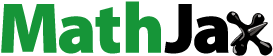
Abstract
Purpose To investigate the effect of CellCept nanoliposomes on Adriamycin-induced nephrotic syndrome in rats. Methods To model nephrotic syndrome, rats were injected with 6.5 mg/kg of Adriamycin in the tail vein. The rats were randomly divided into three groups, including a control group, a free mycophenolate mofetil (MMF)-treated group, and a liposome-encapsulated MMF-treated group. Five weeks after the Adriamycin treatment, the free MMF-treated group received CellCept while the liposome-encapsulated MMF-treated group received the CellCept nanoliposomes for 2 weeks. The general condition of the animals was observed, which included urine volume over 24 h, urine protein levels, and serum biochemical indexes. Renal morphology was also observed. Results The level of urine protein over 24 h was increased in the control group, while plasma albumin (ALB) was decreased. The total cholesterol (TC) and triacylglycerol (TG) levels increased significantly (P < 0.05, P < 0.01). The pathological examination of the kidneys showed some abnormalities. In contrast, these parameters were improved significantly in the free mycophenolate mofetil (MMF)-treated and liposome-contained mycophenolate mofetil (MMF)-treated groups. Conclusion The CellCept nanoliposomes have a good therapeutic effect on Adriamycin-induced nephrotic syndrome in rats.
Introduction
The kidneys are one of the vital organs in the human body and function in urine production and internal secretion (Morin et al. Citation1977). They are able to generate, among others, prostaglandins, renin and kinins (Odaka et al. Citation2015, Simic et al. Citation2013). In addition, they also play a role in the degradation of internally secreted molecules (Odaka et al. Citation2015). They not only support a stable environment in the organism but also sustain the metabolism (Hacihamdioğlu et al. Citation2014, Morin et al. Citation1977, Neuhaus and Laube Citation2012).
Nephrotic syndrome (NS) is mainly caused by several diseases, and the clinical symptoms include proteinuria, hypoproteinaemia, high cholesterol and anasarca. Currently, glucocorticoids and immune system inhibitors are widely used to treat NS, with only a few positive effects (Bo and Yuan Citation2012, Cadnapaphornchai et al. Citation2014, Scarpioni et al. Citation2015, Svenningsen et al. Citation2015). However, due to their negative effect on hormone production, their toxicity, resistance and poor reliability, the prospective use of glucocorticoids and immune system inhibitors in the clinic is limited (Cadnapaphornchai et al. Citation2014, Chen and Mao Citation2015, Cheong et al. Citation2012). Therefore, the development of new technologies is urgently needed to successfully treat NS. Our study shows that the CellCept nanoliposomes have a beneficial therapeutic effect on the Adriamycin-induced nephrotic syndrome in rats.
Materials and methods
Materials
Mycophenolate Mofetil Capsules (CellCept) were purchased from Roche Pharmaceuticals Ltd (Shanghai, China). Doxorubicin and Cholesterol (Chol) were obtained from Sigma (St. Louis, MO). 1,2-Dioleoyl-sn-glycero-3-phosphocholine (DOPC) and 1,2-distearoyl-sn-glycero-3-phosphoethanolamine-N-[(polyethylene glycol)-2000] (DSPE-PEG-NH2) were purchased from Avanti Polar Lipids, Inc. (Alabaster, AL). All other chemicals were of reagent grade and were used as received. Ten Sprague Dawley (SD) rats aged between 3 and 4 months were purchased from the Shanghai Experimental Animal Center.
Experimental methods
Preparation of the CellCept nanoliposomes
The CellCept nanoliposomes were prepared by a modified solvent extraction/evaporation double-emulsion method. Briefly, 0.25 g of CellCept was dissolved in 6.0 mL of a deionized water aqueous phase, which was distributed into the internal aqueous phase and external aqueous phase in certain proportions. The organic phase was prepared by dissolving 12 mg of DOPC, 8.1 mg of Chol and 1 mg of DSPE-PEG-NH2 in 3.0 mL of dichloromethane solution. Then, the internal aqueous solution was slowly mixed dropwise under sonication (Ningbo Xinyi Ultrasonic Equipment Co., Ltd., JY92-IIN, Ningbo, China) for 120 s at a 100 W output level, into the organic phase to form the stabilized original emulsion. Subsequently, the formed original emulsion was poured into the external aqueous phase, which was sonicated for 180 s at a 200 W output level. The double emulsion was stirred in a rotary vacuum evaporator (Shanghai Jukun Vacuum Instrument Equipment Co. Ltd., R210D, Shanghai, China) to allow the complete evaporation of the organic solvent.
Characterization of the CellCept nanoliposomes
Particle size and distribution uniformity will directly influence the behavior of liposomes in the tissues of an organism and are also important variables to assess for the characterization of the biochemical, biophysical and pharmacodynamic properties of liposomes. In this study, we applied advanced technologies, such as transmission electron microscopy (TEM), Zeta electronic potential, and particle size analysis to characterize our custom-made liposomes.
Transmission electron microscopy images of the liposomes
The CellCept nanoliposomes were placed on a copper grid coated with film and observed using transmission electron microscopy (JEOL Ltd., JEOL-100C XII, Tokyo, Japan).
Particle size distribution and zeta potential of the liposomes
Approximately 0.2 mL of liposomes were diluted into 2.5 mL of water immediately after preparation. The average particle size and zeta potential were determined by quasielastic laser light scattering using a Malvern Zetasizer system (Malvern Instruments Limited, Mastersizer 2000, Malvern, Britain) at 25 °C.
Stability testing
For the successful delivery of liposomes, their stability must be certified. Therefore, we compared several parameters between the CellCept nanoliposomes and blank liposomes. The experiments were divided into two groups: the blank liposomes and the CellCept nanoliposomes.
Test method: During the testing experiments, the temperature was maintained at 25 ± 2 °C and the humidity at 60 ± 10%. A saturated sodium bromide solution was placed at the bottom of the drier (relative humidity of 58%, 20 °C) and the sample was added. The dryer was closed and placed in an incubator at 25 ± 2 °C.
The liposomes were conserved at 25 ± 2 °C with a humidity level of 60 ± 10%. The average particle size, potential, encapsulation efficiency, leakage rate and phospholipid content were measured at 15 days, 1 m, 2 m and 3 m, respectively. The cholesterol level was detected via an enzymatic method using the total cholesterol kit – CHO.
Detection of the encapsulation efficiency and drug-loading ratio
(a) The encapsulation efficiency refers to the amount of liposomes encapsulating the active material. The free and encapsulated active substances were separated and detected by gel column chromatography, centrifugation–filtration, and dialysis methods. The formula 2–1 was:
(b) The drug loading capacity of the liposomes directly affects the clinical dosage of the drug. Therefore, a higher drug loading capacity facilitates the fulfillment of clinical needs. The drug-loading capacity (formula 2–2) is usually related to the nature of the drug, with lipophilic drugs or hydrophilic drugs being easier to encapsulate into the liposomes.
In this formula, WA is the CellCept mass contained in the liposomes and WB is the sum of the polymer mass and the encapsulated CellCept mass.
The method to detect the encapsulation efficiency required 0.5 mL of CellCept to be loaded into long-circulating liposomes. The preparation was then centrifuged at 15,000 r/min for 30 min, and the CellCept content of the supernatant was detected by UV absorbance.
Sustained-release curve
The in vitro drug release profile of the CellCept nanoliposomes was determined as follows: 0.5 mL (1.0 mg/mL) of a CellCept nanoliposome solution was added to a dialysis bag with 10.0 mL of phosphate buffered saline (PBS) solution (0.1 M, pH 7.4) and incubated at 37 ± 0.5 °C with shaking at 100 rpm. At the designated time intervals, aliquots of buffer solution from the dialysis bag were removed for testing. The amount of CellCept released from the CellCept nanoliposomes was determined using UV-Vis spectroscopy (Shanghai Precision & Scientific Instrument Co., Ltd., 752N, Shanghai, China).
Modeling and drug administration experiments in rats
Twenty rats were randomly divided into three groups: the control treatment group (6 rats), the free mycophenolate mofetil (MMF)-treated group (7 rats) and the liposome-encapsulated MMF-treated group (7 rats).
The rats were adaptively fed for 1w, and the nephrotic syndrome model was generated according to the method of Bertain. Adriamycin (6.5 mg/kg) was injected to the rats via the tail vein for 2 w, without anesthesia. After 5 w, the level of urinary proteins was recorded for quality control. The rats in the free MMF-treated group were injected with CellCept and the rats in the liposome-encapsulated MMF-treated group were injected with CellCept nanoliposomes for 2 weeks. The animal body weight, presence of urinary proteins, rate of urinary protein excretion >24 h and blood biochemical indexes were recorded. The animals were euthanized at the end of the 7th week, and blood was collected from the abdominal aorta. The kidneys were removed under aseptic conditions. The right kidney was fixed in 4% formalin and routine paraffin embedding and sectioned followed by HE staining.
Monitored endpoints
The survival and general health condition of the rats was monitored, including body weight, food consumption, fur glossiness, activity level, presence of edema, and stool appearance. The quantitative determination of urinary proteins over a 24 h period was performed as follows: the rats were fed individually in metabolic cages and the urine was collected >24 h. The total urine volume was recorded and the qualitative determination of urinary proteins was performed after 5 w and 7 w, respectively. The urinary proteins produced in 24 h were measured after 7 w. The biochemical analysis of the blood was performed using an automatic biochemical analyzer. The analyzed parameters included the total plasma proteins, albumin, total cholesterol, triglyceride glycerol, urea nitrogen, and creatinine levels. The renal morphology examination of the Hematoxylin & Eosin (HE)-stained kidney sections included the glomerulus, the renal interstitium, and the pathological changes of the renal tubules.
Statistical processing
Data were analyzed using the SPSS 16.0 software (Chicago, IL). One-way ANOVA was used to analyze the data. Measurement data are shown as the means ± SEM. A value of P < 0.05 indicated a statistically significant difference.
Results
TEM imaging of CellCept nanoliposome
TEM images showing that the size of the spherical CellCept nanoliposomes was approximately 350 nm.
Particle size distribution and zeta potential of the liposomes
As shown in , and , the particle size of the CellCept nanoliposomes was homogeneous and the surface potential of the CellCept nanoliposomes was −20.8 ± 9.78 mV. The particle size of the drug carriers was closely related to their distribution in the body. Particles of a different diameter had a different application value. The electrical behavior at the surface of the carrier particles can greatly influence the outcomes of gene therapy, drug adsorption and dispersion in solution.
Table 1. Particle size distribution and zeta potential of the liposomes
Stability evaluation of the CellCept nanoliposomes
Maintaining liposome stability can prolong the storage life of liposome-encapsulated drugs, prolong the drug circulating time in the blood and improve the targeting efficiency of the liposome. A longer liposome preservation time before expiration ensures a higher market value and better safety profile of the liposomes, which represents a great benefit for research and development companies and patients alike.
shows that the surface potential of the liposome prepared in this study was of negative value. The average particle size ranged between 200 and 600 nm. Over time, the particle size increased possibly because of a swelling effect of the liquid state. The particle size of the CellCept nanoliposomes was slightly larger than that of conventional liposomes, which may have been caused by CellCept loading. The cholesterol leakage rate of the CellCept nanoliposomes initially increased and then decreased, being mainly affected by the content of cholesterol in the liposomes. The leakage rate of CellCept was significantly increased and one reason may be the restriction by CellCept loading in liposomes. Alternatively, the decrease in the phospholipid content altered the structure of the liposomes, which accelerated the leakage of the drug. The CellCept exudation could still be detected after 3 months, which also provided evidence of the sustained-release effect of the liposomes.
Table 2. Changes in average particle size, potential, encapsulation efficiency, leakage rate and phospholipid content at different times
Encapsulation efficiency and drug-loading rate
According to the formulas 2–1 and 2–2, the drug loading and encapsulation rates were 6.03 ± 0.17% and 90.65 ± 2.68%, respectively. The encapsulation efficiency reached more than 90%, which indicates that the long-circulating liposomes prepared by the reversed phase evaporation method could achieve the expected effect.
Sustained-release curve
shows that the cumulative amount of released CellCept increased gradually. Indeed, CellCept release showed an almost linear growth trend over a 10 h period, then slowly increased and stabilized around the 7th day, a duration that may be related to the amount of drug loaded in the liposomes.
General situation
After Adriamycin was intravenously injected in the rats of the control and liposome-encapsulated MMF groups for 2 w, two of the rats died, while one rat died in the free MMF group. The food intake of the rats was reduced, their activity level was slower, their response was poor, their amount of urine was reduced, their extremities were black and blue, and their fur was dull and fell out easily.
Changes of blood biochemical parameters in three groups
Compared with the control and the free MMF-treated groups, the level of total plasma proteins (TP) and plasma albumin (ALB) in the liposome-encapsulated MMF-treated group was significantly increased (P < 0.01), while the levels of total cholesterol (TC), triacylglycerol (TG), blood urea nitrogen (BUN) and creatine (CR) were significantly decreased (P < 0.05, P < 0.01), as shown in .
Table 3. Comparison of the blood biochemical parameters between the three groups (n = 5, presented as the mean ± SEM)
Changes of body weight, urine volume >24 h, and amount of urinary proteins between the three groups
The CXC chemokine ligand 16 (CXCL16) is the only chemokine existing in two forms: the transmembrane and soluble forms. Research showed that CXCL16 can regulate cell chemotaxis and inflammatory kidney disease and can be used as scavenger receptor for oxidized low-density lipoproteins (oxLDLs). Compared with the control and the free MMF-treated groups, the body weight and urine volume >24 h in rats of the liposome-encapsulated MMF-treated group was increased significantly (P < 0.01), while the urinary protein levels >24 h and the CXCL16 levels were significantly reduced (P < 0.01), as shown in
Table 4. Body weight, urine volume >24 h and urinary protein content in the three groups
Comparison of the renal histomorphological features between the three groups
As shown in , the level of damage in the kidneys of animals from the control group was worse than that of the liposome-encapsulated MMF-treated group. Simultaneously, in the control group, it was obvious that focal segmental glomerulus sclerosis was present, marked by a significant amount of inflammatory cell infiltration in the glomerulus, increased adhesiveness and thickness of the saccular wall, atrophy of the glomerulus in the sclerotic zone, reduced capillary lumen, partially fused foot process, disturbed organization of the kidney tubules, occasional kidney tubules necrosis, visible zones of partial multifocal kidney tubule expansion or reduction, and lymphocyte infiltration in the interstitial tissue with fibrosis. In the free MMF-treated group, the following changes were observed: a mild hyperplasia of the mesangial area, a partial fusion of the foot process, a clear renal tubules structure with areas of visible expansion, and an obvious lymphocytic infiltration of the mesenchyme. In the liposome-encapsulated MMF-treated group, the following changes were observed: a mild hyperplasia of the mesangial area, a partial fusion of the foot process, a clear renal tubules structure, with interstitial lymphocytic infiltration.
Discussion
According to investigators worldwide, chronic renal failure is a common disease, that is, harmful to public health. (Hodson et al. Citation2015, Zhang et al. Citation2011) The main clinical symptom of patients suffering from chronic kidney diseases is the proteinuria, that is, observed from an early stage of kidney damage (Bo and Yuan Citation2012, Svenningsen et al. Citation2015).
CellCept, the 2-ethyl ester derivative of mycophenolic acid (MPA), can be metabolized back into MPA by the body. MPA inhibits the formation of de novo guanosine nucleotides and suppresses the immune system in vivo. Indeed, by inhibiting the formation of guanosine nucleotides via its metabolite MPA, CellCept selectively stops lymphopoiesis of T and B cells, which is beneficial for inhibiting the rejection of transplanted organs and control autoimmune diseases. Consequently, CellCept is currently used in the clinic for immunosuppression after organ transplantation and in the presence of autoimmune diseases. In addition to the negative toxicity effects, the wide distribution in the human body and the rapid excretion of CellCept by the organism restrict its positive effect. Therefore, in clinical practice, the therapeutic benefits of CellCept are limited.
Liposomes are small spheres formed of one or multiple bimolecular layers of lipids that are used in a carrier reagent. In addition, liposomes are used for drug delivery and immunodetection. Currently, some anti-cancer medicines and new vaccines encapsulated into liposomes are sold, including the liposome-encapsulated forms of amphotericin B, Adriamycin, paclitaxel and cisplatin.
Liposome-encapsulated formulations represent a great breakthrough in the field of medical research. In addition, liposome-encapsulated formulations have a good therapeutic advantage over the corresponding free drug formulations. As a drug delivery system, the particle size and encapsulation of liposome-based drugs are important indicators to evaluate if the system is good or bad. Indeed, a small particle size and a high encapsulation of the drug support a good therapeutic effect.
Long-circulating liposomes, also referred to as sterically stabilized liposomes or stealth liposomes, are modified to display a lower interaction with the constituents in plasma or an enhanced stability in the blood via the addition of a certain proportion of glycolipids to the composition of the liposomes membranes (e.g. the single ganglioside salivary glands, GM1) or via the incorporation of molecules with multi-hydroxyl groups on the phosphoric acid molecule (e.g. polyethylene glycol or PEG). By adding hydrophilic polysaccharide or multi-hydroxyl groups on the liposome surface, the long-circulating liposomes can prolong the presence of the drug in the blood and avoid phagocytosis by the reticuloendothelial system (RES). Additionally, liposome modifications can allow sufficient time for the drug to reach its targeted site. Therefore, the encapsulation of drugs into long-circulating liposomes produces a better therapeutic effect and less negative effects than the free drug.
In our experiments, all of the induced nephropathy rats displayed changes in the blood biochemical criteria and morphological characteristics of the NS pathology in the glomerulus and renal tubules. This indicates that Adriamycin-induced nephropathy is caused by disturbing the lipid metabolism and inducing kidney damage to a variable extent. In comparison to the rats from the control and free MMF-treated groups, the injection of CellCept nanoliposomes remarkably corrected the disturbed thromboxane levels, explicitly reduced the pathological damages, reduced the proteinuria and the CXCL16 levels, increased the plasma albumin levels, lowered the circulating total cholesterol and triacylglycerol significantly and rescued the renal histopathological features. These therapeutic benefits support the notion that the CellCept nanoliposomes are significantly superior to the free CellCept formulation at reducing kidney damage.
In summary, the CellCept nanoliposome decreased the proteinuria, cholesterol levels, and coagulation, while promoting the dissolution of fibrosis and increasing the renal blood flow and the glomerular filtration rate. Our results suggest that the CellCept nanoliposomes represent a good potential treatment approach in the clinic.
Funding information
This work was funded by Science and Technology Program of Yantai, China [grant number 2013WS237].
Ethical approval
All applicable international, national, and/or institutional guidelines for the care and use of animals were followed.
Disclosure statement
The authors declare no competing financial interest.
References
- Bo Y, Yuan L-p. 2012. Glomerular expression of apelin and its association with proteinuria. Indian J PediatrIndian. 79:1028–1032.
- Cadnapaphornchai MA, Tkachenko O, Shchekochikhin D, Schrier RW. 2014. The nephrotic syndrome: pathogenesis and treatment of edema formation and secondary complications. Pediatr Nephrol. 29:1159–1167.
- Chen J-Y, Mao J-H. 2015. Henoch–Schönlein purpura nephritis in children: incidence, pathogenesis and management. World J Pediatr. 11:29–34.
- Cheong HI, Kang HG, Schlondorff J. 2012. GLCCI1 single nucleotide polymorphisms in pediatric nephrotic syndrome. Pediatr Nephrol. 27:1595–1599.
- Hacihamdioğlu DÖ, Gökhan A, Cihan M, Mul K, Ferhan K, Süleymanoğlu S. 2014. Cyclosporin A-induced posterior reversible encephalopathy syndrome in an adolescent with steroid-resistant nephrotic syndrome. Int Urol Nephrol. 46:2055–2056.
- Hodson EM, Hahn D, Craig JC. 2015. Corticosteroids for the initial episode of steroid-sensitive nephrotic syndrome. Pediatr Nephrol. 30:1043–1046.
- Morin RJ, Davidson WD, Joan Rorke S, Guo LSS. 1977. Lipid metabolism in plasma, liver, and adipose tissue of rats with experimental chronic nephrotic syndrome. Lipids. 12:208–214.
- Neuhaus TJ, Laube GF. 2012. Weight dosing of steroids in nephrotic syndrome. Pediatr Nephrol. 27:505.
- Odaka J, Kanai T, Uehara R, Kusano E, Yamagata T. 2015. Seasonal variation in first episode of childhood idiopathic steroid-sensitive nephrotic syndrome and adult minimal change nephrotic syndrome. Clin Exp Nephrol. 19:146–147.
- Scarpioni R, Rigante D, Cantarini L, Ricardi M, Albertazzi V, Melfa L, Lazzaro A. 2015. Renal involvement in secondary amyloidosis of Muckle–Wells syndrome: marked improvement of renal function and reduction of proteinuria after therapy with human anti-interleukin-1β monoclonal antibody canakinumab. Clin Rheumatol. 34:1311–1316.
- Simic I, Tabatabaeifar M, Schaefer F. 2013. Animal models of nephrotic syndrome. Pediatr Nephrol. 28:2079–2088.
- Svenningsen P, Andersen H, Nielsen LH, Jensen BL. 2015. Urinary serine proteases and activation of ENaC in kidney—implications for physiological renal salt handling and hypertensive disorders with albuminuria. Pflügers Archiv Eur J Physiol. 467:531–542.
- Zhang G, Li Q, Wang L, Chen Y, Zhang W, Yang H. 2011. The effects of inflammation on lipid accumulation in the kidneys of children with primary nephrotic syndrome. Inflammation. 34:645–652.