Abstract
Adipose-derived stem cells (ADSCs) derived from adipose tissue have the capacity to differentiate into endodermal, mesoderm, and ectodermal cell lineages in vitro, which are an ideal engraft in tissue-engineered repair. In this study, human ADSCs were isolated from subcutaneous fat. The markers of ADSCs, CD13, CD71, CD73, CD90, CD105, CD166, CYP3A4, and ALB were detected by immunofluorescence assays. Human ADSCs were cultured in a specific hepatogenesis differentiation medium containing HGF, bFGF, nicotinamide, ITS, and oncostatin M for hepatogenic differentiation. The hepatocyte markers were analyzed using immunofluorescence and real-time PCR after dramatic changes in morphology. Hepatocytes derived from ADSCs or ADSCs were transplanted into the mice of liver injury for observation cells colonization and therapy in liver tissue. The result demonstrated that human ADSCs were positive for the CD13, CD71, CD73, CD90, CD105, and CD166 but negative for hepatocyte markers, ALB and CYP3A4. After hepatogenic differentiation, the hepatocytes were positive for liver special markers, gene expression level showed a time-lapse increase with induction time. Human ADSCs or ADSCs-derived hepatocyte injected into the vein could improve liver function repair and functionally rescue the CCl4-treated mice with liver injury, but the ADSCs transplantation was better than ADSCs-derived hepatocyte transplantation. In conclusion, our research shows that a population of hepatocyte can be specifically generated from human ADSCs and that cells may allow for participation in tissue-repair.
Introduction
A number of studies on animal models indicated that transplants consisting of isolated hepatocytes can correct various metabolic deficiencies of liver and reverse liver hepatic failure in current research (Allen et al. Citation2004, Waelzlein et al. Citation2009). However, its applicability remains limited by a number of issues, such as the shortage of hepatocytes, high cost, and relatively poor initial and long-term hepatocyte engraftment in the recipient (Soltys et al. Citation2010). Stem cells have been a focus of regenerative medicine for their multi-potential differentiation ability. It is attractive to develop stem-cell-based therapy or transplanting hepatocytes generated in vitro from stem cells for treatment of liver diseases. Many types of stem cells from different sources have been investigated for hepatic differentiation ability (Banas et al. Citation2007, Chen et al. Citation2012, Yamamoto et al. Citation2003). Embryonic stem cells have enormous differentiation ability, but many limitations including teratoma formation after transplantation, immunogenicity, and ethical issues are arresting their clinical application (Lin et al. Citation2011). Mesenchymal stem cells were elements with multi-differentiation potential isolated from organism, including bone marrow (Bai et al. Citation2015, Herberg et al. Citation2013, Ho et al. Citation2013, Lin et al. Citation2013), adipose tissue (Chung et al. Citation2013, Li Citation2015, Villanueva et al. Citation2013, Zhao et al. Citation2015), derma (Fleming et al. Citation1998, Park et al. Citation2012), liver (Banas et al. Citation2008, Sato et al. Citation2005), lung (Martin et al. Citation2008, Sinclair et al. Citation2013), and other tissues (Bai et al. Citation2015). Adipose tissue has the ability to dynamically expand and shrink throughout the life of an adult. This capacity is mediated by the presence of vascular and non-vascular cells that provide a pool of stem and progenitor cells with regenerative capacity (Banas Citation2012, Strem et al. Citation2005). The multi-differentiation potential of adult stem cells from adipose tissue has been extensively documented (Chung et al. Citation2013, Villanueva et al. Citation2013). The adipose tissue-derived stem cells (ADSCs) are mesenchymal stem cells which have been shown to have hepatogenic capability in vitro and in vivo (Aurich et al. Citation2009, Stock et al. Citation2010, Yin et al. Citation2015) and actions of repair to liver damages (Harn et al. Citation2012, Kim et al. Citation2011). The mechanism of actions was not clearly elucidated but may include their ability to differentiate into hepatocyte-like cells, to reduce inflammation, and to enhance tissue repair at the site of injury. Several lines of evidence indicated that transplanted stem cells adopt the phenotype of hepatocytes and restore liver function by cell fusion rather than differentiation (Vassilopoulos et al. Citation2003, Wang et al. Citation2003), and in vivo studies of stem cells transplantation provide little insight as to which cell populations in the stem cells contribute to hepatic regeneration. In this study, hepatocytes derived from ADSCs and ADSCs were transplanted into mouse acute liver injury model, respectively, to demonstrate and compares the effect of cell therapy between these cells in recovery of liver function.
Materials and methods
Ethics statement
Human Adipose tissues collection for research purposes was approved by the Research Ethics Committees of First People's Hospital of Jingmen. The human samples were obtained from volunteers with informed consent and according to a protocol approved by Research Ethics Committees.
Isolation and culture of human ADSCs
Adipose tissues were separated from abdomen fat of human. The fat tissues were washed three times with PBS containing 104 IU/mL penicillin/streptomycin to remove connective tissue membrane and capillaries. The tissues were chopped into small pieces (about 1mm3), and digested with 0.1% typeIcollagenase (Sigma, St. Louis, MO) at 37 °C for 1 h. Enzymatic digestion was then neutralized with FBS (Gibco, Grand Island, NY). The suspension was filtered with 80 μm cell strainer, and centrifuged at 300 × g for 5 min for collection the ADSCs. The cells were independent cultured in complete medium containing DMEM/F-12 (Gibco), 10% FBS (Gibco), 10 ng/ml bFGF (Peprotech, Rocky Hill, NJ), 2 mM L-glutamine, and 1% B-27 (Gibco) for each mouse. The culture medium was changed every 3 days and the non-adherent cells were removed after 3 days (Yoshimura et al. Citation2007, Zhao et al. Citation2015). When the cells reached 70–80% confluence, added 0.25% trypsin and 0.02% EDTA (Gibco) to dissociate the cells from plates, then terminate trypsinization with complete medium. Cells were subcultured into new plates and incubated at 37 °C with 5% CO2.
Population doubling time
The ADSCs of different passages were plated in 24-well plate at the density of 1 × 104 cell/well, cultured for 7 days and counted every day (3 well each time) afterwards. The mean cell counts at each time point were then used to plot the growth curve, based on which the population doubling time (PDT) was calculated. PDT = (t − t0) log2/(logNt − logN0), t0: starting time of culture; t: termination time of culture; N0: initial cell number of culture; Nt: ultimate cell number of culture.
Hepatogenic differentiation
For hepatogenic differentiation, different passage cells, at 1.0 × 104/cm2, were serum deprived for 2 days, in IMDM supplemented with 20 ng/mL epidermal growth factor and 10 ng/mL bFGF, prior to induction by a two-step protocol. Differentiation was induced by treating MSCs with Step-1 differentiation medium, consisting of IMDM supplemented with 20 ng/mL HGF, 10 ng/mL bFGF, and 0.61 g/L nicotinamide for 7 days, followed by treatment with step-2 maturation medium, consisting of IMDM supplemented with 20 ng/mL oncostatin M, 1 μmol/L dexamethasone, and 50 mg/mL ITS premix, thereafter, medium changes were performed twice weekly and hepatogenesis was assessed by real time PCR for liver-associated genes listed in and by immunofluorescence analysis for cytochrome P450, family 3, subfamily A, polypeptide 4 (CYP3A4), and albumin production (ALB).
Table 1. Primer sequences used in PCR.
Immunofluorescence
Adipose-derived stem cells (ADSCs) or differentiated hepatocyte were washed three times with PBS buffer then fixed with 4% paraformaldehyde for 15 min at room temperature and washed three times with PBS buffer. Cells were permeabilized by incubating with 0.01% Triton X-100 for 10 min. Cells were then washed three times with PBS buffer and incubated with 4% BSA for 30 min. Cells were then incubated with primary antibodies in a humidified chamber at 4 °C overnight. After three washes with PBS, the cells were incubated with FITC-labeled secondary antibodies at room temperature for 1 h, the cells were rinsed three times with PBS for 5 min each. Finally, nuclei were labeled by incubation with 4,6-diamidino-2-phenylindole (DAPI) (Sigma). The cells were examined by a phase contrast fluorescence microscope (Olympus, Tokyo, Japan).
Real time PCR
Real time PCR was used to analyze the special genes expression on different passages ADSCs and different induced-days of hepatocyte. Total RNA was isolated from cells according to the protocol described by Chomczynski and Sacchi (Citation1987). Primers were listed in corresponding to the standards of O'Driscoll et al. (Citation1993) PCR was conducted according to the protocol described by Saiki et al. (Citation1988). Real-time PCR was carried out using a Takara SYBR Green qPCR kit (Takara, China). The PCR mixture, which contained 20 pmol of forward and reverse primers and 2 μl of cDNA, was subjected to amplification with a DNA Engine Opticon 1 (Takara, China). The cycles were set at 95 °C for 10 min for pre-heating, followed by 40 cycles at 94 °C for 15 s, at 55 °C for 30 s, and at 72 °C for 30 s. The amplicons were detected directly by measuring the increase in fluorescence caused by the binding of the SYBR Green I dye to gene-specific and amplified double-strand DNA using a DNA Engine Opticon 1. Following the completion of the PCR reaction, the temperature was raised from the annealing temperature to 95 °C for melting curve analysis. The expression level was calculated by the 2−△△Ct method and compared with the relative expression.
Transplantation of ADSCs or hepatocyte into model of acute liver injury
The mouse model of acute liver injury was made using previously report methods (Manuelpillai et al. Citation2010). Animals received cyclosporin A (10 mg/kg/d) beginning 1 day before transplantation until the end of the study. The mice were injected with a single intraperitoneal dose of CCl4 solution in olive oil (5.0 ml/kg body weight as 1%, vol/vol; Sigma-Aldrich) to induce acute liver injury. Vehicle (olive oil)-injected mice (n = 3) were used as controls. Transplantation surgeries occurred 7 days after liver injury, each experimental mouse received 2–3 × 106 hepatocytes derived from ADSCs or ADSCs in 0.5 ml PBS buffer intravenously. Control animals received equal volume of PBS. Mice were subsequently killed and livers assessed at three weeks after transplantation under anesthesia. After three weeks of transplantation, mice were sacrificed and then perfused with saline and 4% paraformaldehyde (Sigma). A total of 50 cross sections with thickness of 10 μm were made from all liver tissues. Due to special human nuclei protein, we use immunohistochemistry to identify transplanted hepatocytes derived from ADSCs or ADSCs. To distinguish between the injected hepatocytes derived from ADSCs, ADSCs, and control group, DAPI staining was performed as counterstaining and stained cells were observed at a wavelength of 488 nm under a fluorescence microscope.
ALT and AST analysis
Serum was harvested following 2 days, 4 days, 6 days, 8 days, 10 days, 12 days, 14 days, and 3 week of transplantation for the different groups. The ALT and AST content of mouse serum was quantified using a commercially available enzyme-linked immunosorbent assay (ELISA) kit (Alpha Diagnostic Intl, San Antonio, TX) according to the manufacturer’s protocol.
Statistical analysis
Statistical analyses of the data were performed with a one-way ANOVA followed by the Tukey–Kramer honestly significant difference (HSD) test for the three sets of results. A P value of less than 0.05 was considered significant. Statistical analyses were done with a JMP® Statistical Discovery Software (SAS Institute, Cary, NC).
Results
Culture of hADSCs in vitro
The human ADSCs were isolated, cultured and proliferated in vitro from adipose tissue and cell line was established. Human ADSCs were large, lucent, and with strong refraction. The non-adherent cells were removed on the third day and ADSCs were fusiform and showed cell-like clone with growth being slower. Growth and proliferation of human ADSCs was similar in P0, P10, P20, P30, and P50 according to the growth curves (data not shown). After the latency phase of 1–3 days, cell growth entered the logarithmic phase, and reached plateau phase at about day 7. The population doubling time (PDT) was showed in and there are no significant changes in PDT of different passages. These cells were maintained in culture for more than 100 days with no sign of senescence or differentiation (. The special genes of ADSCs, CD13, CD71, CD73, CD90, CD105, CD166, ALB, and CYP3A4 were detected by immunofluorescence assays. Immunofluorescence staining and real time PCR results showed that different passages of human ADSCs expressed CD13, CD71, CD73, CD90, CD105, and CD166, but did not express ALB and CYP3A4. ALB and CYP3A4 is special marker for hepatocyte (.
Figure 1. Characteristics of human ADSCs in vitro. (A) Proliferation potential of human ADSCs under the cultural conditions in vitro. (B) Population doubling time (PDT) of human ADSCs, the result showed that PDT was no significant change among seven consecutive culture passages.
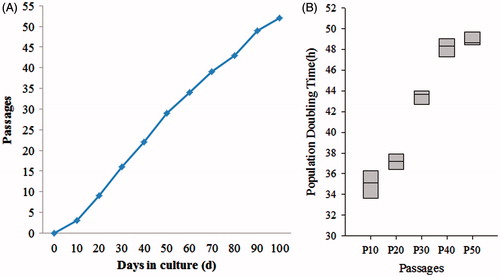
Figure 2. Special gene characteristics of human ADSCs. Immunoflourescence staining results showed that human ADSCs were positive for the CD13, CD71, CD73, CD90, CD105, and CD166, but negative for liver-associated genes, ALB and CYP3A4 (Scan bar = 50 μm).
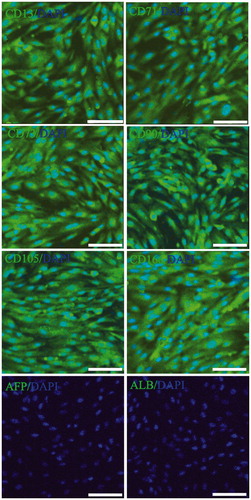
Figure 3. Special gene expression in different passages human ADSCs. CD13, CD71, CD73, CD90, CD105, and CD166, the special genes of ADSCs were analyzed using real time PCR in P0, P10, P20, P30, and P50, the result showed that these genes ware no significant change among seven consecutive culture passages.
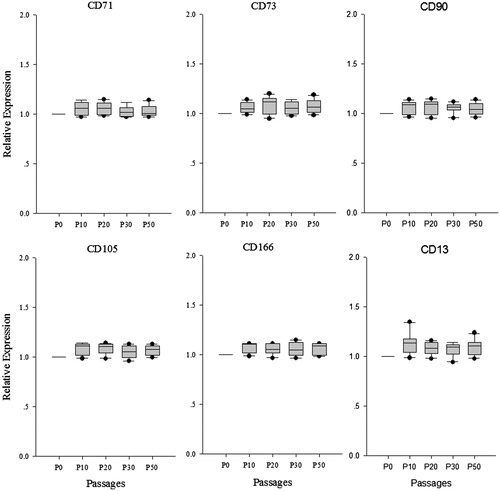
In vitro hepatogenic differentiation of human ADSCs
To examine the hepatogenic differentiation ability in vitro, human ADSCs were cultured in a specific hepatogenesis differentiation medium containing HGF, bFGF, nicotinamide, ITS, and oncostatin M. Cell morphology was monitored every 2 days using a phase-contrast microscope. During the differentiation procedure, cells gradually changed from spindle, bipolar, fibroblast like cells to round or polygonal epithelioid cells ( (phase)). On Day 19, almost all induced cells showed epithelioid and cuboidal shapes, similar to primary human hepatocytes. Cells cultured in a medium without conditioned factors were set as control and showed no significant changes in morphology. At day 20, the cells were detected ALB and CYP3A4 using immunofluorescence, the results showed ALB and CYP3A4 double positive in differentiated hepatocyte (). Undifferentiated cells showed no hepatocyte-specific gene expression except for ALB and CYP3A4. In addition, gene expression of liver-associated genes showed a time-dependent decrease following differentiation of hepatocyte. Gene expressions in each time point were determined by Real-time PCR and then normalized to that of control (0 day), the result showed in . CYP3A4 mRNA levels increased in differentiated hepatocyte at day 20 of culture (. At this stage, CYP3A4 protein was detected in differentiated hepatocyte (. ALB mRNA levels were higher (P < 0.05) in differentiated hepatocyte compared to untreated control at day 20 of culture (. At day 20, TAT mRNA levels and CNX32 mRNA levels were significantly increased (P < 0.05) in differentiated hepatocyte compared to untreated control (.
Figure 4. Identification surface markers of hepatocyte using immunoflourescence staining. Hepatocytes are the chief functional cells of the liver and perform an astonishing number of metabolic, endocrine, and secretory functions. ALB functions primarily as a carrier protein for steroids, fatty acids, and thyroid hormones and plays a role in stabilizing extracellular fluid volume. CYP3A4 is an important enzyme in the body, mainly found in the liver and in the intestine, which is a member of the cytochrome P450 superfamily of enzymes. The cytochrome P450 proteins are monooxygenases that catalyze many reactions involved in drug metabolism and synthesis of cholesterol, steroids, and other lipids components. Immunoflourescence staining result showed that ALB and CYP3A4 ware double positive in hepatocytes derived from ADSCs.
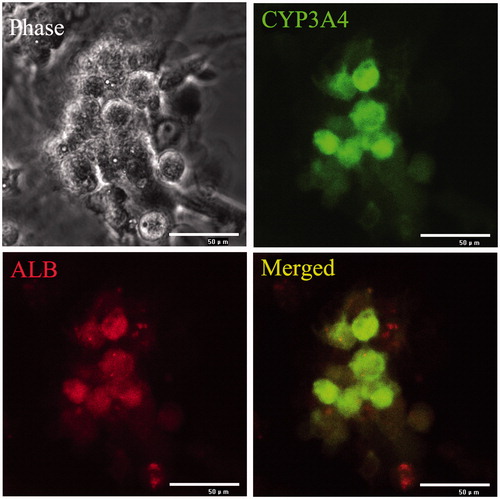
Figure 5. Gene expression of liver-associated genes detected in continuously cultured human ADSCs. Gene expressions in each time point were determined by real-time PCR and then normalized to that of control (0 day). The result showed relative expression level liver-associated genes mRNA were higher in differentiated hepatocyte compared to untreated control from day 14 of culture. At day 20, these genes mRNA levels were significantly increased (P < 0.05) in differentiated hepatocyte compared to control.
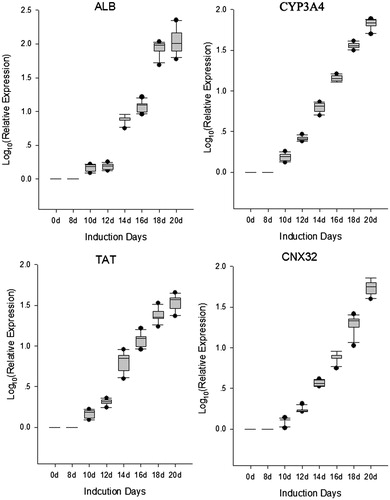
Transplantation of human hepatocyte or ADSCs into model of acute liver injury
Pathological change was observed in mice liver after CCl4 treatment, cytoplasm vacuolization, karyopyknosis and apoptosis were appeared in hepatocyte around central veins (). hADSCs-derived hepatocyte and hADSCs were used to transplant via intravenous injection (I.V.) respectively and take part in tissue regeneration in acute liver injury. To test whether transplantation of hepatocyte and hADSCs taken part in recovery of hepatic lobule, paraffin section was used to observed the location for transplantation of hepatocyte or ADSCs in scathing liver tissue. Human nuclei protein was used as a label to discover the human hepatocyte or ADSCs in tissue of hepatic lobule. The transplanted hepatocyte and hADSCs were counted from 11 and 23 serial sections slide out of a total of 50 sections, respectively. The results demonstrated that human hepatocyte and ADSCs could take part in recovery of acute liver injury, but the treatment effect of ADSCs group was significantly higher than human hepatocyte group in serological test. ALT and AST were significantly increase after acute liver injury through serological testing by ELISA, the concentrate of ALT and AST were significantly decrease after injected ADSCs 8 days, but the hepatocyte group were observed changes after 12 days (). Three weeks later, the concentrate of ALT and AST of ADSCs-treated mice reduced to a normal level (<50 U/L). In contrast, the ALT and AST concentrate of the injected hepatocyte mice was still above 100 U/L, and these mice could survive for nearly 4 weeks. These results showed that the ADSCs or ADSCs-derived hepatocyte, when injected into the vein, could improve liver function repair and functionally rescue the CCL4-treated mice with liver injury, but the ADSCs transplantation was better than ADSCs-derived hepatocyte transplantation.
Figure 6. Transplantation of hADSCs, hADSCs-derived hepatocyte resulted in mouse acute liver injury. To test whether transplantation of hepatocyte and hADSCs taken part in recovery of hepatic lobule, paraffin section was used to observed the location for transplantation of hepatocyte in scathing liver tissue. Human nuclei protein was used as a label to discover the hADSCs or hADSCs-derived hepatocyte in tissue of hepatic lobule. The results showed that the human cells were found in mouse liver tissue through IV, but the group of hADSCs was significantly higher than group of hADSCs-derived hepatocyte. (A) Hematoxylin and eosin staining of paraffin section in acute liver injury. (B, C, and D, long arrow: central veins, short arrow: apoptotic hepatocytes) Representative immunofluorescence images of liver 3 weeks post-transplantation, stained for human nuclei protein to confirm the presence of hADSCs. Arrow: central veins. (bar = 100 μm).
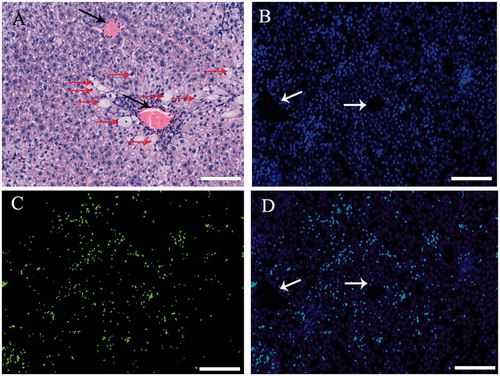
Figure 7. AST and ALT detection after injury and transplantation. ALT and AST were significantly increase after acute liver injury through serological testing by ELISA, the concentrate of ALT and AST were significantly decrease after injected ADSCs 8 days, but the hepatocyte group were observed changes after 12 days. Three weeks later, the concentrate of ALT and AST of ADSCs-treated mice reduced to a normal level (<50 U/L). In contrast, the ALT and AST concentrate of the injected hepatocyte mice was still above 100 U/L, and these mice could survive for nearly 4 weeks. These results showed that the ADSCs or ADSCs-derived hepatocyte, when injected into the vein, could improve liver function repair and functionally rescue the CCL4-treated mice with liver injury, but the ADSCs transplantation was better than ADSCs-derived hepatocyte transplantation.
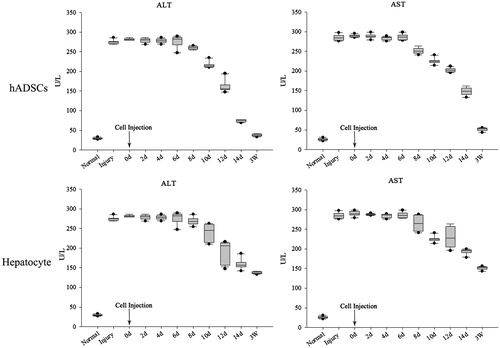
Discussion
Cell therapy has emerged as a strategy for the treatment of many diseases. At present, tissue stem cells were tentatively expanded and orientationally induced in vitro to some seed cells that are needed, which are then transplanted into patients to repair damage, to replace regressive tissue and improve the function of hereditarily defective tissue. The transplantation of hematopoietic stem cell has been widely used in the treatment of hematopoietic malignent diseases, which provides an exemplification for the study of tissue stem cells. Recent studies challenge the view that tissues are maintained solely by organ-specific stem cells. There is evidence that adult stem cells from a variety of sources can generate not only their own lineages, but those of other tissues, sometimes crossing barriers of embryonic derivation previously thought impenetrable (Korbling and Estrov Citation2003, Neuringer and Randell Citation2004). MSCs have several properties that make them attractive as a potential treatment for liver injury. MSCs avoid allo-recognition, home to sites of injury and suppress inflammation as well as immune responses (Curley et al. Citation2012, Nemeth et al. Citation2009). In this study, we have shown that, by manipulation of the culture conditions, hADSCs can be encouraged to differentiate into hepatocyte in vitro, and then ADSCs and hADSCs-derived hepatocyte were injected into acute liver injury mice respectively via I.V. to demonstrate capacity of cells repair. The results showed that stem cells were better than differentiated thesocyte due to stem cell homing. Stem cell homing was firstly found in hematopoietic stem cells, transplantation of hematopoietic stem cells is dependent upon the successful homing, engraftment and repopulation of stem cells in the bone marrow. Stem cell homing through the circulation to the bone marrow is the critical step in this process. Stem cell homing is the phenomenon whereby cells migrate to the organ of their origin. By homing, transplanted stem cells are able to travel to and engraft or establish residence in the injured organ. Chemokines and their receptors are now recognized as important mediators of stem cell homing.
Carbon tetrachloride (CCl4) has been widely used to experimentally induce liver injury in rodents, a single dose of CCl4 leads to centrizonal necrosis and steatosis (Pierce et al. Citation1987), while prolonged administration leads to liver fibrosis, cirrhosis, and Hepatocellular carcinoma (HCC). CCl4 impairs hepatocytes directly by altering the permeability of the plasma, lysosomal, and mitochondrial membranes. Hepatocytes will release a lot of inflammatory factors to blood circulation after CCl4-injury, which is important signal for stem cell homing. It is not known which physiological or pathological factors influence the homing and little is known about the signals that direct circulating stem cells to sites of injured tissue. For this reason, the ADSCs transplantation was better than ADSCs-derived hepatocyte transplantation after liver CCl4-injury. In addition, additional experimental, clinical, and cell biological studies are needed to increase the understanding of the function of stem cells and of the factors that determine their number and turnover rate as well as the mechanisms that stimulate or inhibit their mobilization, differentiation, and homing in vitro and in vivo. Such investigations are required to explore areas of future basic and clinical research, particularly because the first clinical trials using stem cells have just been started.
In summary, the results of our research demonstrated that hADSCs have the capacity to generate hepatocytes in vitro and in vivo. In light of their potential to repair the injured liver function, hADSCs could theoretically and virtually provide an unlimited supply of cells for transplantation. Their great promise provides hope that hepatic lobule derivatives of hADSCs could be grafted to reconstitute the hepatic tissue in a variety of liver diseases.
Disclosure statement
The authors report no conflicts of interest. The authors alone are responsible for the content and writing of this article.
References
- Allen KJ, Cheah DM, Wright PF, Gazeas S, Pettigrew-Buck NE, Deal YH, Mercer JF, Williamson R. 2004. Liver cell transplantation leads to repopulation and functional correction in a mouse model of Wilson's disease. J Gastroenterol Hepatol. 19:1283–1290.
- Aurich H, Sgodda M, Kaltwasser P, Vetter M, Weise A, Liehr T, et al. 2009. Hepatocyte differentiation of mesenchymal stem cells from human adipose tissue in vitro promotes hepatic integration in vivo. Gut. 58:570–581.
- Bai C, Gao Y, Li Q, Feng Y, Yu Y, Meng G, Zhang M, Guan W. 2015. Differentiation of chicken umbilical cord mesenchymal stem cells into beta-like pancreatic islet cells. Artif Cells Nanomed Biotechnol. 43:106–111.
- Banas A. 2012. Purification of adipose tissue mesenchymal stem cells and differentiation toward hepatic-like cells. Methods Mol Biol. 826:61–72.
- Banas A, Teratani T, Yamamoto Y, Tokuhara M, Takeshita F, Quinn G, Okochi H, Ochiya T. 2007. Adipose tissue-derived mesenchymal stem cells as a source of human hepatocytes. Hepatology. 46:219–228.
- Banas A, Teratani T, Yamamoto Y, Tokuhara M, Takeshita F, Osaki M, et al. 2008. IFATS collection: in vivo therapeutic potential of human adipose tissue mesenchymal stem cells after transplantation into mice with liver injury. Stem Cells. 26:2705–2712.
- Chen X, Zhang S, Liu T, Liu Y, Wang Y. 2012. Maintenance of rat hepatocytes under inflammation by coculture with human orbital fat-derived stem cells. Cell Mol Biol Lett. 17:182–195.
- Chomczynski P, Sacchi N. 1987. Single-step method of RNA isolation by acid guanidinium thiocyanate-phenol-chloroform extraction. Anal Biochem. 162:156–159.
- Chung BH, Lim SW, Doh KC, Piao SG, Heo SB, Yang CW. 2013. Human adipose tissue derived mesenchymal stem cells aggravate chronic cyclosporin nephrotoxicity by the induction of oxidative stress. PLoS One. 8:e59693.
- Curley GF, Hayes M, Ansari B, Shaw G, Ryan A, Barry F, et al. 2012. Mesenchymal stem cells enhance recovery and repair following ventilator-induced lung injury in the rat. Thorax. 67:496–501.
- Fleming JE Jr, Haynesworth SE, Cassiede P, Baber MA, Caplan AI. 1998. Monoclonal antibody against adult marrow-derived mesenchymal stem cells recognizes developing vasculature in embryonic human skin. Dev Dyn. 212:119–132.
- Harn HJ, Lin SZ, Hung SH, Subeq YM, Li YS, Syu WS, et al. 2012. Adipose-derived stem cells can abrogate chemical-induced liver fibrosis and facilitate recovery of liver function. Cell Transplant. 21:2753–2764.
- Herberg S, Fulzele S, Yang N, Shi X, Hess M, Periyasamy-Thandavan S, et al. 2013. Stromal cell-derived factor-1beta potentiates bone morphogenetic protein-2-stimulated osteoinduction of genetically engineered bone marrow-derived mesenchymal stem cells in vitro. Tissue Eng Part a. 19:1–13.
- Ho IA, Toh HC, Ng WH, Teo YL, Guo CM, Hui KM, Lam PY. 2013. Human bone marrow-derived mesenchymal stem cells suppress human glioma growth through inhibition of angiogenesis. Stem Cells. 31:146–155.
- Kim SJ, Park KC, Lee JU, Kim KJ, Kim DG. 2011. Therapeutic potential of adipose tissue-derived stem cells for liver failure according to the transplantation routes. J Korean Surg Soc. 81:176–186.
- Korbling M, Estrov Z. 2003. Adult stem cells for tissue repair – a new therapeutic concept? N Engl J Med. 349:570–582.
- Li JD. 2015. Directed differentiation of airway epithelial cells of human bone marrow mesenchymal stem cells. Artif Cells Nanomed Biotechnol. [Epub ahead of print]. doi: 10.3109/21691401.2015.1070858.
- Lin BN, Whu SW, Chen CH, Hsu FY, Chen JC, Liu HW, Liou HM. 2013. Bone marrow mesenchymal stem cells, platelet-rich plasma and nanohydroxyapatite-type I collagen beads were integral parts of biomimetic bone substitutes for bone regeneration. J Tissue Eng Regen Med. 7:841–854.
- Lin J, Xiang D, Zhang JL, Allickson J, Xiang C. 2011. Plasticity of human menstrual blood stem cells derived from the endometrium. J Zhejiang Univ Sci B. 12:372–380.
- Manuelpillai U, Tchongue J, Lourensz D, Vaghjiani V, Samuel CS, Liu A, Williams ED, Sievert W. 2010. Transplantation of human amnion epithelial cells reduces hepatic fibrosis in immunocompetent CCl4-treated mice. CCl(4)-treated mice. Cell Transplant. 19:1157–1168.
- Martin J, Helm K, Ruegg P, Varella-Garcia M, Burnham E, Majka S. 2008. Adult lung side population cells have mesenchymal stem cell potential. Cytotherapy. 10:140–151.
- Nemeth K, Leelahavanichkul A, Yuen PS, Mayer B, Parmelee A, Doi K, et al. 2009. Bone marrow stromal cells attenuate sepsis via prostaglandin E(2)-dependent reprogramming of host macrophages to increase their interleukin-10 production. Nat Med. 15:42–49.
- Neuringer IP, Randell SH. 2004. Stem cells and repair of lung injuries. Respir Res. 5:6. doi: 10.1186/1465-9921-5-6.
- O'Driscoll L, Daly C, Saleh M, Clynes M. 1993. The use of reverse transcriptase-polymerase chain reaction (RT-PCR) to investigate specific gene expression in multidrug-resistant cells. Cytotechnology. 12:289–314.
- Park BW, Kang EJ, Byun JH, Son MG, Kim HJ, Hah YS, et al. 2012. In vitro and in vivo osteogenesis of human mesenchymal stem cells derived from skin, bone marrow and dental follicle tissues. Differentiation. 83:249–259.
- Pierce RA, Glaug MR, Greco RS, Mackenzie JW, Boyd CD, Deak SB. 1987. Increased procollagen mRNA levels in carbon tetrachloride-induced liver fibrosis in rats. J Biol Chem. 262:1652–1658.
- Saiki RK, Gelfand DH, Stoffel S, Scharf SJ, Higuchi R, Horn GT, Mullis KB, Erlich HA. 1988. Primer-directed enzymatic amplification of DNA with a thermostable DNA polymerase. Science. 239:487–491.
- Sato Y, Araki H, Kato J, Nakamura K, Kawano Y, Kobune M, et al. 2005. Human mesenchymal stem cells xenografted directly to rat liver are differentiated into human hepatocytes without fusion. Blood. 106:756–763.
- Sinclair K, Yerkovich ST, Chambers DC. 2013. Mesenchymal stem cells and the lung. Respirology. 18:397–411.
- Soltys KA, Soto-Gutierrez A, Nagaya M, Baskin KM, Deutsch M, Ito R, et al. 2010. Barriers to the successful treatment of liver disease by hepatocyte transplantation. J Hepatol. 53:769–774.
- Stock P, Bruckner S, Ebensing S, Hempel M, Dollinger MM, Christ B. 2010. The generation of hepatocytes from mesenchymal stem cells and engraftment into murine liver. Nat Protoc. 5:617–627.
- Strem BM, Hicok KC, Zhu M, Wulur I, Alfonso Z, Schreiber RE, Fraser JK, Hedrick MH. 2005. Multipotential differentiation of adipose tissue-derived stem cells. Keio J Med. 54:132–141.
- Vassilopoulos G, Wang PR, Russell DW. 2003. Transplanted bone marrow regenerates liver by cell fusion. Nature. 422:901–904.
- Villanueva S, Carreno JE, Salazar L, Vergara C, Strodthoff R, Fajre F, et al. 2013. Human mesenchymal stem cells derived from adipose tissue reduce functional and tissue damage in a rat model of chronic renal failure. Clin Sci (Lond). 125:199–210.
- Waelzlein JH, Puppi J, Dhawan A. 2009. Hepatocyte transplantation for correction of inborn errors of metabolism. Curr Opin Nephrol Hypertens. 18:481–488.
- Wang X, Willenbring H, Akkari Y, Torimaru Y, Foster M, Al-Dhalimy M, et al. 2003. Cell fusion is the principal source of bone-marrow-derived hepatocytes. Nature. 422:897–901.
- Yamamoto H, Quinn G, Asari A, Yamanokuchi H, Teratani T, Terada M, Ochiya T. 2003. Differentiation of embryonic stem cells into hepatocytes: biological functions and therapeutic application. Hepatology. 37:983–993.
- Yin L, Zhu Y, Yang J, Ni Y, Zhou Z, Chen Y, Wen L. 2015. Adipose tissue-derived mesenchymal stem cells differentiated into hepatocyte-like cells in vivo and in vitro. Mol Med Rep. 11:1722–1732.
- Yoshimura H, Muneta T, Nimura A, Yokoyama A, Koga H, Sekiya I. 2007. Comparison of rat mesenchymal stem cells derived from bone marrow, synovium, periosteum, adipose tissue, and muscle. Cell Tissue Res. 327:449–462.
- Zhao XL, Yang B, Ma LN, Dong YH. 2015. MicroRNA-1 effectively induces differentiation of myocardial cells from mouse bone marrow mesenchymal stem cells. Artif Cells Nanomed Biotechnol. [Epub ahead of print]. doi: 10.3109/21691401.2015.1080168.
- Zhao Y, Jiang H, Liu XW, Chen JT, Xiang LB, Zhou DP. 2015. Neurogenic differentiation from adipose-derived stem cells and application for autologous transplantation in spinal cord injury. Cell Tissue Bank. 16:335–342.