Abstract
Mouse bone marrow mesenchymal stem cells C3H10T1/2 were divided into Ad-BMP2 (bone morphogenetic protein 2) group, Ad-VEGF165 (vascular endothelial growth factor 165) group, Ad-VEGF165 + Ad-BMP2 group, empty adenovirus group and control group. BMP2 and VEGF165 were highly co-expressed in Ad-VEGF165 + Ad-BMP2 group. Ad-BMP2 and Ad-VEGF165 + Ad-BMP2 groups, especially the latter (P < 0.05), had significantly higher expression levels of osteocalcin, osteoprotegerin, and osteopontin mRNA and OPN protein (P < 0.05). Ad-VEGF165 + Ad-BMP2 group had highest alkaline phosphatase (ALP) activity, strongest ALP staining and most calcium salt deposits (P < 0.05). Combining VEGF165 obviously enhanced the inducing effects of BMP2 on osteogenic differentiation capacity of C3H10T1/2 cells.
Introduction
Bone defects caused by traumas, infections, and tumors have become a global concern, and accelerating their repair remains challenging. Nowadays, tissue-engineered bones, which are constructed in vitro based on complexation of exogenous growth factor genes with scaffold biomaterials, have inspired clinical treatment of bone defects (Chen et al. Citation2014, Liu et al. Citation2011). Recently, bone defects and bone nonunion have been successfully treated by bone tissue engineering using seed cells, growth factors and scaffolds.
With pluripotent differentiation potential, bone marrow mesenchymal stem cells (BMSCs), which can differentiate into osteoblasts, chondrocytes, adipocytes, neurocytes and hepatic parenchymal cells, are well-established seed cells (Munir and McGettrick Citation2015). As a member of the transforming growth factor beta (TGF-β) superfamily, bone morphogenetic protein (BMP) is one of the most powerful regulatory factors that induce the osteogenic differentiation of BMSCs (Kang et al. Citation2004). Although BMP2 can induce osteogenesis and promote bone regeneration, the effects are not obvious, probably because BMPs barely facilitate ossein synthesis or proliferation of osteoblasts (Srinivasan et al. Citation2012). Vascular endothelial growth factor (VEGF) is a key factor for angiogenesis, dominantly controlling the osteogenic pathway of cartilage ossification (Peng et al. Citation2002). Therefore, in studies regarding the promoting effects of combined exogenous bone growth factors on bone regeneration, ossein synthesis as well as proliferation and differentiation of osteoblasts, it is of global interest to analyze the interactions between these factors, their roles in the healing of bone defect and the optimum dose ratios between them.
In this study, mouse BMSCs C3H10T1/2 were selected as seed cells, and the effects of combined BMP2 and VEGF165 on them were assessed, aiming to verify that combining VEGF165 obviously enhanced the inducing effects of BMP2 on the osteogenic differentiation capacity of C3H10T1/2 cells. The results may provide an eligible method for the treatment of bone defects in clinical practice.
Materials and methods
Materials
Recombinant adenoviruses Ad-BMP2 and Ad-VEGF165 as well as empty adenovirus were purchased from SinoGenoMax Co., Ltd. Mouse BMSCs C3H10T1/2 were provided by ATCC (Beijing, China). Fetal bovine serum (FBS) was bought from Hyclone (Logan, UT). Real-time PCR kit, RNA extraction kit, and PCR mix were obtained from Life Technologies (Frederick, MD). β-Actin antibody and goat anti-rabbit IgG secondary antibody were purchased from Santa Cruz (MA). Osteopontin (OPN) primary antibody was bought from Immunoway Co (Newark, DE). Alkaline phosphatase (ALP) staining kit and other quantification kits were obtained from BD (NY). Alizarin red was provided by Sigma Aldrich (Hong Kong, China). Other reagents were import-subpacks or analytically pure. Primer sequences were designed by Primer 3.0 () and synthesized by Sigma.
Table 1. Primer sequences for real-time PCR.
Culture and infection of C3H10T1/2 cells
C3H10T1/2 cells were cultured in Dulbecco’s modified Eagle’s medium containing 10% FBS and incubated at 37 °C in a 5% CO2 atmosphere. Cells in the logarithmic growth phase were inoculated into 6-well plates, and the adherent ones were divided into an Ad-BMP2 group, and Ad-VEGF165 group, an Ad-VEGF165 + Ad-BMP2 group, an empty adenovirus (Ad-null) group and a control group. Three replicate wells were set for each group. The cells were observed under an inverted fluorescence microscope 48 h after infection.
Detection of VEGF165 and BMP2 mRNA expression levels by real-time PCR
Total RNA was extracted 2 d and 7 d after infection respectively to determine the concentrations, and reverse-transcribed into first-strand cDNA that was then used as the template for real-time PCR. PCR system (20 μL): 10 μL of 2 × SYBR Green, 0.5 μL of upstream and downstream primers each, 2 μL of template and double-distilled water. PCR conditions: 95 °C for 3 min; 95 °C for 15 s, 55 °C for 30 s, 72 °C for 20 s, 40 cycles in total; melt curve, 65 °C–95 °C, with β-actin as the internal reference. Primer sequences are listed in .
Detection of osteocalcin (OC), osteoprotegerin (OPG), and OPN mRNA expression levels by real-time PCR
cDNA prepared by reverse transcription 7 d after infection was used to detect the expression levels of OC, OPN, and OPG mRNA. PCR system (20 μL): 10 μL of 2 × SYBR Green, 0.5 μL of upstream and downstream primers each, 2 μL of template and double-distilled water. PCR conditions: 95 °C for 3 min; 95 °C for 15 s, 55 °C for 30 s, 72 °C for 20 s, 40 cycles in total; melt curve, 65 °C–95 °C, with β-actin as the internal reference. Primer sequences are listed in .
Detection of OPN protein expression level by Western blot
C3H10T1/2 cells were grouped and cultured as mentioned above. Total protein was extracted 7 d after infection, loaded (50 μg/well), separated by 10% SDS-PAGE, electronically transferred to a polyvinylidene difluoride membrane (250 mA, 1 min/kD), blocked with 5% skim milk at room temperature for 1–2 h, washed three times with TBST (10 min each time), incubated overnight with rabbit anti-mouse OPN (1:500 diluted) and mouse anti-mouse β-actin (1:200 diluted) monoclonal antibodies at 4 °C, washed three times with TBST, incubated with HRP-labeled goat anti-rabbit IgG antibody (1:100 diluted) at room temperature for 1 h, washed three times again with TBST, and subjected to ECL imaging.
Quantification of ALP activity
C3H10T1/2 cells were inoculated into 24-well plates at the density of 2 × 105/well and grouped as mentioned above. Three replicate wells were set for each group. The supernatant was discarded 7 d after infection, and the cells were gently washed twice with phosphate-buffered saline (PBS) and lyzed with 1 × luciferase lysate (100 μL/well) for 5 min. The resulting suspension was transferred to Eppendorf tubes and centrifuged at 13,000 r/min for 3 min, from which the supernatant was collected (5 μL) and reacted with 5 μL of ALP substrate and 15 μL of 1 × Lupo buffer in dark for 30 min. The absorbance at 562 nm was measured using a spectrofluorimeter. The protein concentration of the remaining supernatant was determined with the BSA method to normalize ALP level.
Detection of ALP expression
Cells were grouped and cultured according to the section “Quantification of ALP activity”, and ALP expression was detected by the ALP staining method 7 d after infection. Small amounts of Fast Blue BB salt powders were added into double-distilled water to prepare a pale yellow solution into which 1/25 volume of AS-MX was added, giving the final staining solution. The cells were washed twice with PBS and incubated with the staining solution (200 μL/well) in dark at 37 °C for 1 h. After removal of the staining solution, the cells were washed with PBS and observed under a light microscope.
Detection of calcium salt deposition
Cells were grouped and cultured as mentioned above. Vitamin C and β-glycerophosphate at the final concentrations of 50 μg/ml and 10 mmol/L respectively were added 2 d after infection. After 21 d of culture, calcium salt deposition was detected by alizarin red staining. The supernatant was discarded, and the cells were washed three times with PBS, fixed with 4% paraformaldehyde for 2 h, washed twice with PBS, stained with 250 μL of 2% alizarin red–Tris-HCl staining solution (pH 8.3) at 37 °C for 30 min, rinsed with double-distilled water, and observed under the light microscope.
Statistical analysis
All data were analyzed by SPSS17.0. The data were expressed as x±s. Inter-group comparisons were performed with one-way analysis of variance, and pairwise comparisons were conducted by two-tailed least significant difference t-test. P < 0.05 was considered statistically significant.
Results
Infection of C3H10T1/2 cells with recombinant adenoviruses
As observed under the inverted fluorescence microscope, fluorescent protein was expressed 48 h after infection (), suggesting that C3H10T1/2 cells were successfully infected with the recombinant adenoviruses.
High expressions of adenovirus-mediated VEGF165 and BMP2 in C3H10T1/2 cells
Real-time PCR showed that although gene expression levels decreased 7 d after infection compared with those 5 d ago, the VEGF165 mRNA expression levels of Ad-VEGF165 and Ad-VEGF165 + Ad-BMP2 groups exceeded those of the other groups, and the BMP2 expression levels of Ad-BMP2 and Ad-VEGF165 + Ad-BMP2 groups were also higher ( and ). Therefore, VEGF165 and BMP2 were efficiently expressed in C3H10T1/2 cells through adenoviral mediation.
Table 2. VEGF165 and BMP2 mRNA expression levels detected by real-time PCR (x±s, n = 3).
Expressions of osteogenic indices
The OC, OPG, and OPN mRNA expression levels of Ad-BMP2 and Ad-VEGF165 + Ad-BMP2 groups were significantly higher than those of the other three groups 7 d after infection (P < 0.05), and the levels of the latter group also significantly surpassed those of the former group (P < 0.05). However, the Ad-VEGF165 group did not have significantly different results (). Meanwhile, Western blot showed that the Ad-VEGF165 + Ad-BMP2 group had the highest OPN expression level (). Taken together, VEGF165 alone failed to affect the osteogenic differentiation of C3H10T1/2 cells within a short time, but BMP2 managed to do so, and such effect was further boosted when combined with VEGF165.
Figure 3. Detection of osteogenic indices by Western blot. 1: Control group; 2: Ad-null group; 3: Ad-VEGF165 group; 4: Ad-BMP2 group; 5: Ad-VEGF165 + Ad-BMP2 group.

Table 3. OC, OPN, and OPG expression levels (x±s, n = 3).
Quantification of ALP activity and staining results
The ALP activities of Ad-BMP2 and Ad-VEGF165 + Ad-BMP2 groups were significantly higher than those of the other three groups, and the Ad-VEGF165 + Ad-BMP2 group showed the highest activity. The ALP activity of the Ad-VEGF165 group was not significantly different from that of Ad-null group or control group (P > 0.05) ( and ). In the meantime, there were purplish blue positive cells in Ad-BMP2 and Ad-VEGF165 + Ad-BMP2 groups, especially in the latter group. In contrast, positive cells were scarce in the other three groups (). Thus, the enhancing effects of BMP2 on ALP activity and expression were further augmented when combined with VEGF165.
Figure 4. ALP activities (x±s, n = 3). a: comparison between Ad-BMP2, Ad-VEGF165 + Ad-BMP2 groups and control, Ad-null and Ad-VEGF165 groups, P < 0.05; b: comparison between Ad-VEGF165 + Ad-BMP2 and Ad-BMP2 groups, P < 0.05.
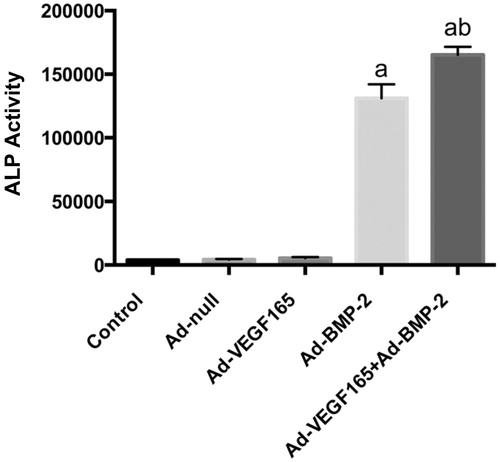
Figure 5. ALP staining results (100×). (A): Control group; (B): Ad-null group; (C): Ad-VEGF165 group; (D): Ad-BMP-2 group; (E): Ad-VEGF165 + Ad-BMP2 group.
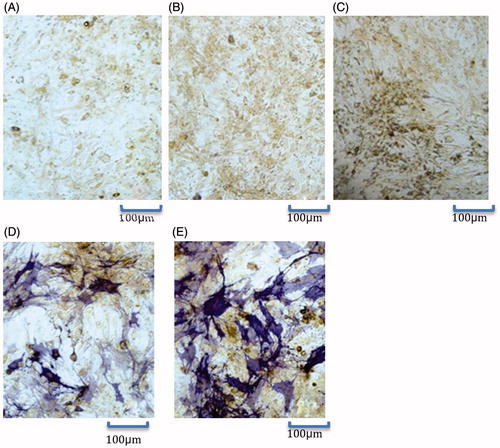
Table 4. ALP activities (x±s, n = 3).
Calcium salt deposition
Alizarin red staining showed that compared with the other three groups, there were more red calcium salt deposits in Ad-BMP2 and Ad-VEGF165 + Ad-BMP2 groups, especially in the latter group (). Accordingly, BMP2-induced calcium salt deposition in C3H10T1/2 cells was further facilitated when combined with VEGF165.
Discussion
Regulated by many cytokines, repair of bone defect is a complex process involving osteogenesis and angiogenesis. Angiogenesis accelerates bone growth by providing oxygen and nutrients, promoting metabolism and establishing a beneficial local microenvironment. Therefore, studying the mechanisms of bone growth and angiogenesis as well as their interactions can provide theoretical evidence for constructing vascularized bone scaffolds in bone tissue engineering (Kanczler and Oreffo Citation2008). With pluripotent differentiation potential, BMSCs can differentiate into osteoblasts upon in vitro induction or in the microenvironment where bones heal and repair. They are popular among tissue engineering researchers due to high migration and proliferation capacities as well as ethics debate-free sampling (Udehiya et al. Citation2013).
As a main factor that promotes bone formation, BMP predominantly controls the differentiation of osteogenic cells. Direct use of BMP or local use through vectors is conducive to the repair of bone defect (Cowan et al. Citation2007). On the other hand, VEGF can specifically promote the proliferation of vascular endothelial cells and angiogenesis (Wang et al. Citation2013). Thus, BMP and VEGF are important cytokines during bone growth. As one of the most potent cytokines for vascular endothelial cells, VEGF165 can stimulate the differentiation and proliferation of vascular endothelial precursor cells and osteocytes, and accelerate bone formation and remodeling (Wang et al. Citation2013). It has previously been reported that VEGF induced angiogenesis and benefited bone growth by cooperating with BMP4 and BMP6 (Cui et al. Citation2010, Peng et al. Citation2002). Besides, VEGF165 and different BMPs exert various synergistic effects on stem cell-induced osteogenesis (Peng et al. Citation2005, Scharpfenecker et al. Citation2007). In this study, the effects of VEGF165 on BMP2-induced osteogenic differentiation of BMSCs were evaluated.
ALP is a key enzyme at the early stage of osteoblast differentiation, which produces phosphoric acid that reacts with calcium to form calcium phosphate deposits in bones. Accordingly, increased ALP activity marks the differentiation of BMSCs into osteoblasts. The differentiation and maturation degrees of osteoblasts are commonly assessed by ALP staining and activity quantification. In this study, BMP2 enhanced the ALP activity of C3H10T1/2 cells 7 d after infection through recombinant Ad-VEGF165 and Ad-BMP2, being consistent with that reported previously (Zhang Citation2003). Although VEGF165 can promote the osteogenic differentiation of mouse BMSCs (Liu and Zhang Citation2007), we herein found VEGF165 alone failed to do so for C3H10T1/2 cells, probably because the latter cells that differentiated earlier were insensitive to VEGF165. Upon simultaneous stimulation with strong osteogenic factor BMP2, the ALP activity and expression were both augmented. OC is a non-collagen protein that exists in the bone matrix to maintain normal bone calcification and to inhibit cartilage calcification. During differentiation, ALP is expressed in osteoblast progenitor cells and osteoblasts, while OC mRNA is only expressed in mature osteoblasts. Therefore, OC has been used as the marker for the differentiation and maturation of osteoblasts (Tsuda et al. Citation2005). Moreover, the expressions of medium/advanced stage markers for osteoblast differentiation, i.e. OC, OPN, OPG, and calcium salt deposition were detected. OC and OPN expressions increased, similar to the effects of BMP2 on rabbit BMSCs reported previously (Zhang et al. Citation2015a). In short, BMP2, when combined with VEGF165, induced expressions of OC, OPN and OPG as well as calcium salt deposition in C3H10T1/2 cells more obviously.
Midy and Plouet (Citation1994) found that fetal bovine osteoblasts also bound recombinant VEGF that induced chemotactic migration. Meanwhile, the ALP activity and OC expression increased in these cells, suggesting that expression of VEGF gene potentially enhanced the differentiation of osteoblasts. Recombinant VEGF also can enhance the ALP activity of human osteoblasts (Street et al. Citation2002). By culturing osteoblast precursor cells in vitro, Marini et al. (Citation2012) found that small amounts of VEGF receptors were expressed at the beginning of differentiation. These factors were highly expressed along with cellular calcium secretion, forming calcium nodules easily upon addition of human recombinant VEGF. All these suggest that VEGF directly affects the growth, differentiation and chemotaxis of osteoblasts.
Although TGF-β promotes the expression of VEGF, BMP2, as a member of the TGF-β family, barely does so. Meanwhile, VEGF overexpression does not affect the transcriptional regulation of BMP2. Hence, VEGF and BMP2 may exert a synergistic effect via the downstream signaling transduction network (Zhang et al. Citation2015b). The Smad family, as crucial downstream transduction proteins of the TGF-β superfamily signaling pathway, plays key roles in regulating the differentiation of many types of cells. Smad1 and Smad5 are closely associated with osteoblast differentiation, the phosphorylation of which is stimulated by BMPs to form complexes with Smad4, participating in the process after entering the cell nucleus (Chen et al. Citation2012, Guo and Wang Citation2009, Liu et al. Citation2013). On the other hand, there are Smad1- and Smad5-binding elements in the VEGF promoter of zebrafish, suggesting that the BMP/Smad signaling pathway is involved in regulating VEGF expression (Crosier et al. Citation2002, He and Chen Citation2005). Therefore, we postulated that BMP2 and VEGF synergistically induced osteogenic differentiation probably by activating the BMP/Smad signaling pathway.
In summary, combining BMP2 with VEGF165 enhanced the in vitro osteogenic differentiation of BMSC that had been infected through recombinant adenoviral mediation. The findings provide experimental basis for seeking combinations of growth factors to induce osteogenesis in bone tissue engineering. Nevertheless, the mechanism still needs in-depth studies. In vivo experiments regarding the repairing effects of seed cells on bone defect are ongoing in our group to pave the way for effective clinical treatment.
Declaration of interest
The authors report no conflicts of interest. The authors alone are responsible for the content and writing of this article.
References
- Chen G, Deng C, Li YP. 2012. TGF-β and BMP signaling in osteoblast differentiation and bone formation. Int J Biol Sci. 8:272–288.
- Chen N, Bei C, Jiang L, Su M, Xu W. 2014. Progress of induced osteogenesis of bone marrow mesenchymal stem cells transfected by double-gene. Zhongguo Xiu Fu Chong Jian Wai Ke Za Zhi. 28:1540–1543.
- Cowan CM, Aghaloo T, Chou YF, Walder B, Zhang X, Soo C, Ting K, Wu B. 2007. MicroCT evaluation of three-dimensional mineralization in response to BMP-2 doses in vitro and in critical sized rat calvarial defects. Tissue Eng. 13:501–512.
- Crosier PS, Kalev-Zylinska ML, Hall CJ, Flores MV, Horsfield JA, Crosier KE. 2002. Pathways in blood and vessel development revealed through zebrafish genetics. Int J Dev Biol. 46:493–502.
- Cui F, Wang X, Liu X, Dighe AS, Balian G, Cui Q. 2010. VEGF and BMP-6 enhance bone formation mediated by cloned mouse osteoprogenitor cells. Growth Factors. 28:306–317.
- Guo X, Wang XF. 2009. Signaling cross-talk between TGF-beta/BMP and other pathways. Cell Res. 19:71–88.
- He C, Chen X. 2005. Transcription regulation of the vegf gene by the BMP/Smad pathway in the angioblast of zebrafish embryos. Biochem Biophys Res Commun. 329:324–330.
- Kanczler JM, Oreffo RO. 2008. Osteogenesis and angiogenesis: the potential for engineering bone. Eur Cell Mater. 15:100–114.
- Kang Q, Sun MH, Cheng H, Peng Y, Montag AG, Deyrup AT, et al. 2004. Characterization of the distinct orthotopic bone-forming activity of 14 BMPs using recombinant adenovirus-mediated gene delivery. Gene Ther. 11:1312–1320.
- Liu B, Li X, Liang G, Liu X. 2011. VEGF expression in mesenchymal stem cells promotes bone formation of tissue-engineered bones. Mol Med Rep. 4:1121–1126.
- Liu BL, Zhang XQ. 2007. Protein expression and osteogenic activity of bone marrow mesenchymal stem cells after human vascular endothelial growth factor 165 gene transfection. J Clin Rehabil Tissue Eng Res. 11:9242–9245.
- Liu DD, Zhang JC, Zhang Q, Wang SX, Yang MS. 2013. TGF-β/BMP signaling pathway is involved in cerium-promoted osteogenic differentiation of mesenchymal stem cells. J Cell Biochem. 114:1105–1114.
- Marini M, Sarchielli E, Toce M, Acocella A, Bertolai R, Ciulli C, et al. 2012. Expression and localization of VEGF receptors in human fetal skeletal tissues. Histol Histopathol. 27:1579–1587.
- Midy V, Plouet J. 1994. Vasculotropin/vascular endothelial growth factor induces differentiation in cultured osteoblasts. Biochem Biophys Res Commun. 199:380–386.
- Munir H, McGettrick HM. 2015. Mesenchymal stem cell therapy for autoimmune disease: risks and rewards. Stem Cells Dev. 24:2091–2100.
- Peng H, Usas A, Olshanski A, Ho AM, Gearhart B, Cooper GM, Huard J. 2005. VEGF improves, whereas sFlt1 inhibits, BMP2-induced bone formation and bone healing through modulation of angiogenesis. J Bone Miner Res. 20:2017–2027.
- Peng H, Wright V, Usas A, Gearhart B, Shen HC, Cummins J, Huard J. 2002. Synergistic enhancement of bone formation and healing by stem cell-expressed VEGF and bone morphogenetic protein-4. J Clin Invest. 110:751–759.
- Scharpfenecker M, van Dinther M, Liu Z, van Bezooijen RL, Zhao Q, Pukac L, Löwik CW, ten Dijke P. 2007. BMP-9 signals via ALK1 and inhibits bFGF-induced endothelial cell proliferation and VEGF-stimulated angiogenesis. J Cell Sci. 120:964–972.
- Srinivasan PP, McCoy SY, Jha AK, Yang W, Jia X, Farach-Carson MC, et al. 2012. Injectable perlecan domain 1-hyaluronan microgels potentiate the cartilage repair effect of BMP2 in a murine model of early osteoarthritis. Biomed Mater. 7:024109
- Street J, Bao M, deGuzman L, Bunting S, Peale FV, Jr., Ferrara N, et al. 2002. Vascular endothelial growth factor stimulates bone repair by promoting angiogenesis and bone turnover. Proc Natl Acad Sci USA. 99:9656–9661.
- Tsuda H, Wada T, Yamashita T, Hamada H. 2005. Enhanced osteoinduction by mesenchymal stem cells transfected with a fiber-mutant adenoviral BMP2 gene. J Gene Med. 7:1322–1334.
- Udehiya RK, Amarpal Aithal HP, Kinjavdekar P, Pawde AM, Singh R, Taru Sharma G. 2013. Comparison of autogenic and allogenic bone marrow derived mesenchymal stem cells for repair of segmental bone defects in rabbits. Res Vet Sci. 94:743–752.
- Wang T, Liao TA, Zhong SB. 2013. Transfection of bone marrow mesenchymal stem cells using green fluorescence protein labeled hVEGF165 recombinant plasmid mediated by liposome. Asian Pac J Trop Med. 6:739–742.
- Zhang C, Liu HM, Li QW, Chen GW, Liang X, Meng CY. 2015a. Construction and observation of tissue engineering bone with recombinant adenovirus vectors co-expressing hBMP2 and hVEGF165 infected rabbit bone mesenchymal stem cells and n-HA/PA66 scaffold in vitro. Orthop J China. 23:270–275.
- Zhang C, Liu HM, Li QW, Chen GW, Liang X, Meng CY. 2015b. Effect of overexpression of vascular endothelial growth factor 165 on the osteogenic potential of bone morphogenetic protein. Orthop J China. 23:727–732.
- Zhang ZS. 2003. Differentiation state of bone marrow stromal cells influences the expression of adenovirus mediated bone morphogenetic protein. Doctoral Dissertation. Beijing: Peking University.