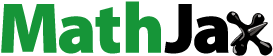
Abstract
Context Isradipine is an effective calcium channel blocker used in the management of hypertension. It undergoes extensive first pass metabolism and has low oral bioavailability. Hence we attempted to develop isradipine-loaded invasomes. Objective The purpose of this work was to prepare and characterize invasomes carrier for isradipine, and to evaluate the optimized formulation obtained for pharmacodynamic study. Materials and methods Isradipine-loaded invasomes were prepared by conventional thin layer evaporation technique using Phospholipon® 90G, β-citronellene (terpene) and ethanol. Prepared formulations were characterized in terms of size, size distribution, morphology, entrapment efficiency, and antihypertensive activity. Results and discussion It was observed that prepared isradipine-loaded invasomes delivers ameliorated flux, reasonable entrapment efficiency, and more effectiveness for transdermal delivery. The optimized formulation presented the particle size of 194 ± 18 nm, entrapment efficiency (88.46%), and attained mean transdermal flux of 22.80 ± 2.10 μg/cm2/h through rat skin. Confocal laser scanning microscopy revealed an enhanced permeation of Rhodamine-Red-loaded isradipine invasomes to the deeper layers of the rat skin. During antihypertensive study, the treatment group showed a substantial and constant decrease in blood pressure, for up to 24 h. The isradipine invasomes formulation was found to be effective, with a 20% reduction in blood pressure by virtue of better permeation through Wistar rat skin. Conclusion It was concluded that the developed isradipine invasomes accentuate the transdermal flux and the results obtained encouraged the use of the isradipine-loaded invasomes as the formulation for the potential management of hypertension.
Introduction
Transdermal drug delivery systems are designed so that the drug can be delivered at a predetermined and controlled rate (Ahad et al. Citation2015b, Citation2016), which is particularly conducive to treating chronic disorders like hypertension (Ahad et al. Citation2013a). Correspondingly, the adverse effects and inconvenience concomitant with oral route are circumvented. In addition, a good number of antihypertensive drugs undergo extensive first-pass metabolism which too can be avoided by transdermal therapy. Hence, antihypertensive agents for therapeutic and prophylactic usages have been subjected to transdermal investigation.
Essential attempts have been made to develop transdermal systems, to circumvent the drawbacks of conventional drug delivery of antihypertensive drugs (Ahad et al. Citation2013a, Citation2015a, Citation2016, Gungor and Ozsoy Citation2012, Selvam et al. Citation2010). The biggest challenges in the development of transdermal systems are to mitigate the barrier property of skin without causing harmful effects, principally local irritation. The obstacle function of the skin is chiefly due to the stratum corneum, which is responsible for the poor penetration of drugs into the skin. To overcome this barrier, several proposals have been assessed. Among the approaches used, chemical penetration enhancers have been intensively investigated over the years (Ahad et al. Citation2009, Citation2010).
In recent years, vesicular systems have been intensively studied as drug carrier systems for the dermal and transdermal administration of drugs. Traditional liposomal formulations, compared to conventional dosage forms, have shown in vitro an enhanced cutaneous drug accumulation allowing a reduction of the dose applied onto the skin (Touitou et al. Citation1994). In the last two decades, new classes of lipid vesicles were introduced by different researchers. Cevc and Blume (Citation1992) introduced the first generation of the highly deformable, elastic liposomes, referred as Transfersomes®. They consist of phospholipids and a surfactant molecule, the so called “edge activator”, which destabilizes lipid bilayer and increases its deformability. Subsequently, Touitou et al. (Citation2000) developed ethosomes, new soft vesicular carriers mainly consisting of phospholipids, ethanol, and water. More recently, researchers investigated the novel vesicular systems called as invasomes (Dragicevic-Curic et al. Citation2008b, Citation2009, Verma et al. Citation2003a). Briefly, invasomes contain not only phospholipids but also ethanol and terpenes, which make the vesicles deformable, and also serve as penetration enhancers. This system has shown to improve skin penetration of hydrophilic and lipophilic drugs (Shah et al. Citation2015). Ethanol is a good penetration enhancer while terpenes have also shown potential to increase the penetration of many drugs by disrupting the tight lipid packing of the stratum corneum (Aqil et al. Citation2007, Sapra et al. Citation2008).
The aim of the present study was to develop and characterize isradipine-loaded invasomal drug carrier systems. Isradipine has been previously identified as a promising candidate for transdermal drug delivery (Al-Suwayeh Citation2003, Tirunagari et al. Citation2010). Isradipine () has low oral bioavailability of about 15–24% (Tirunagari et al. Citation2010). It has low molecular weight (371.4 Da) and melting point (168–170 °C) with a log partition coefficient (4.28) and terminal elimination half-life is often stated to be about 8 h although a value of less than 4 h has also been reported (Ahad et al. Citation2013a). All the above characteristics make isradipine a good candidate for transdermal delivery.
Materials and methods
Materials
Isradipine was procured from Novartis, Basel, Switzerland. Phospholipon® 90G was received as a gratis sample from Lipoid AG, Sennweidstrasse, CH-6312 Steinhausen, Switzerland. β-Citronellene was purchased from Sigma-Aldrich Chemicals Private Ltd., New Delhi, India. Ethanol was purchased from Changshu Yangquan Chemical, Suzhou, China. High-Performance Liquid Chromatography (HPLC) grade acetonitrile was purchased from BDH, Poole, England. Carbopol® 934, triethanolamine, and propylene glycol were purchased from S. D. Fine Chemical, Mumbai, India, and Loba Chemie Pvt. Ltd., Mumbai, India, respectively. Double-distilled water was used for all experiments.
Animals
Albino Wistar rats (180–200 g) were supplied by Central Animal House of Hamdard University and inhabited under standard laboratory conditions in 12 h light/dark cycle at 25 ± 2 °C. Animals were nourished with pellet diet (Lipton, India) and water ad libitum. The animals were received after the study was duly approved by the University Animal Ethics Committee and Committee for the Purpose of Control and Supervision on Experiments on Animals (CPSCEA), Government of India. Hairs on the skin of animal were removed with electrical clipper; subcutaneous tissues were surgically removed, and dermis side was wiped with isopropyl alcohol to remove residual adhering fat. The skin was washed with phosphate buffer saline, wrapped in aluminum foil and stored in a deep freezer at −20 °C until further use (used within two weeks of preparation). On the day of experiment, skin was brought to room temperature and skin samples were mounted over the diffusion cells in such a way that stratum corneum side faced the donor compartment whereas the dermis faced the receiver compartment.
Preparation of invasomes
Isradipine invasomes formulations were prepared by conventional thin layer evaporation technique (Shah et al. Citation2015). Briefly, Phospholipon® 90G, isradipine and terpene were taken in a clean, dry, round bottom flask, and dissolved in chloroform, methanol, 2:1 (v/v). The organic solvent was removed by rotary evaporation (Rotary Evaporator, Model-HS-2005V-N, Hahnshin Scientific Co., Bucheon-si, Korea). Final traces of solvent were removed under vacuum overnight. The deposited lipid film was hydrated with ethanolic–phosphate buffer saline (pH 7.4) mixture by rotation at 60 rpm for 1 h at room temperature. The resulting vesicles were swollen for 2 h at room temperature to get large multilamellar vesicles. To prepare smaller particles, large particles were probe sonicated at 4 °C at 40% output frequency (at 40 W) (Titanium probe, Ultrasonicator, Model-UP100H, Hielscher Ultrasonics GmbH, Berlin, Germany). The compositions of various formulations and characterization parameters such as particle size, entrapment efficiency, and transdermal flux are presented in .
Table 1. Formulation details and characterization of isradipine-loaded invasomes.
Particle size and shape determination
The mean invasomes particles size and polydispersity index were analyzed by dynamic light scattering technique using Malvern Zetasizer (Zetasizer, HAS 3000; Malvern Instruments, Malvern, UK). The sample was placed in quartz cuvette, diluted with distilled water and size measurements were carried out at 25 ± 1 °C and at a scattering angle of 90°. All observations were recorded in triplicate for each formulation. The particle shape was visualized by using a Philips, CM10 (Philips Research, Hamburg, Germany) transmission electron microscope. Samples were dried on carbon-coated grid and negatively stained with aqueous solution of phosphotungstic acid. After drying the specimen was viewed under the microscope at 10–100 k-fold enlargements at an accelerating voltage of 100 kV (Ahad et al. Citation2012, Citation2013b).
Determination of entrapment efficiency
Entrapment efficiency of isradipine-loaded invasomes particles was determined by sephadex minicolumn centrifugation method (Chourasia et al. Citation2011, El Maghraby et al. Citation2001). In brief, sephadex G-50 (10% w/v) was placed in a beaker and allowed to swell overnight. To prepare the minicolumn, Whatman filter pad was inserted in the 1 ml syringe and the swollen gel was poured into it. To avoid air entrapment this assembly was centrifuged at 1000 rpm for 5 min and the excessive amount of water was removed. Invasomal suspension 200 μl separately was then poured on to the prepared column and centrifuged at 5000 rpm for 5 min. The same procedure was repeated by adding 100 μl of water to obtain all the vesicles containing entrapped drug. The eluted invasomal vesicles were lysed by using Triton X-100 (0.5% v/v) and filtered through a 0.45 μm nylon disk filter. The concentration of isradipine was analyzed by UV–Visible spectrophotometry at 330 nm. The determination of entrapment efficiency was done in triplicate, all maintained at 25 °C using the following equation.
where CP: concentration of isradipine analyzed after lysing with Triton X-100 as described in the above methods; Ci: the initial concentration of isradipine loaded into the formulation.
In vitro skin permeation study
The in vitro skin permeation of invasomes system was studied using locally fabricated Franz’s diffusion cell having an effective permeation area and receiver cell volume of 2 cm2 and 15 ml, respectively. The receptor cell contained 15 ml of phosphate buffer saline pH 7.4 and ethanol (ratio 70:30), which was constantly stirred with a magnetic stirrer at 100 rpm. Experiments were carried out for 24 h at 32 °C ± 1 °C. Samples were withdrawn through the receiver cell sampling port at 0.5, 1.0, 2.0, 4.0, 8.0, 12.0, and 24.0 h and analyzed for drug content by UV spectrophotometer at 330 nm. The receptor cell after each withdrawal was replenished with an equal volume of fresh vehicle (Ahad et al. Citation2014a, Citation2014b).
Confocal laser scanning microscopy
In order to determine the extent of penetration confocal laser scanning microscopy was performed on the intact skin. Rats were sacrificed and the abdominal skin was removed. Invasomal formulation loaded with Rhodamine B dye was applied to the excised abdominal skin for 18 h. The skin was washed with distilled water and placed on the slide with stratum corneum facing upward and observed under confocal microscope (Olympus Fluoview TM-FV1000, Olympus, Melville, NY) with an argon laser beam with excitation at 488 nm and emission at 590 nm. The skin sample was sliced in sections of 6–10 μm thickness through the z-axis by laser confocal microscope.
Pharmacodynamic study
The rats (180–200 g) were randomly divided into two groups. Hypertension was induced in both the groups (Group A and B) by allowing them to drink 1% NaCl in drinking water and by injecting deoxycorticosterone acetate (DOCA, 5 mg/kg in corn oil) subcutaneously at every fourth day for two weeks (Detroja et al. Citation2011, Majima et al. Citation1991). After two weeks, Group B was subjected to isradipine-loaded invasomal transgel formulation. Formulation was applied to the previously shaven abdominal area of rat skin. The rat was then placed in the restrainer and the systolic blood pressure was recorded using noninvasive blood pressure system (NIBP 200A; Biopac System, Inc., Goleta, CA) based on cuff tail technique at 0, 1, 2, 4, 8, 12, 24 h time intervals.
Results and discussion
Preparation of invasomes
As a new type of vesicle structured drug carrier, invasomes showed the characteristics of good flexibility, high entrapment efficiency, satisfactory permeability, and reliable stability, which enabled the delivery of drugs into deeper skin layers and/or the systemic circulation. In the present work, invasomes containing phospholipid (1, 2, and 3% w/v), 0.1 and 0.5% w/v of the terpene (β-citronellene) and 10% w/v ethanol were prepared by thin film hydration technique in order to accentuate the transdermal penetration of isradipine. Invasomal system was found to be easy to prepare and composed mainly of phospholipids, ethanol, and terpene compounds commonly found in pharmaceutical preparations.
Particle size and shape
Particle size analysis of the optimized formulation revealed a mean particle size of 194 ± 18 nm () which is primarily suitable for transdermal delivery. The observed polydispersity index of the optimized formulation was found to be 0.272 ± 0.062 which also supported the presence of homogeneity and narrow distribution of particle size. Furthermore, obtained by transmission electron micrograph revealed the presence of spherical shape and smooth and even surface of the isradipine-loaded invasomes formulation. The amount of terpene used in formulations has an effect on the particle size as can be seen in formulations F1 and F3 bearing 0.1% β-citronellene have smaller particle size as compared to formulations F2 and F4, having 0.5% terpene (Verma et al. Citation2003b). While this was not the case with formulations F4 and F5; which might be due to the highest phospholipid content in these formulations. Moreover the particles size depicted by duo formulations is not significantly different.
Entrapment efficiency
Entrapment efficiency is a direct commentary on the ability of drug to integrate with lipoidal content to form particles of suitable integrity. The entrapment efficiency for all formulations (F1–F6) isradipine-loaded invasomes systems was more than 80% (). The entrapment efficiency was least found with 80.2% for the formulation F6 and the formulation F3 with a moderate level of phospholipid content (2.0%), and least concentration of terpene (0.1%) showed maximum entrapment (88.46%) as compared to rest of the formulations. In addition to high entrapment efficiency; F3 also elicited a least particle size of 194 nm with a low polydispersity index of 0.272, indicating homogeneous populations of particles.
In vitro skin permeation studies
The in vitro skin permeation profile shows that invasomes formulation (F3) presents maximum transdermal flux value, i.e. 22.80 ± 2.10 μg/cm2/h through rat skin. The reason for this better performance of invasomes is that it contains not only phospholipids but also ethanol and terpenes. Terpenes are considered as potent penetration enhancers, and they have been shown to enhance the percutaneous absorption of hydrophilic and lipophilic drugs (Ahad et al. Citation2011a, Citation2011b, Aqil et al. Citation2007). The presence of ethanol and terpenes makes the particles deformable and may also serve as penetration enhancers. The permeation enhancing effect of invasomes has been explained by fluidizing the stratum corneum lipid bilayer structure, thus disturbing the organization of stratum corneum lipids. These phenomena, i.e. an increased deformability of particles and a disorganized stratum corneum bilayer structure, are thought to facilitate the penetration of invasomes. Thus, invasomes may follow the transepidermal osmotic gradient, into and through the stratum corneum of the skin (Dragicevic-Curic et al. Citation2008a).
The effect of variables such as phospholipid, ethanol and terpene on transdermal flux is shown in . However, to the contrary of our expectation, the transdermal flux value of isradipine delivered by invasomes with 0.5% terpene was smaller, but not significantly, compared to the flux delivered by invasomes with 0.1% terpene. The optimized formulation was selected based on the criteria of attaining the maximum value of transdermal flux and entrapment efficiency; minimizing the particle size. The formulation F3 was found to fulfill requisites of an optimized formulation.
Confocal laser scanning microscopy
To visualize the permeation modes of the invasomes through the stratum corneum, invasomes were further investigated by using confocal laser scanning microscopy. shows the penetration of Rhodamine B-loaded invasomes at different depth levels through the skin. As observed by the confocal laser scanning microscopy, the Rhodamine B-loaded invasomes formulation (F3) traversed the skin thickness to an extent of approximately 171.18 μm with high fluorescence intensity (. This prominently efficient delivery of isradipine-loaded invasomes particles proposes their increased penetration and subsequent fusion with the lipid membrane in the depths of the skin confirming the hypothesis of researchers (Ahad et al. Citation2012, Citation2013b, Godin and Touitou Citation2004, Jain et al. Citation2008, Touitou et al. Citation2000). Overall, invasomes could enhance the delivery of isradipine in terms of depth and quantity.
Pharmacodynamic study
The optimized invasomes formulation (F3) was selected for further pharmacodynamic study. For ease of application and longer contact time; the F3 formulation was converted into gel. Concisely, carbopol 934 (2% w/v) was soaked in minimum amount of water for an hour for complete humectation of polymer chains (Das et al. Citation2013). The formulation F3 was added slowly to the swollen polymer under stirring (Ahad et al. Citation2014a). Additional constituents like polyethylene glycol 400 as plasticizer and triethanolamine (0.1% (w/v)) were added to get uniform gel formulation and named as isradipine-loaded invasomal transgel. The prepared gel formulation presented good homogeneity with absence of lumps. The pH value of 6.4 was observed for developed gel formulation. The drug content was found to be 99.80%. During pharmacodynamics study, administration of DOCA raised the blood pressure to 144.18–155.24 mm Hg in normotensive rats. However, the application of the isradipine-loaded invasomal transgel resulted in a steady reduction of blood pressure. It was noticed that the isradipine-loaded invasomal transgel showed a 17.43% decrease in blood pressure at a 4 h time point. discloses that after application of isradipine-loaded invasomal transgel, the blood pressure of DOCA induced hypertensive rats was controlled for up to 24 h. It was observed that at 8–24 h time point the applied invasomal transgel was found to maintain the blood pressure in propinquity of the normal blood pressure value and the effect was continued till 24 h. It was observed that the isradipine invasomal transgel was found productive in retroverting the rat blood pressure to normal values in DOCA induced hypertensive rats. The above results suggest that the prepared isradipine invasomal transgel admits promise for the management of hypertension.
Conclusion
An invasomes system capable of delivering isradipine through rat epidermis was successfully developed. The flexible invasomes stimulated an increase of the percutaneous permeation of isradipine, thus improving the antihypertensive activity in DOCA induced hypertensive rats. In conclusion, the invasomes delivery systems may be a promising carrier for transdermal delivery of isradipine for the management of hypertension.
Disclosure statement
All authors have approved the final manuscript and the authors declare that they have no conflicts of interest to disclose.
References
- Ahad A, Al-Jenoobi FI, Al-Mohizea AM, Akhtar N, Raish M, Aqil M. 2015a. Systemic delivery of beta-blockers via transdermal route for hypertension. Saudi Pharm J. 23:587–602.
- Ahad A, Al-Jenoobi FI, Al-Mohizea AM, Aqil M, Kohli K. 2013a. Transdermal delivery of calcium channel blockers for hypertension. Expert Opin Drug Deliv. 10:1137–1153.
- Ahad A, Al-Mohizea AM, Al-Jenoobi FI, Aqil M. 2016. Transdermal delivery of angiotensin II receptor blockers (ARBs), angiotensin-converting enzyme inhibitors (ACEIs) and others for management of hypertension. Drug Deliv. 23:579–590.
- Ahad A, Al-Saleh AA, Akhtar N, Al-Mohizea AM, Al-Jenoobi FI. 2015b. Transdermal delivery of antidiabetic drugs: formulation and delivery strategies. Drug Discov Today. 20:1217–1227.
- Ahad A, Aqil M, Ali A. 2014a. Investigation of antihypertensive activity of carbopol valsartan transdermal gel containing 1,8-cineole. Int J Biol Macromol. 64:144–149.
- Ahad A, Aqil M, Kohli K, Chaudhary H, Sultana Y, Mujeeb M, Talegaonkar S. 2009. Chemical penetration enhancers: a patent review. Expert Opin Ther Pat. 19:969–988.
- Ahad A, Aqil M, Kohli K, Sultana Y, Mujeeb M. 2013b. Enhanced transdermal delivery of an anti-hypertensive agent via nanoethosomes: statistical optimization, characterization and pharmacokinetic assessment. Int J Pharm. 443:26–38.
- Ahad A, Aqil M, Kohli K, Sultana Y, Mujeeb M, Ali A. 2010. Transdermal drug delivery: the inherent challenges and technological advancements. Asian J Pharm Sci. 5:276–288.
- Ahad A, Aqil M, Kohli K, Sultana Y, Mujeeb M, Ali A. 2011a. Interactions between novel terpenes and main components of rat and human skin: mechanistic view for transdermal delivery of propranolol hydrochloride. Curr Drug Deliv. 8:213–224.
- Ahad A, Aqil M, Kohli K, Sultana Y, Mujeeb M, Ali A. 2011b. Role of novel terpenes in transcutaneous permeation of valsartan: effectiveness and mechanism of action. Drug Dev Ind Pharm. 37:583–596.
- Ahad A, Aqil M, Kohli K, Sultana Y, Mujeeb M, Ali A. 2012. Formulation and optimization of nanotransfersomes using experimental design technique for accentuated transdermal delivery of valsartan. Nanomedicine. 8:237–249.
- Ahad A, Raish M, Al-Mohizea AM, Al-Jenoobi FI, Alam MA. 2014b. Enhanced anti-inflammatory activity of carbopol loaded meloxicam nanoethosomes gel. Int J Biol Macromol. 67:99–104.
- Al-Suwayeh SA. 2003. Transdermal delivery of isradipine through excised rabbit skin: effect of vehicle and drug concentration. Saudi Pharmaceut J. 11:46–51.
- Aqil M, Ahad A, Sultana Y, Ali A. 2007. Status of terpenes as skin penetration enhancers. Drug Discov Today. 12:1061–1067.
- Cevc G, Blume G. 1992. Lipid vesicles penetrate into intact skin owing to the transdermal osmotic gradients and hydration force. Biochim Biophys Acta. 1104:226–232.
- Chourasia MK, Kang L, Chan SY. 2011. Nanosized ethosomes bearing ketoprofen for improved transdermal delivery. Results Pharma Sci. 1:60–67.
- Das B, Nayak AK, Nanda U. 2013. Topical gels of lidocaine HCl using cashew gum and Carbopol 940: preparation and in vitro skin permeation. Int J Biol Macromol. 62:514–517.
- Detroja C, Chavhan S, Sawant K. 2011. Enhanced antihypertensive activity of candesartan cilexetil nanosuspension: formulation, characterization and pharmacodynamic study. Sci Pharm. 79:635–651.
- Dragicevic-Curic N, Grafe S, Albrecht V, Fahr A. 2008a. Topical application of temoporfin-loaded invasomes for photodynamic therapy of subcutaneously implanted tumours in mice: a pilot study. J Photochem Photobiol B. 91:41–50.
- Dragicevic-Curic N, Scheglmann D, Albrecht V, Fahr A. 2008b. Temoporfin-loaded invasomes: development, characterization and in vitro skin penetration studies. J Control Release. 127:59–69.
- Dragicevic-Curic N, Scheglmann D, Albrecht V, Fahr A. 2009. Development of different temoporfin-loaded invasomes-novel nanocarriers of temoporfin: characterization, stability and in vitro skin penetration studies. Colloids Surf B Biointerfaces. 70:198–206.
- El Maghraby GM, Williams AC, Barry BW. 2001. Skin delivery of 5-fluorouracil from ultradeformable and standard liposomes in-vitro. J Pharm Pharmacol. 53:1069–1077.
- Godin B, Touitou E. 2004. Mechanism of bacitracin permeation enhancement through the skin and cellular membranes from an ethosomal carrier. J Control Release. 94:365–379.
- Gungor S, Ozsoy Y. 2012. Systemic delivery of antihypertensive drugs via skin. Ther Deliv. 3:1101–1116.
- Jain SK, Gupta Y, Jain A, Rai K. 2008. Enhanced transdermal delivery of acyclovir sodium via elastic liposomes. Drug Deliv. 15:141–147.
- Majima M, Katori M, Hanazuka M, Mizogami S, Nakano T, Nakao Y, et al. 1991. Suppression of rat deoxycorticosterone-salt hypertension by kallikrein–kinin system. Hypertension. 17:806–813.
- Sapra B, Jain S, Tiwary AK. 2008. Percutaneous permeation enhancement by terpenes: mechanistic view. AAPS J. 10:120–132.
- Selvam RP, Singh AK, Sivakumar T. 2010. Transdermal drug delivery systems for antihypertensive drugs – a review. Int J Pharm Biomed Res. 1:1–8.
- Shah SM, Ashtikar M, Jain AS, Makhija DT, Nikam Y, Gude RP, et al. 2015. LeciPlex, invasomes, and liposomes: a skin penetration study. Int J Pharm. 490:391–403.
- Tirunagari M, Jangala VR, Khagga M, Gannu R. 2010. Transdermal therapeutic system of isradipine: effect of hydrophilic and hydrophobic matrix on in vitro and ex vivo characteristics. Arch Pharm Res. 33:1025–1033.
- Touitou E, Dayan N, Bergelson L, Godin B, Eliaz M. 2000. Ethosomes – novel vesicular carriers for enhanced delivery: characterization and skin penetration properties. J Control Release. 65:403–418.
- Touitou E, Junginger HE, Weiner ND, Nagai T, Mezei M. 1994. Liposomes as carriers for topical and transdermal delivery. J Pharm Sci. 83:1189–1203.
- Verma DD, Verma S, Blume G, Fahr A. 2003a. Liposomes increase skin penetration of entrapped and non-entrapped hydrophilic substances into human skin: a skin penetration and confocal laser scanning microscopy study. Eur J Pharm Biopharm. 55:271–277.
- Verma DD, Verma S, Blume G, Fahr A. 2003b. Particle size of liposomes influences dermal delivery of substances into skin. Int J Pharm. 258:141–151.