the peptide for 60 min. Centrifugation with 0.9% NaCl was used for washing the human erythrocytes several times followed by incubation for 60 min at 37 °C. The cells were then centrifuged at 900 × g for 5 min until the supernatant was separated from the pellet. 200 μL were transferred from each sample supernatant as 4 replicates into a 96-well plate and their absorbance measured at 570 nm. 0.9% NaCl was used as the negative control, 0.1% Triton X-100 as the positive control. The percent hemolysis was calculated using the following equation: hemolysis = (A − A°− A0)/(AX − A0) × 100, where A is OD 570 nm with the drug solution, A° is OD 570 nm for drug alone without cells (control drug solution). A0 is OD 570 nm in NaCl, and AX is OD 570 nm with 0.1% Triton X-100.
Bacterial strains
The bacterial strains used for the determination and testing of the antimicrobial activity of nanocurcumin and curcumin, by evaluating the MBC against four bacterial strains including two gram positive (Micrococcus luteus ATCC 9341, Staphylococcus aureus ATCC 29213) and two gram negative (Escherichia coli ATCC 25922, Pseudomonas aeruginosa ATCC 27853). The different bacterial strains were inoculated into nutrient broth media and on nutrient agar media, the resulting pure colonies were used to prepare bacterial suspensions. A loop full of the tested bacteria was used to inoculate nutrient broth and was incubated for 24 h at 37 °C. The size was adjusted to 0.5 McFarland standard turbidity. These strains were provided by the Laboratory of Microbiology and Biotechnology, Department of Biological Sciences, Yarmouk University (Jordan).
Minimum bactericidal concentration (MBC)
Susceptibility testing was performed by adopting the micro-broth dilution method outlined by the Clinical and Laboratory Standards Institute guidelines using sterile 96-well plates (Almaaytah et al. Citation2014, Komatsuzawa et al. Citation2000). The tested bacterial strains were cultured into 10 ml of nutrient broth tube and incubated for 24 h at 37 °C. After incubation, 10 μl of each tube was added into separated well of sterile 96-well micro-plate. Different volume of drug (curcumin and nanocurcumin) was added into different well, also nutrient broth was added into well up to 200 μl final volume to get a concentration range from 500 to 1000 μg/ml. were prepared with distilled water, each drug solution was prepared individually from a single stock solution, for curcumin solution 25% DMSO was added to solution, and for nanocurcumin solution 0% DMSO under sonication conditions for about 1 h. The control wells for parent curcumin and nanocurcumin contain 10 μl from bacterial strain and 190 μl nutrient broth to reach a final volume of 200 μl and each test was carried out in triplicate. Then 10 μl of each well was inoculated onto nutrient agar plate and incubated at 37 °C for 24 h (Almaaytah et al. 2014). The bacterial colonies were enumerated and the average number of colony counts for each group was determined.
The inhibition was calculated using the following formula:
where T is colony forming unit of the test sample (cfu/ml) and C is cfu/ml of the control (Basniwal et al. Citation2011). The MBC value was defined as the lowest concentration of antimicrobial agent that can kill > 99% of the microorganism population (Basri et al. Citation2012).
Statistical analysis
The data were processed using Kaleidagraph software version 4.02 and presented as the mean ± standard error of the mean. Statistical analysis was performed using SPSS version 21. Data were shown as the mean values calculated from all experiments. All comparisons between the experimental groups were performed by AKNOVA test. P values less than 5% were considered to be statistically significant.
Results
Characterization of curcumin nanoparticles
Environmental Scanning Electron Microscope (ESEM) – Quanta 450 FEG-USA/EEU – Just at Nano Center in Jordan University of Science and Technology was used for morphological observations of the as-prepared powders. First, small amount of the powder was spread over a carbon tab then dried by nitrogen stream. Even though the particles were aggregated, ESEM image revealed the existence of nanoparticles, but it was difficult to determine the size, because of high tendency of agglomeration. To overcome that, the powder was coated by platinum (Pt). The new ESEM image () showed clearly the formation of nanoparticles with an average size in the range of 34.0–359.4 nm.
Viability of eukaryotic cells in culture
Yellow MTT is reduced by the mitochondria of living cells into purple formazan and the crystals that develop can be dissolved in DMSO with subsequent spectrophotometric measurement of the color. The changes in this color can be used to measure the extent of cell death and viability. The anti-proliferative activity for both curcumin and nanocurcumin was measured using cell proliferation assay, which was performed against one type of cancer cell line (PC3) and one type of mammalian cells (HEK). Half maximal inhibitory concentration (IC50) values for both nanocurcumin and parent curcumin were summarized in .
Table 1. IC50 values for the nanocurcumin and parent curcumin.
The comparative toxicity between curcumin and nanocurcumin against PC3 cells was investigated at six different concentrations (50, 100, 150, 300, 450, and 600 μM) as shown in . This figure illustrated the viability of PC3 after treatment with nanocurcumin and parent curcumin and incubated overnight at 37 °C with 5% CO2 incubation. There is a significant difference between nanocurcumin and parent curcumin groups at all concentrations (P < 0.05). However, from the figure, we can notice that the differences between two bars of both nanocurcumin and parent curcumin were clearer at concentrations of (150, 300, and 450 μM) than other concentrations. On the other hand, it can be noticed that the viability for nanocurcumin is less than that for curcumin; the IC50 for curcumin was 302.24 μM compared with 121.92 μM for nanocurcumin with an efficiency % (E%) of 59.66% (nanocurcumin efficacy compared with parent curcumin to reduce the cell viability). This means that nanocurcumin has higher toxicity than parent curcumin on PC3 cells, which can be explained by the fact that the nanocurcumin is more soluble in addition to higher cellular uptake; the increment of cellular uptake leads nanocurcumin to be more saturate than parent curcumin in the cells after overnight incubation.
Figure 2. The cell viability for PC3 prostate cancer cell line after treatment by curcumin and nanocurcumin, after incubated overnight at 37 °C and 5% CO2. The Y axis indicates the cell viability at λ = 540 nm and the X axis corresponding to curcumin and nanocurcumin concentration. Data represent three independent experiments.
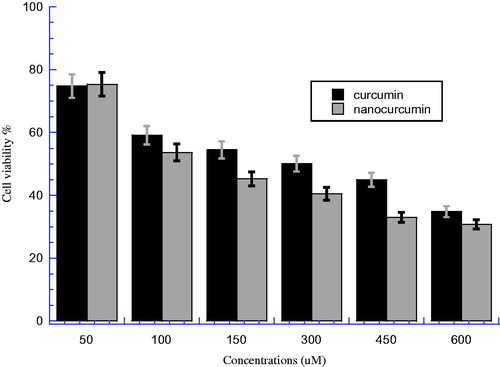
The comparative effect between nanocurcumin and parent curcumin against HEK normal cells was investigated at similar concentrations as shown in . There is a significant difference between nanocurcumin and parent curcumin groups at all concentrations (P < 0.05). However, from the figure, we can see that the differences can be considered negligible (less significant) within the range of error bars for both drugs at all concentrations. The IC50 for curcumin was 458.14 μM which is higher than 292.88 μM for nanocurcumin, and the E% for IC50 between two samples was 36.07%. This means that nanocurcumin has more toxicity on HEK cells than curcumin, which means that nanocurcumin is more soluble with higher cellular uptake; the increment of cellular uptake leads nanocurcumin to be more saturate than parent curcumin in the cells after overnight incubation.
Figure 3. The cell viability for HEK cell line after treatment by curcumin and nanocurcumin, after incubated overnight at 37 °C and 5% CO2. The Y axis indicates the cell viability at λ = 540 nm and the X axis corresponding to curcumin and nanocurcumin concentration. Data represent three independent experiments.
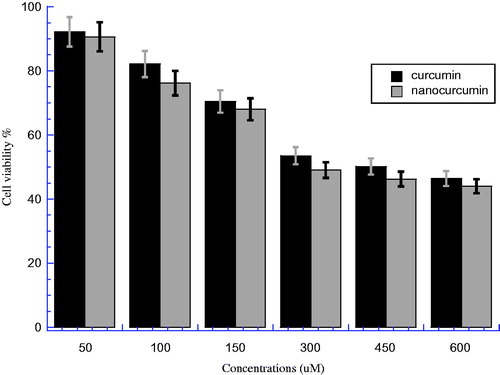
The comparative viability between PC3 cancer cells and HEK normal cells after being treated by nanocurcumin and parent curcumin was investigated at similar concentrations as shown in . clearly shows lower viability for PC3 than HEK at all concentrations, which means that curcumin has more toxicity on PC3 than HEK cells; IC50 for PC3 is 302.24 μM which is lower than 458.14 μM for HEK with E% of ∼34.03%. The statistical analysis of variance for both PC3 and HEK indicates a significant difference in favor of PC3 (where P < 0.05). On the other hand, also shows a considerable difference between viability of PC3 and HEK; where the viability of PC3 is lower than that of HEK at all concentrations, which means nanocurcumin has more toxicity on PC3 than HEK. The IC50 for PC3 is much lower than for HEK; 121.92 and 292.88 μM, respectively, with E% ∼58.37%. The statistical analysis of variance of both PC3 and HEK revealed that there is a significant difference in favor of PC3 (where P < 0.05). The nanocurcumin (water) has helped to keep the IC50 for PC3 lower than for HEK with a larger efficiency in comparison with parent curcumin (DMSO).
Figure 4. (A) The cell viability for HEK and PC3 cells line after treatment by curcumin, after incubated overnight at 37 °C and 5% CO2 (P < 0.05). (B) The cell viability for HEK and PC3 cells line after treatment by nanocurcumin, after incubated overnight at 37 °C and 5% CO2 (P < 0.05). The Y axis indicates the cell viability at λ = 540 nm and the X axis corresponding to curcumin and nanocurcumin concentration. Data represent three independent experiments.
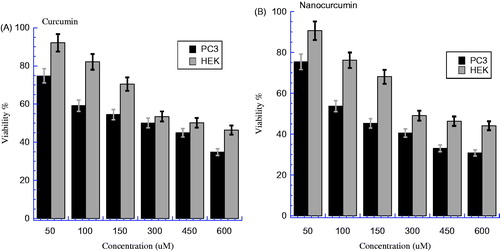
Hemolytic activity of nanocurcumin against human erythrocytes
Hemolysis is defined as the breakage of the RBC membrane, causing the release of the hemoglobin and other internal components into the surrounding fluid. Hemolysis can be visually detected by showing a pink to red tinge in serum or plasma. Hemolysis is a common occurrence seen in serum samples and may compromise the laboratory’s test parameters. The nanocurcumin solution was prepared with distilled water and 0% DMSO under sonication conditions for 1 h, while the parent curcumin solution was prepared with distilled water and 1% DMSO was added to solution.
The hemolytic activity and potential toxicity of nanocurcumin and parent curcumin against human erythrocytes was examined at five different concentrations (50, 100, 200, 400, and 600 μM) after 60 min at 37 °C incubation, as shown in . The results of the hemolytic activity of nanocurcumin and parents curcumin are presented in . The hemolysis % for both nanocurcumin and parents curcumin increases with increasing concentration in a dose-dependent manner. the hemolysis % of both nanocurcumin and parent curcumin did not reach high value up to the highest concentration (); the parent curcumin demonstrates low hemolysis % at all concentrations even up to high concentration (600 μM); i.e., 18.56 ± 2.09% after 60-min incubation (). Likewise, nanocurcumin demonstrates very low hemolysis % up to high concentration; i.e., 19.46 ± 1.26% at 600 μM. This means that both parent curcumin and nanocurcumin have no significant toxicity on RBCs; even up to the high concentration the hemolysis % did not reach 50% hemolysis. Moreover, when nanocurcumin was evaluated against human erythrocytes at a concentration close to their determined respective IC50 for the PC3 cancer cell line (121.92 μM), the nanocurcumin caused a less hemolysis of ∼4.19%. Similarly, when the curcumin was evaluated against human erythrocytes at a concentration close to their determined respective IC50 for the PC3 cancer cell line (302.24 μM), the curcumin caused a hemolysis of ∼11.97% (). This confirms the poor toxicity of both nanocurcumin and parent curcumin against human erythrocytes compared with PC3. Moreover, the results obtained in this study also indicate that there is no significant difference between nanocurcumin and parent curcumin groups, where P > 0.05 (). Additionally, the hemolysis E% between nanocurcumin and curcumin is very low at all deferent concentrations; i.e., the highest E% is 14.6% at concentration 50 μM (). This means that there is no much superior toxicity between nanocurcumin and parent curcumin against human erythrocytes.
Figure 5. Nanocurcumin versus curcumin hemolysis %. Different concentrations of solutions (50, 100, 200, 400, and 600 μM) were incubated with 4% human erythrocytes suspension. Results were recorded at λ = 450 nm after 60 min incubation, P > 0.05 between two groups.
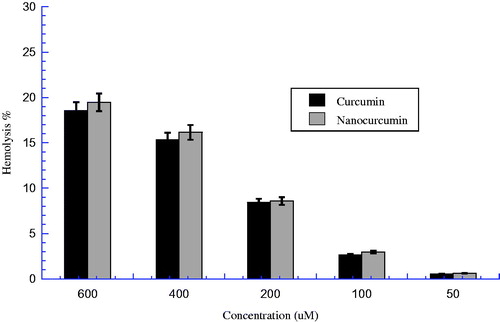
Table 2. The hemolytic effect for both curcumin and nanocurcumin and the efficiency % on human erythrocytes after incubated 60 min.
Antimicrobial activity of nanocurcumin
The bacterial strains used for the determination and examination the antimicrobial activity of nanocurcumin and parent curcumin, by evaluating the MBC against four bacterial strains, including two gram positive (Micrococcus luteus ATCC 9341, S taphylococcus aureus ATCC 29213) and two gram negative (Escherichia coli ATCC 25922, Pseudomonas aeruginosa ATCC 27853). The nanocurcumin solution was prepared with distilled water and 0% DMSO under sonication conditions for 1 h. While the parent curcumin solution was prepared with distilled water and 25% DMSO was added to solution. The results were obtained after treating fourth different bacterial strains over 24 h (100, 200, 300, 400, 500, 600, 700, 800, 900 and 1000 μg/ml) in three independent trials. The MBCs value was defined as the lowest concentration of antimicrobial agent that can kill >99% of the microorganism population and determined using Kaleidagraph, the MBCs were summarized in . shows that, the inhibition % for all tested bacterial strains increased with increasing concentration in a dose-dependent manner. On the other hand, the figure clearly shows that, nanocurcumin has more ability to inhibit all tested bacterial strains compared with parent curcumin. Moreover, the S. aureus has a highest inhibition % (curve) for both nanocurcumin and parent curcumin comparing with other tested bacterial strains. This means that, nanocurcumin displayed potent antimicrobial activities against all bacterial strains studied with E. coli, M. luteus, P. aeruginosa, and S. aureus in comparison with parent curcumin. That’s in turn, due to nanocurcumin (water) more solubility than parent curcumin (DMSO). Moreover, selective gram-positive displayed more sensitivity than selective gram-negative for both nanocurcumin and parent curcumin. The highest bacterial sensitivity was found for S. aureus (MBC = 608.5 μg/ml) when treated by nanocurcumin compared with (MBC = 764.14 μg/ml), while the lowest sensitivity was for E. coli (MBC = 901.93 μg/ml) when treated by nanocurcumin compared with (MBC = 988.78 μg/ml), as shown in .
Figure 6. The bacterial growth curve for Escherichia coli, Micrococcus luteus, Pseudomonas aeruginosa, and Staphyloccus aureus after treatment by both nanocurcumin and parent curcumin at different concentrations then incubated overnight at 37 °C. The Y axis represents the inhibition % and the X axis corresponding to compounds concentrations. The results were repeated in triplicates with standard deviation range (0–1.85) for nanocurcumin and (0.14–1.63) for parent curcumin.
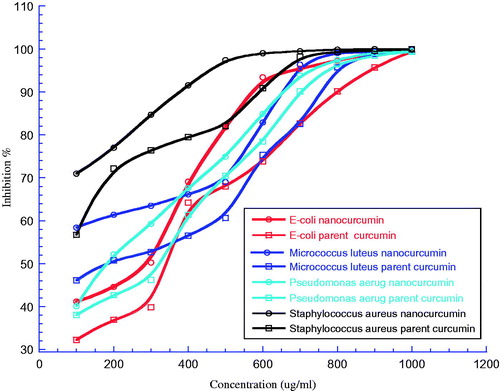
Table 3. Minimum inhibitory concentrations (MBCs) of both nanocurcumin and parent curcumin against the test microorganisms.
Discussion
Curcumin has displayed to possess anti-inflammatory, hypoglycemic, wound-healing, antimicrobial activities, anti-tumoral, and anti-oxidant agent capable of inducing apoptosis in numerous cellular systems (Aggarwal and Sung Citation2009, Akram et al. Citation2010). Moreover, curcumin has strong therapeutic potential and protective effect against a variety type of cancers as well as ability to suppress proliferation, transformation, and metastasis of tumors (Nakamura et al. Citation2002, Shishodia et al. Citation2007). It has been reported as one of the most studied chemopreventive agents (Duvoix et al. Citation2005). Curcumin could not make a clinical impact due to its rapid degradation and poor bioavailability in biological systems (Sharma et al. Citation2007). Some research works on nanomaterials have shown that nano-loaded curcumin has better bioavailability (Muqbil et al. Citation2011). On the other hand, numerous investigations have been conducted to develop more efficient systems for drug delivery. It has been found that the particle size is an important parameter as it can directly affect the physical stability, cellular uptake, and drug release from the nanoparticles (Feng Citation2004). Nanocurcumin demonstrates comparable in vitro therapeutic efficacy to parent’s curcumin against a panel of human pancreatic cancer cell lines, as assessed by cell viability which was tested by MTT assay (Bisht et al. Citation2007). Moreover, a study conducted by Yallapu et al. (Citation2012) suggested that the cellulose-curcumin formulation possesses characteristics that will improve the therapeutic treatment of PC. Curcumin-loaded PLGA nanoparticles showed better intracellular uptake in DU145, PC3, and LNCaP cell lines than parent’s curcumin (Mukerjee and Vishwanatha Citation2009). The authors reported also that MTT assay confirmed that curcumin-loaded PLGA nanoparticles exhibited a lower IC50 value in comparison to curcumin in all studied cancer cell lines, and similar uptake characteristics of curcumin nanocarriers prepared from methoxy polyethylene glycol palmitate in HeLa cell lines (Feng Citation2004). Another research work (Yallapu et al. Citation2011) also presented a study on five different curcumin nanoformulations, and reported that RBCs treated with PLGA, â-cyclodextrin, cellulose, and nanogel curcumin nanoformulations showed no signs of hemolysis at any tested concentration. Also, the dendrimer curcumin nanoformulations did not show signs of hemolysis until 100 μM, but after that, extreme dose-dependent hemolytic activity was observed. Curcumin has shown a strict capability to inhibit bacterial growth. Furthermore, nanocurcumin demonstrated inhibition being more pronounced in gram-positive bacterial strains than gram-negative bacterial strains (Basniwal et al. Citation2011).
Therefore, by taking into consideration all the above results previously reported in the literature, and in order to enhance the cellular uptake, bioavailability, and ultimate efficiency of curcumin, our current research work was mainly focusing on the fabrication of nanocurcumin. The latter was prepared by a process based on a wet-milling technique (Basniwal et al. Citation2011) and ESEM analysis reveals the formation of nanoparticles with a broad particle size distribution in the range of 34.0–359.4 nm (. In this research work, nanocurcumin was used for the development of a new compound with enhanced anti-tumor and antimicrobial activities and decreased toxicity against mammalian cells. Nanocurcumin was modified by reducing the parent curcumin particles size to nano-scale, which in turn, leads to an increase in the cellular uptake, bioavailability, and ultimate efficiency.
The results compared with curcumin (DMSO) show that the toxicity depends on IC50. The IC50 for nanocurcumin (water) was 121.92 and 292.88 μM for PC3 and HEK respectively, compared with curcumin (DMSO) 302.24 and 458.14 μM for PC3 and HEK respectively (), giving an E% for nanocurcumin to curcumin at IC50 59.66 and 36.07% for both PC3 and HEK, respectively. The values of IC50 and E% indicate that nanocurcumin has more uptake than curcumin in both PC3 and HEK, and that nanocurcumin demonstrated more toxicity against PC3. Our results also demonstrate that the viability for nanocurcumin was lower than curcumin at most concentrations on both PC3 and HEK cells ( and ). Moreover, summarized that, the E% for nanocurcumin was (58.37%), which is quite higher than curcumin (34.03%), and this can be considered as an important result. nanocurcumin (water) without DMSO will eliminate the use of toxic chemical substance DMSO as curcumin’s solvent, which in turn, leads to symptoms of harmful side effects by enhancing the solubility and bioavailability by means of preparing curcumin nanoparticles. Moreover, it is important to mention that our results are in general, in good agreement with some previous studies reported in the literature. Liu (Citation2013) reported that the anti-cancer activity of the formulated nanocurcumin was significantly higher than that of pure ones (P = < 0.001). This study suggested that the formulated nanocurcumin was more effective to kill cancer cells; in fact, nanocurcumin, unlike curcumin, favors much more cellular uptake, and readily dispersed in aqueous media (Feng Citation2004). This phenomenon is amplified by using curcumin-loaded nanoparticles, leading to increased preferential cytotoxicity of cancer cells (Mukerjee and Vishwanatha Citation2009). In addition, by the fact that curcumin preferentially induces apoptosis in highly proliferating cells, the death will be more pronounced in cancer cells than in normal cells (Sa and Das Citation2008), which in turn, lead to nanocurcumin being more toxic on cancer cells PC3 than normal cells HEK. Therefore, nanocurcumin might be a better therapeutic approach than curcumin for treating PC3. On the other hand, in order to confirm negligible cytotoxicity of nanocurcumin against human erythrocytes, the results of the examined compounds nanocurcumin and parent curcumin against human erythrocytes displayed that, the hemolysis % of both nanocurcumin and parent curcumin did not reach high value up to the highest concentration (); the parent curcumin demonstrates low hemolysis % at all concentrations even up to high concentration (600 μM); i.e., 18.56 ± 2.09% after 60-min incubation (). Likewise, nanocurcumin demonstrate very low hemolysis % up to high concentration; i.e., 19.46 ± 1.26% at 600 μM. This means that both parent curcumin and nanocurcumin have no significant toxicity on RBCs; even up to the highest concentration and the hemolysis % did not reach 50% hemolysis. On the other hand, it is important to mention that, when nanocurcumin was evaluated against human erythrocytes at a concentration close to their determined respective IC50 for PC3 cancer cell line (121.92 μM), the nanocurcumin caused a less hemolysis of ∼4.19%. In contrary, when the curcumin was evaluated against human erythrocytes at a concentration close to their determined respective IC50 for the PC3 cancer cell line (302.24 μM), the curcumin caused a much higher hemolysis of ∼11.97% (), which is almost three times higher than nanocurcumin. The obtained results are in good agreement with some above previous studies (Aggarwal et al. Citation2003, Banerjee et al. Citation2008, Niki et al. Citation1988, Yallapu et al. Citation2011).
Finally, the resultant changes in the particles size of nanocurcumin lead to a significant enhancement in the antimicrobial activity. The inhibition % for all different bacterial strains was found to increase with increasing both nanocurcumin or parent curcumin concentration with dose-dependent manner (). The MBCs of nanocurcumin were lower than curcumin at all different bacterial strains, where the MBCs of nanocurcumin for Micrococcus luteus ATCC 9341, Staphylococcus aureus ATCC 29213, Escherichia coli ATCC 25922, and Pseudomonas aeruginosa ATCC 27853 were 811.72, 608.5, 901.93, and 894.89 μg/ml, respectively, compared to 903.44, 764.14, 989.65, and 959.88 μg/ml for curcumin, with E% was 10.15, 20.37, 8.86, and 6.77% respectively, as shown in . That’s in turn, due to nanocurcumin (water) having more solubility than parent curcumin (DMSO). On the other hand, the inhibition % for nanocurcumin was higher than curcumin for all tested bacterial strains (). Nanocurcumin displayed potent antimicrobial activities against all selected bacterial strains compared with parent curcumin. Moreover, selective gram-positive displayed more sensitivity than selective gram-negative, which is due to differences between their cell membrane constituents and structure. It is known that gram-positive phospholipid membrane bacteria contain an outer peptidoglycan layer, while gram-negative bacteria contain an outer, and this would lead to different types of interaction when encountered by curcumin (Basniwal et al. Citation2011). Additionally, the highest bacterial sensitivity was for S. aureus (MBC = 608.5 μg/ml) when treated by nanocurcumin compared with (MBC = 764.14 μg/ml). While the lowest sensitivity was found for E. coli (MBC = 901.93 μg/ml) when treated by nanocurcumin compared with MBC = 988.78 μg/ml (see ). By taking into account both MBCs and inhibition %, It can be noticed that the toxicity of nanocurcumin is higher than curcumin, on all different bacterial strains and this can be associated with size reduction; i.e., 34 nm for nanocurcumin particles dissolved in water, which is tremendously smaller than 500–800 nm for curcumin particles dissolved in DMSO. This can be considered as the main responsible factor for better penetration and higher uptake by the cells, leading to more damage in the cell membrane of bacteria (Basniwal et al. Citation2011). The results obtained in this study are in agreement with some earlier reports on nanoparticles of different chemistries found to mobilize inside the cell (Jain et al. Citation2009). Elsewhere Basniwal et al. (Citation2011) developed a method for the preparation of nanocurcumin by a process based on a wet-milling technique and then examined its antimicrobial properties by zone inhibition and MIC. It was found that the curcumin nanoparticles are believed to manifest antibacterial properties by anchoring to the cell wall of the bacterial cell, breaking it, then penetrating inside the cell, and disrupting the structure of cell organelles.
Most of the previous results reported in the literature, indicate that the resultant changes in particles size lead to significant enhancement in the anti-tumor and antimicrobial activities of nanocurcumin toward PC3 cancer cells and microbial cells, while remaining safe against eukaryotic cells (HEK and RBCs). In addition, the increase in anti-tumor and antimicrobial activities was also accompanied by a stable percentage activity of nanocurcumin against HEK and RBCs. As reported earlier by using MTT cytotoxicity assay, nanocurcumin particles caused much lower IC50 of PC3 compared with IC50 of HEK which means that the cytotoxicity of nanocurcumin against human HEK cell line is much lower than PC3. On the other hand, nanocurcumin caused negligible hemolysis % at concentration close to IC50 of PC3 when compared with the parent curcumin which was found to be almost having similar hemolytic and displayed weaker selectivity toward PC3 cancer cells and microbial cells. Finally, and in order to confirm its ability to penetrate the cell membrane, nanocurcumin managed to inhibit the bacterial growth much better compared with parent curcumin on all selected bacterial strains. Finally, the nanocurcumin was able to cause complete inhibition of the bacterial growth on lower concentrations compared with parent curcumin.
Conclusion
In summary, we report the fabrication of nanocurcumin and functional characterization of anti-cancer, antimicrobial, and cytotoxic activities. The obtained results showed enhanced potent anti-cancer and antibacterial activities as well as low hemolytic and cytotoxic activities against eukaryotic cells. The potency of nanocurcumin combined with its mild cytotoxic profile indicate that nanocurcumin has a great potential as an effective anti-tumor (PC3 antiproliferative) and antimicrobial agent, thereby worthy of being subjected to further investigation in order to optimize its pharmacodynamic and pharmacokinetic profiles.
Declaration of interest
The authors report no declarations of interest. The authors alone are responsible for the content and the writing of the paper.
Acknowledgements
The authors would like to acknowledge the support from staff at the Laboratory of Biomedical Physics, Department of Physics, Jordan University of Science and Technology (Jordan), Dr. Ammar Almaaytah, and his Microbiology Laboratory team Mohamad Adel Al-Fandi and Ahmad K. Abu Alhaigaa at Jordan University of Science and Technology (Jordan).
References
- Aggarwal BB, Harikumar KB. 2009. Potential therapeutic effects of curcumin, the anti-inflammatory agent, against neurodegenerative, cardiovascular, pulmonary, metabolic, autoimmune and neoplastic diseases. Int J Biochem Cell Biol. 41:40–59.
- Aggarwal BB, Kumar A, Bharti AC. 2003. Anticancer potential of curcumin: preclinical and clinical studies. Anticancer Res. 23:363–398.
- Aggarwal BB, Sung B. 2009. Pharmacological basis for the role of curcumin in chronic diseases: an age-old spice with modern targets. Trends Pharmacol Sci. 30:85–94.
- Akram M, Shahab-Uddin AA, Usmanghani K, Hannan A, Mohiuddin E, Asif M. 2010. Curcuma longa and curcumin: a review article. Rom J Biol-Plant Biol. 55:65–70.
- Almaaytah A, Tarazi S, Abu-Alhaijaa A, Altall Y, Alshar’i N, Bodoor K, Al-Balas Q. 2014. Enhanced antimicrobial activity of AamAP1-lysine, a novel synthetic peptide analog derived from the scorpion venom peptide AamAP1. Pharmaceuticals. 7:502–516.
- Almaaytah A, Tarazi S, Mhaidat N, Al-Balas Q, Mukattash TL. 2013. Mauriporin, a novel cationic α-helical peptide with selective cytotoxic activity against prostate cancer cell lines from the venom of the scorpion Androctonus mauritanicus. Int J Pept Res Ther. 19:281–293.
- Ammon H, Wahl MA. 1991. Pharmacology of Curcuma longa. Planta Med. 57:1–7.
- Banerjee A, Kunwar A, Mishra B, Priyadarsini K. 2008. Concentration dependent antioxidant/pro-oxidant activity of curcumin: studies from AAPH induced hemolysis of RBCs. Chem Biol Interac. 174:134–139.
- Basnet P, Skalko-Basnet N. 2011. Curcumin: an anti-inflammatory molecule from a curry spice on the path to cancer treatment. Molecules. 16:4567–4598.
- Basniwal RK, Buttar HS, Jain V, Jain N. 2011. Curcumin nanoparticles: preparation, characterization, and antimicrobial study. J Agric Food Chem. 59:2056–2061.
- Basri DF, Luoi CK, Azmi AM, Latip J. 2012. Evaluation of the combined effects of stilbenoid from Shorea gibbosa and vancomycin against methicillin-resistant Staphylococcus aureus (MRSA). Pharmaceuticals. 5:1032–1043.
- Bass S, Young AL. 1996. Dietary Supplement Health and Education Act. A Legislative History and Analysis. Washington (DC): The Food and Drug Law Institute.
- Bisht S, Feldmann G, Soni S, Ravi R, Karikar C, Maitra A, Maitra A. 2007. Polymeric nanoparticle-encapsulated curcumin (“nanocurcumin”): a novel strategy for human cancer therapy. J Nanobiotechnol. 5:1–18.
- Capasso A, de Feo V, de Simone F, Sorrentino L. 1996. Pharmacological effects of aqueous extract from Valeriana adscendens. Phytother Res. 10:309–312.
- Dhillon N, Aggarwal BB, Newman RA, Wolff RA, Kunnumakkara AB, Abbruzzese JL, et al. 2008. Phase II trial of curcumin in patients with advanced pancreatic cancer. Clin Cancer Res. 14:4491–4499.
- Duvoix A, Blasius R, Delhalle S, Schnekenburger M, Morceau F, Henry E, Dicato M, Diederich M. 2005. Chemopreventive and therapeutic effects of curcumin. Cancer Lett. 223:181–190.
- Feng SS. 2004. Nanoparticles of biodegradable polymers for new-concept chemotherapy. Expert Rev Med Devices. 1:115–125.
- Gupta SC, Patchva S, Aggarwal BB. 2013. Therapeutic roles of curcumin: lessons learned from clinical trials. AAPS J. 15:195–218.
- Gupta SC, Patchva S, Koh W, Aggarwal BB. 2012. Discovery of curcumin, a component of golden spice, and its miraculous biological activities. Clin Exp Pharmacol Physiol. 39:283–299.
- Hamed OA, Mehdawi N, Taha AA, Hamed EM, Al-Nuri MA, Hussein AS. 2013. Synthesis and antibacterial activity of novel curcumin derivatives containing heterocyclic moiety. Iran J Pharm Res. 12:47–56.
- Jain J, Arora S, Rajwade JM, Omray P, Khandelwal S, Paknikar KM. 2009. Silver nanoparticles in therapeutics: development of an antimicrobial gel formulation for topical use. Mol Pharm. 6:1388–1401.
- Jonas WB. 1998. Alternative medicine—learning from the past, examining the present, advancing to the future. JAMA. 280:1616–1618.
- Kanai M, Yoshimura K, Asada M, Imaizumi A, Suzuki C, Matsumoto S, et al. 2011. A phase I/II study of gemcitabine-based chemotherapy plus curcumin for patients with gemcitabine-resistant pancreatic cancer. Cancer Chemother Pharmacol. 68:157–164.
- Komatsuzawa H, Ohta K, Sugai M, Fujiwara T, Glanzmann P, Berger-Bächi B, Suginaka H. 2000. Tn551-mediated insertional inactivation of the fmtB gene encoding a cell wall-associated protein abolishes methicillin resistance in Staphylococcus aureus. J Antimicrob Chemother. 45:421–431.
- Kunchandy E, Rao M. 1989. Effect of curcumin on hydroxyl radical generation through Fenton reaction. Int J Pharm. 57:173–176.
- Liu D. 2013. Engineering Nano-curcumin with Enhanced Solubility and In-vitro Anti-cancer Bioactivity. New Brunswick, NJ: Rutgers University–Graduate School.
- Miller LG. 1998. Herbal medicinals: selected clinical considerations focusing on known or potential drug-herb interactions. Arch Int Med. 158:2200–2211.
- Mukerjee A, Vishwanatha JK. 2009. Formulation, characterization and evaluation of curcumin-loaded PLGA nanospheres for cancer therapy. Anticancer Res. 29:3867–3875.
- Muqbil I, Masood A, Sarkar FH, Mohammad RM, Azmi AS. 2011. Progress in nanotechnology based approaches to enhance the potential of chemopreventive agents. Cancers. 3:428–445.
- Nakamura K, Yasunaga Y, Segawa T, Ko D, Moul JW, Srivastava S, Rhim JS. 2002. Curcumin down-regulates AR gene expression and activation in prostate cancer cell lines. Int J Oncol. 21:825–830.
- Niki E, Komuro E, Takahashi M, Urano S, Ito E, Terao K. 1988. Oxidative hemolysis of erythrocytes and its inhibition by free radical scavengers. J Biol Chem. 263:19809–19814.
- Rao M. 1993. Curcumin inhibits iron-dependent lipid peroxidation. Int J Pharm. 100:93–97.
- Sa G, Das T. 2008. Anti cancer effects of curcumin: cycle of life and death. Cell Div. 3:14
- Schraufstätter E, Bernt H. 1949. Antibacterial action of curcumin and related compounds. Nature. 164:456–457.
- Sharma RA, Steward WP, Gescher AJ. 2007. Pharmacokinetics and Pharmacodynamics of Curcumin. The Molecular Targets and Therapeutic Uses of Curcumin in Health and Disease. New York: Springer, pp. 453–470.
- Shishodia S, Chaturvedi MM, Aggarwal BB. 2007. Role of curcumin in cancer therapy. Curr Prob Cancer. 31:243–305.
- Sou K. 2012. Curcumin towards nanomedicine. Recent Pat Nanomed. 2:133–145.
- Tønnesen HH, Greenhill JV. 1992. Studies on curcumin and curcuminoids. XXII: curcumin as a reducing agent and as a radical scavenger. Int J Pharm. 87:79–87.
- Tønnesen HH, Kristensen S, Grinberg LN. 1994. Studies on curcumin and curcuminoids: XXV. Inhibition of primaquine-induced lysis of human red blood cells by curcumin. Int J Pharm. 110:161–167.
- Vogel A, Pelletier J. 1815. Examen chimique de la racine de Curcuma. J Pharm. 1:289–300.
- Yallapu MM, Dobberpuhl MR, Maher DM, Jaggi M, Chauhan SC. 2012. Design of curcumin loaded cellulose nanoparticles for prostate cancer. Curr Drug Metab. 13:120–128.
- Yallapu MM, Ebeling MC, Chauhan N, Jaggi M, Chauhan SC. 2011. Interaction of curcumin nanoformulations with human plasma proteins and erythrocytes. Int J Nanomed. 6:2779–2790.
- Yusra M, Duaa SS, Dalal AA. 2013. Novel probiotic bifidobacterium overcomes synergistic effect of three natural biotic omni drug and antibiotic against some UTI pathogens. Int J Sci Nature. 4:456–462.