Abstract
Objective To assess the effect of the fusion of rabbit bone marrow stromal cells (rBMSCs) and Nano-hydroxyapatite/poly (l-lactic acid) (Nano-HA/PLLA) in repairing the rabbit knee joint with full-thickness cartilage defect. Method The rBMSCs were isolated and cultured in vitro, and the third generation of rBMSCs was co-cultured with the Nano-HA/PLLA to construct the tissue-engineered cartilage (TEC). Eighteen New Zealand white rabbits were selected and randomly divided into three groups, namely, TEC group, Nano-HA/PLLA group, and control group. A cartilage defect model with the diameter of 4.5 mm and depth of 5 mm was constructed on the articular surface of medial malleolus of rabbit femur. General observation, histological observation, and Wakitani’s histological scoring were conducted in the 12th and 24th week postoperatively. Results The results of TEC group indicated that new cartilage tissue was formed on the defect site and subchondral bone achieved physiological integration basically. Histological and immunohistochemical analyses indicated the generation of massive extracellular matrix. In contrast, limited regeneration and reconstruction of cartilage was achieved in the Nano-HA/PLLA group and control group, with a significant difference from the TEC group (p < 0.05). Moreover, the effect of cartilage repair was positively correlated with time. Conclusion The porous Nano-HA/PLLA combined with BMSCs promoted the repair of weight-bearing bone of adult rabbit’s knee joint with cartilage defect.
Introduction
Articular cartilage has no blood vessels, nerves, and lymphatic return. Therefore, if the articular cartilage suffers from trauma or diseases, its self-repairing capacity is very limited (Harris et al. Citation2010). Bionic artificial cartilage developed based on tissue engineering technology is a breakthrough for the treatment of cartilage injury. Our previous study (Zhu et al. Citation2008) indicated that after in vitro culture and induction, the allogeneic bone marrow stromal cells (BMSCs) combined with Nano-hydroxyapatite/poly (l-lactic acid) (Nano-HA/PLLA) display a certain chondrogenetic capacity and the ability to form the functional tissue-engineered cartilage (TEC) in vitro. The objective of this article was to evaluate the efficacy of TEC in repairing the rabbit knee with cartilage defect. Our work provides an experimental basis for the clinical treatment of cartilage defect.
Materials and methods
Experimental materials and main reagents
Experimental animal
Eighteen adult New Zealand white rabbits weighing 2–2.5 kg were selected regardless of gender (provided by Guangdong Medical Laboratory Animal Center).
Main reagents and instruments
DMEM culture medium, fetal bovine serum (FBS), and trypsin were purchased from HyClone, South Logan, UT; FBS from Gibico, Grand Island, NY; insulin, dexamethasone, ITS, and transferrin from Sigma, St. Louis, MO; TGF-β1 from PeproTech, Rocky Hill, NJ; type-II anti-collagen from Invitrogen, Carlsbad, CA; DMMB assay kit from Biocolor, Carrickfergus, UK; microtome from Leica, Wetzlar, Germany; porous Nano-HA/PLLA scaffold material was provided by Central Laboratory of Department of Medicine, the Chinese University of Hong Kong, China.
Methods
Isolation, culture, and induction of rabbit BMSCs (rBMSCs)
Experimental method was referred to that of literature (Zhu et al. Citation2013) and the general procedure was as follows. One randomly selected New Zealand white rabbit of 6–8 weeks old was intravenously injected with 3% pentobarbital from the edge of ear (1 mL/kg) and then intraperitoneally injected with ketamine (0.1 g/kg). Following the successful anesthesia, bone marrow aspiration was performed from the lateral condyle of the femur, and 4–5 mL of bone marrow was collected. The bone marrow was mixed with l-DMEM containing 10% FBS and centrifuged at 1500 rpm at room temperature for 5 min. The upper layer of fat was discarded and the cells were resuspended in l-DMEM. The cell suspension was slowly added into a centrifuge tube containing Percoll separating medium of equal volume and centrifuged at 1800 rpm for 20 min. The nebulous mononuclear cell layer on the interface was collected, resuspended in l-DMEM, centrifuged at 1500 rpm at room temperature for 3 min, and rinsed for two times. Then the cells were inoculated to the culture flask at the ratio of 5 × 105 cells/cm2 and cultured with complete l-DMEM (10% FBS, 1 × 105 IU/L penicillin, and 100 mg/L streptomycin) at a 37 °C incubator containing 50 mL/L CO2. The culture medium was refreshed every 72 h. Erythrocytes, leukocytes, suspending rBMSCs, and other rBMSCs not adhering to the inner wall of culture flask were excluded. On 10–14 d, the cells grew to 80–90% confluence, and 0.25% trypsin containing 0.02% EDTA was added for cell digestion for 2–3 min in the incubator. The cells were observed under the inverted microscope. When most cells became shrunken, rounded, and fell off, 2 mL of l-DMEM medium containing 10% FBS was added to terminate the digestion. The cells were fully mixed and resuspended, centrifuged at 1500 rpm at room temperature for 3 min and rinsed with PBS for two times. Then subculture was performed with the initial density of 1 × 105/cm2 at the ratio of 1:2. The culture condition and the procedures of medium replacement were the same as above.
Preparation of scaffold material
Porous Nano-HA/PLLA scaffold material was sterilized with ethylene oxide and well packaged. Before co-culture, the cells were placed into 96-well plate and prewet with the culture medium containing 10% FBS under aseptic condition. After 24 h of immersion, the culture medium was sucked out and the cells were cultured in 37 °C incubator containing 5% CO2 for 24 h.
Construction of TEC
Sterilized porous Nano-HA/PLLA scaffold material (4.5 mm× 5 mm) was placed into a 24-well plate (1 granule/well) and prewet with the culture medium containing 10% FBS for 24 h. Then the culture medium was removed with sterile filter paper and scaffold materials were cultured in a 37 °C incubator containing 5% CO2 for 24 h. The third generation of BMSCs was digested with 0.25% trypsin and then collected. After counting, the cell concentration was adjusted to 4 × 106/mL. Thirty microliters of cell suspension was added to the experimental material dropwise until saturation. After co-culture for 24 h, the cartilage culture medium was refreshed. In subsequent culture, the culture medium was refreshed every 3 d. The scaffold material cultured for two weeks could be used for graft.
Animal grouping and modeling
The 18 New Zealand white rabbits were acclimatized for one week before the experiment. These rabbits were randomly divided into group A (TEC group), group B (Nano-HA/PLLA group), and group C (control group). The rabbits were intravenously injected with 3% pentobarbital from the edge of ear (1 mL/kg) and intraperitoneally injected with ketamine (0.1 g/kg). Following the successful anesthesia, an incision of about 4 cm was made near the patella of inner side of knee under aseptic condition. The knee was hyperextended and the patella was dislocated outward to expose the internal and external condyles of the femur. A flat head bit was used to drill on the internal condyle of the femur to build a cartilage defect model with the diameter of 4.5 mm and the depth of 5 mm. TEC and Nano-HA/PLLA were implanted into the site of defect, respectively, while the control group received no treatment. The wound was repeatedly flushed with gentamicin in normal saline solution, and then the joint capsule and skin were sutured layer by layer. After the rabbits recovered consciousness, they were sent back to the cage and fed as usual. For 3 d postoperatively, each rabbit was intramuscularly injected with 400,000 units of penicillin every day.
General observation
The major indicators of observation included the exudation of synovial fluid, hyperplasia of synovial membrane as well as the height, smoothness, continuity, glossiness, and the presence of fissure of the repaired tissue.
Histological observation
The specimen was soaked in 4% formalin (v/v) for 3 d and decalcified in 5% nitric acid (v/v) for 4–5 days. After routine dehydration, wax-filling, and embedding, the tissue was sliced and treated with HE staining, Safranine O staining, and type-II collagen immunohistochemical staining. The repair effect was assessed and quantified using Wakitani’s scoring method.
Statistical processing
Analysis of variance was performed for the scores of each group using software SPSS16.0 (Chicago, IL) and the intergroup comparison was performed with the SNK method. p < 0.05 was considered as statistically significant and the data were expressed as mean ± standard deviation (x ± s).
Results
General condition
On the day of surgery and the next day, the rabbits had few activities with lame foot. Normal diet and activities were restored after 2–3 d. There were two deaths during the experiment, which were supplemented with new rabbits.
General observation
Adhesion in the articular cavity of knee, loose bodies in knee joint, hyperplasia of synovial membrane, and osteophyte formation on the cartilage around the defect site were not observed in any cases. TEC group: In the 12th week, the defect site was filled with pale red tissue with clear boundary, and the margin was slightly sunken. In the 24th week, the defect site was repaired and well integrated with the surrounding normal cartilage, with smooth surface and similar texture (). Nano-HA/PLLA group: In the 12th week, the tissue around the defect site was ivory, with rough texture, and small pits. The defect site was basically repaired in the 24th week, but the surface was not smooth, showing a clear boundary (). Control group: In the 12th week, the tissue of the defect site was gray and a few fibers crawled to the margin where the tissue was repaired. The defect site became shallower, but the subchondral bone was still visible. In the 24th week, the fibrous tissue around the defect site was thickened, but there was still a pit in the center ().
Figure 1. (A) In the 24th week, the samples of the TEC group showed a smooth surface; the appearance was similar to the surrounding cartilage tissue, and the repaired tissue was well integrated with the surrounding normal cartilage. (B) In the 24th week, the defect site of Nano-HA/PLLA group was repaired basically, but the surface was uneven, showing an obvious boundary with the surrounding tissue. (C) In the 24th week, the defect of the control group became smaller, but there was still a pit in the center, showing an obvious boundary with the surrounding tissue.
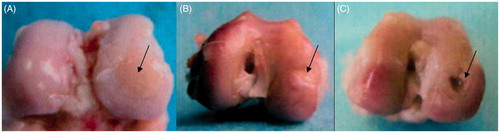
Histological observation
TEC group: In the 12th week, the HE staining result indicated an abundant number of cells, but a lack of zonal arrangement of typical chondrocytes. The porous scaffold was clearly visible and the cartilage-like tissue was found on the surface of the defect site. The grafting area treated with Safranine O staining presented the metachromasia of sulfated glycosaminoglycans. HE staining result in the 24th week showed the increase of chondrocytes and secreted matrix. The pores of scaffold were filled, but there was fissure on the margin of one side of the grafting area, with distinct tidal line (). The matrix showed red color and the subchondral bone was green after Safranine O staining. It was observed that there were slight fissures on one side and a calcified interface between the cartilage layer and the bone layer ().
Figure 2. (A) In the 24th week, the chondrocytes stained by HE were increased in the TEC group. The matrix increased, and the pores of the scaffold were filled, but there was fissure on one side of the integrated area, with an obvious tidal line (×40). (B) In the 24th week, the cells stained by HE in the Nano-HA/PLLA group were decreased, with poor cellular layer. The surface tissue was flimsy, with no matrix of chondrocytes. The pores of the scaffold material were visible, with no tidal line (×40). (C) In the 24th week, HE staining of the control group showed that the repaired tissues were mainly threadlike fibers. The surface was uneven, and there was still a pit in the center, with no secretion of cartilage matrix (×40).
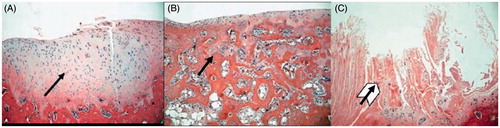
Figure 3. (A) In the 24th week, Safranine O staining of TEC group indicated the increase of matrix secreted by chondrocytes, with the matrix showing red color and the subchondral bone the green color. There were slight fissures on one side (×40). (B) In the 24th week, Safranine O staining of the Nano-HA/PLLA group showed that the surface tissue was thin and dominated by fibrocytes. The pores of the scaffold were visible, with no matrix secreted by the chondrocytes (×40). (C) In the 24th week, Safranine O staining of the control group indicated that the surface tissue was mainly composed of threadlike fibers and sunken in the center, with no matrix of chondrocytes (×40).
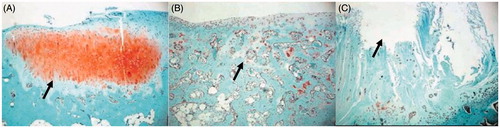
Nano-HA/PLLA group: The samples of the 12th week indicated the existence of porous scaffold but fewer cells in round shape and poor cellular layer. HE and Safranine O staining both showed no deposition of cartilage matrix. In the 24th week, HE staining showed that the number of cells was still small, and the repaired tissue was flimsy and smooth. But there was no chondrocyte layer or cartilage matrix. The pores of the porous scaffold were visible, with no tidal line (). Safranine O staining showed few cells on the surface but no matrix secreted by chondrocytes and there was no tidal line ().
Control group: The samples of the 12th week showed irregular surface. There were fibrous tissues around the defect site, with no cartilage matrix stained. The samples of the 24th week were dominated by threadlike fibers, showing uneven surface and no deposition of the cartilage matrix ( and ).
Immunohistochemical staining
In the TEC group, the type-II collagen immunohistochemical staining of the repaired tissue in the defect site showed positive result in the 12th week, and the staining effect was enhanced in the 24th week (). In the Nano-HA/PLLA group and control group, there was almost no presentation of type-II collagen in the repaired tissue in the 12th week. In the 24th week, there was still no expression of type-II collagen in the entire defect site ( and ).
Figure 4. (A) In the 24th week, the type-II collagen immunohistochemical staining of the TEC group showed strongly positive result, and the scope of staining was consistent with that of HE staining in the 24th week (×40). (B) In the 24th week, the type-II collagen immunohistochemical staining of the Nano-HA/PLLA group showed that the pores of scaffold were still visible (×40). (C) In the 24th week, the type-II collagen immunohistochemical staining of the control group showed that the center was sunken and the margins were uneven (×40).
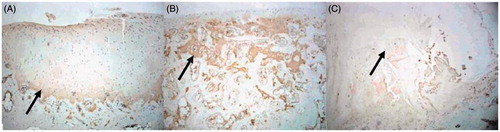
Pathological scoring
In the 24th week, the Wakitani score of TEC group, Nano-HA/PLLA group, and control group was 2.2 ± 0.837, 7.4 ± 1.144, and 8.2 ± 1.225, respectively. The result of TEC group was superior to that of other groups at the same time point, with statistically significant difference (p < 0.05, ). The scores of the TEC group at different time points showed significant differences (p < 0.05, ).
Table 1. Wakitani’s histological score (x ± SD, n = 6).
Discussion
There is still no perfect repair method for articular cartilage injury. Most of the existing clinical repair methods have ideal short-term effect, but the long-term effect is uncertain or even deteriorated (Zhu et al. Citation2014). The development of tissue engineering technology provides a new approach to treat the injured articular cartilage. The objective of this work was to provide an experimental basis for the clinical repair of bone with cartilage defect (Wang et al. Citation2004).
The injured articular cartilage is difficult to be repaired due to the deficiency of stem cells possessing seed function such as mesenchymal stem cells (MSCs). In this article, the rabbit MSCs were isolated, cultured in vitro, and combined with Nano-HA/PLLA, to construct TEC. General observation indicated that the defect site of TEC group was filled with pale red tissue in the 12th week, but pits could be still found. In the 24th week, the tissue was basically repaired and the defect site was integrated with the surrounding normal cartilage. The histochemical observation showed that the number of cells of group A was increased in the 12th week and the tissue had grown into the porous scaffold, suggesting the deficiency of chondrocytes. In the 24th week, some cartilage-like tissues could be found on the defect site. After immunohistochemical staining, the type-II collagen of extracellular matrix in the group A showed the brown color, which means strong positive expression.
The differences of the three groups were as follows. For the TEC group, the injured area was repaired basically in the 24th week and its surface was smooth. The cartilage-like tissues were increased and well integrated with the surrounding normal tissue. For the Nano-HA/PLLA group, although the injured area was repaired basically and became smooth in the 24th week, there was no deposition of cartilage matrix, with the ingrowth of a few cells into the porous scaffold. For the control group, the injured area was not integrated with the surrounding tissue in the 24th week and the surface was uneven, with no deposition of cartilage matrix. The experimental result of TEC group showed strong capability of tissue repair and fusion and regeneration. This was due to the fusion of BMSCs and Nano-HA/PLLA.
Nano-hydroxyapatite (Nano-HA) has high biocompatibility (Reddi et al. Citation2011, Radin and Rose Citation1986), cell adhesive ability, and strong toughness (Roberts et al. Citation2009). Literature (Barkarmo et al. Citation2013) indicated that this material plays an important role in the repair of articular cartilage and can reduce the friction and external impact on cartilage, which was consistent with the finding of this article (Yan-Zhong et al. Citation2011). In in vitro culture of MSCs, the cells can effectively adhere to the porous Nano-HA/PLLA scaffold with satisfactory growth and proliferation. This means that this material is effective in in vitro culture of MSCs.
In the TEC group, the type-II collagen immunohistochemical staining of the repaired tissue indicated that the type-II collagen of extracellular matrix near the outer layer was strongly positive and the scope of staining was the same as that of HE staining. There was no presentation of type-II collagen in the Nano-HA/PLLA group and control group. This means that the cartilage was basically regenerated in the TEC group, while the repaired tissue in the other two groups failed to grow to hyaline cartilage.
In immunohistochemical staining, type-II collagen serves as an indicator for the repair of cartilage. This provides guidance for the clinical application in the repair of human cartilage and has a certain clinical value for the study of osteoarthritis of the young people (Glowacki and Mizuno Citation2008).
The result of TEC group indicated that after the fusion of MSCs and Nano-HA/PLLA, the defect site was repaired, and new cartilage was formed and basically integrated with the surrounding normal tissue. The histological and immunohistochemical staining revealed the formation of type-II collagen of extracellular matrix, which suggests the function of cartilage regeneration and reconstruction. The results of the Nano-HA/PLLA group and control group indicated that the Nano-HA/PLLA material alone could not effectively repair the bone with cartilage defect (Harris et al. Citation2010). In conclusion, it was confirmed in this article that through the fusion of rBMSCs and Nano-HA/PLLA composite material, evident efficacy was achieved in the repair of rabbit knee joint with full-thickness cartilage defect. This outcome is important for the repair of weight-bearing bone of rabbit knee joint with cartilage defect.
Funding information
This study received financial support from Guangdong Science and Technology Project (the project number is 2013B021800100 and 2015A020212001) Shenzhen Science and Technology Project (the project number is CXZZ20130321152713220 and JCYJ20140414170821164).
Disclosure statement
The authors have no conflict of interest.
References
- Barkarmo S, Wennerberg A, Hoffman M, Kjellin P, Breding K, Handa P, Stenport Vet al. 2013. Nano-hydroxyapatite-coated PEEK implants: a pilot study in rabbit bone. J Biomed Mater Res Part A. 101:465–471.
- Glowacki J, Mizuno S. 2008. Collagen scaffolds for tissue engineering. Biopolymers. 89:338–344.
- Harris JD, Siston RA, Pan X, Flanigan DC. 2010. Autologous chondrocyte implantation: a systematic review. J Bone Joint Surg Am Vol. 92:2220–2233.
- Radin EL, Rose RM. 1986. Role of subchondral bone in the initiation and progression of cartilage damage. Clin Orthopaed Relat Res. 213:34–40.
- Reddi AH, Becerra J, Andrades JA. 2011. Nanomaterials and hydrogel scaffolds for articular cartilage regeneration. Tissue Eng Part B Rev. 17:301–305.
- Roberts S, Menage J, Sandell LJ, Evans EH, Richardson JBet al. 2009. Immunohistochemical study of collagen types I and II and procollagen IIA in human cartilage repair tissue following autologous chondrocyte implantation. Knee. 16:398–404.
- Wang J, Xia WY, Cui Let al. 2004. Construction of allogeneic cartilage utilizing chondrocytes from difference phase. Chin J Exp Surg. 21:22–23.
- Yan-Zhong Z, Yan-Yan H, Jun Z, Shai-Hong Z, Zhi-You L, Ke-Chao Z. 2011. Characteristics of functionalized nano-hydroxyapatite and internalization by human epithelial cell. Nanoscale Res Lett. 6:600.
- Zhu WM, Chen K, Lu W, Sun Q, Peng L, Fen W, et al. 2014. In vitro study of nano-HA/PLLA composite scaffold for rabbit BMSC differentiation under TGF-β1 induction. In Vitro Cell Dev Biol Anim. 50:214–220.
- Zhu WM, Huang JH, Lu W, Sun Q, Peng L, Fen W, et al. 2013. Performance test of Nano-HA/PLLA composites for interface fixation. Artif Cells Nanomed Biotechnol. 42:331–5.
- Zhu WM, Xiao JD, Wang DP, Liu J, Xiong J, Liu L, Zhang X, Zeng Yet al. 2008. Experimental study of nano-HA artificial bone with different pore sizes for repairing the radial defect. Int Orthopaed. 33:567–571.