Abstract
Our main investigation in the present research was to developt and evaluate targeting ligand-anchored multiwalled carbon nanotubes (MWCNTs) as prospective targeted drug delivery system, with a special focus on the MWCNTs surface functionalization (FA-PEG bis-amine functionalized, carboxylated MWCNTs). In vitro release of 5-fluorouracil (5-FU) was studied at pH 7.4 phosphate buffer and 5.5 acetate buffer, which displayed initial faster followed by sustained release up to 900 min. Further, 5-FU/FA-PEG bis amine-MWCNTs was found to be long circulating, prolonged half-life and increased drug accumulation in target tissue.
Introduction
Nanotechnology involves research and technology development at atomic, molecular, or macromolecular levels and the use of functionalized nanostructures, nanodevices, and nanosystems that take advantage of specific properties of matter, which exist at the nanoscale. It plays significant role in medicine, especially in the management of severe diseases like cancer, leishmaniasis, AIDS, and diabetes (Agarwal et al. Citation2009). Nanotechnology can be exploited to deliver drugs, peptides, proteins, and nucleic acids using various delivery systems and plays a significant role in drug delivery and targeting of pharmaceutical, therapeutic, and diagnostic agents (Allard-Vannier et al. Citation2012, Bethune et al. Citation1993).
Carbon nanotubes (CNTs) were fully described by Sumio Iijima (Iijima Citation1991) of Nippon Electronic Company in 1991 and it represents unique and outstanding physicochemical properties in drug delivery and targeting (Hirlekar et al. Citation2009, Mehra et al. Citation2008, Mehra and Palakurthi Citation2015, Pruthi et al. Citation2012). CNTs mediated targeted and controlled drug delivery has aroused growing awareness as precious, promising nanoarchitecture due to its unique physicochemical properties, including a high aspect ratio, ultralight weight, high biocompatibility, and huge surface area in the treatment of cancer (Bhadra et al. Citation2003, Cao and Feng Citation2008, Chiang et al. Citation2001, Das et al. Citation2013, Datsyuk et al. Citation2008, De la Cruz et al. Citation2012).
CNTs are insoluble in water (hydrophobic in nature) or organic solvents, nonbiodegradable, have short blood circulation half-life (3–3.5 h) and are excreted through urine (94%) and feces (6%) (Huang et al. Citation2011, Sharma et al. Citation2015). Depending upon their diameter, lengths, and the presence of number of walls, CNTs can be single-, double-, triple-, multiwalled CNTs (Mehra and Jain Citation2015a,Citationb, Citation2016, Mehra et al. Citation2015). As literature suggests that the multifunctional CNTs have higher surface area and available major and minor grooves for loading and conjugation of high drug loading and entrapment capability as compared with other existing nanomaterials.
Pristine CNTs usually contain metallic particles, amorphous carbon, and multishell impurities in large amounts. Purification must be conducted before the utilization of nanotubes to remove and separate impurities from nanotubes for drug delivery and targeting (Huczko Citation2002, Iijima and Ichihashi Citation1993, Ren et al. Citation2012). Different methods used for the purification of CNTs are cutting (cutting of CNTs into smaller pieces) (Jain et al. Citation2009), microwave treatment (controlled temperature and pressure conditions with a minimal need for solvents) (Jain et al. Citation2009), vacuum oven (Kayat et al. Citation2016), ultrasonication (separate the impurities from pristine CNTs) (Li et al. Citation2007), chemical oxidation (conjugation of CNTs with biomolecules by using HNO3, H2O2:NH4OH, HNO3:H2SO4, and HNO3: HCl) (Lodhi et al. Citation2013, Mehra and Jain Citation2013, Spinato et al. Citation2016).
In the present research study, our main aim was to the development and evaluation of ligand-anchored MWCNTs for targeting to breast cancer with a spatial focus on the MWCNTs surface functionalization (FA-PEG bis amine functionalized and carboxylated MWCNTs) and compared it with free 5-FU solution. 5-FU-loaded purified carboxylated MWCNTs and FA-PEG bis amine-MWCNTs was developed and evaluated the in vitro release, ex vivo and in vivo study.
Materials and methods
Raw (Pristine) MWCNTs were procured from Sigma Aldrich Pvt. Ltd. 5-FU was received as a benevolent gift from United Biotech, New Delhi, India. PEG-bis amine was purchased from Sigma Aldrich Pvt. Ltd. Dulbecco’s modified Eagle medium (DMEM) was obtained from Hi-Media, Mumbai. Dicyclo hexyl carbodiimide (DCC), dimethyl sulfoxide (DMSO), N-hydroxy succinimide (NHS), nitric acid, ammonium hydroxide, hydrogen peroxide, and folic acid (FA) were purchased from Lobachemie Pvt. Ltd. Ultrapure water was purchased by Millipore System and other reagents were obtained from the Lobachemie and used as received.
Purification of procured MWCNTs
The procured MWCNTs were purified by selective oxidation method as reported by Li et al. (Citation2007) with slight modifications. Initially, pristine MWCNTs were purified using vacuum oven (kept inside the Hot Air Sterilizer, New Delhi, India at 250 ± 2 °C for 1 h) (Jain et al. Citation2009), then microwave (kept in the domestic microwave at 100 ± 2 °C for 15 min) (Mehra et al. Citation2014) and subsequently strong acid treatment (H2SO4:HNO3 1:3) followed by NH4OH and H2O2 to remove any metallic or amorphous impurities from the unpurified carboxylated MWCNTs (Mittal et al. Citation2010, Yudianti et al. Citation2011). The particle size and polydispersity index (PDI) of purified MWCNTs were characterized by zeta sizer (Delsa Nano C particle Analyzer, Beckman coulter). Purified MWCNTs was confirmed by FTIR spectroscopy (Parkin Elmer, Shelton, CT).
Dispersion of MWCNTs in surfactants
The MWCNTs (0.50 mg) were dispersed in 0.5 mL of different surfactants (sodium lauryl sulfate, Tween-80, and Triton X-100) and 2 mL of distilled water and sonicated (Steryl Medi Equip, 40,050, India) for 2 h. The different dispersions were visualized at different time intervals (Mehra et al. Citation2014).
Conjugation of FA-PEG bis amine to carboxylated MWCNTs
Activation of folic acid
Folic acid (FA) (1 g) was dissolved in DMSO:TEA (40:0.5 mL) was added in a reaction vessel and mixture of N-hydroxy succinimide (NHS) (0.52 g) and N,N-dicyclo hexyl carbodiimide (DCC) (0.50 g) was added with continuous stirring (at room temperature in dark) for 18 h (Mehra and Jain Citation2013). For the removal of precipitated by product (dicyclohexylurea; DCU) mixture was filtered. Further, triethylamine (TEA) was removed by evaporation under reduced pressure (temperature –20 °C; pressure – 400 Pascal; rpm-50), and remaining solution (activated FA; FA-NHS) was stored at −20 °C. The obtained product was characterized through FT-IR spectroscopy.
Conjugation of activated FA and PEG bis amine
The activated FA (FA-NHS) was conjugated to PEG-bis amine (Sigma Aldrich, St. Louis, MO) according to previously reported method after slight modifications (Mehra and Jain Citation2013). The activated ester FA-NHS (8 mg) was mixed with 112.5 mg of PEG bis amine (Sigma Aldrich), in the presence of DMSO (40 mL) and TEA (4.0 μL) in the reaction vessel, after which the mixture was magnetically stirred continuously at 100 rpm for 24 h. Folate-conjugated PEG bis amine (FA-PEG bis amine) as pale yellow solution was detected using UV-Vis spectrophotometer (λmax 363 nm) (Mehra et al. Citation2015, Mehra and Jain Citation2013).
Preparation of FA-PEG bis amine/MWCNTs
Carboxylated MWCNTs (10 mg) were dispersed in the mixture of EDC and DMSO (6.41 mg/mL) in the reaction bottle and FA-PEG bis amine (3 mL) was added to it with continuous magnetic stirring for 6 h at 100 rpm, after which the mixture was stirred for 5 days. The remaining product was purified by dialysis (5–6 kDa, HiMedia, Mumbai, India) to remove the un-conjugated FA-PEG bis amine. The purified product (FA-PEG bis amine/MWCNTs) was collected and characterized by FTIR spectroscopy (Mehra et al. Citation2015, Mehra and Jain Citation2013, Zheng et al. Citation2009).
Drug loading
To the best of our knowledge, 5-FU was used for the first time in functionalized MWCNTs. 5-FU (5 mg) was added to the mixture of acetone (0.2 mL) and TEA (0.3 mL). The solution was magnetically stirred vigorously for 2–3 min and the dispersion of MWCNTs (5 mg) in phosphate buffer pH 7.4 (2.5 mL) was added in the drug solution with continuous stirring for overnight (24 h). The amount of bound drug was estimated spectrophotometerically ( Bhadra et al. Citation2003, Huang et al. Citation2011, Mehra and Jain Citation2013, Tavakolifard et al. Citation2015). For loading of 5-FU in FA-PEG bis amine/MWCNTs conjugate 5-FU (25 mg) was added in the dispersion of FA-PEG bis amine/MWCNTs (5 mL). The 5-FU-FA-PEG bis amine/MWCNTs mixture was magnetically stirred over night for 24 h. This conjugate was dialyzed under strict sink conditions at 10 min (to remove the free drug from formulation), which was then estimated spectrophotometerically (Shimadzu, Japan) to determine the amount of drug bound with system.
In vitro study
In vitro release of 5-FU from 5-FU/MWCNTs and 5-FU/FA-PEG bis amine-MWCNTs formulation was studied in phosphate buffer (pH 7.4) and acetate buffer (pH 5.0) at room temperature (37 ± 0.5 °C) in 5, 10, 15, 20, 30, 60, 90, 120, 150, 180, and 900 min time points. 5-FU-loaded formulations (5-FU/MWCNTs and 5-FU/FA-PEG bis amine-MWCNTs) was filled in dialysis membrane (MWCO 5–6 kDa, Hi Media, India) separately, hermetically tied at both ends and instantly located into the receptor media maintaining strict sink conditions with steady stirring using magnetic stirrer at room temperature (100 RPM; Remi, Mumbai, India). The samples were collected at different time points and concentration of 5-FU was determined in triplicate by UV/Visible spectrophotometer at λmax 266 nm (UV/Vis, Shimadzu 1601, Kyoto, Japan) (Ren et al. Citation2012, Shi et al. Citation2009, Singh et al. Citation2008).
Cell line study
Cytotoxicity studies
MCF-7 (Michigan Cancer Foundation-7) human breast cancer cell was procured from National Center of Cell Sciences (NCCS), Pune, India, which is of folate over expression. Cells were cultured in DMEM containing 10% FBS and 1% penicillin/streptomycin. Cells were cultured in humidified environment at 37 °C with CO2. MCF-7 cells were incubated in 96-well plates for 12 h, the old medium was removed, and after incubation, cells were incubated in media containing 5-FU-loaded MWCNTs and FA-PEG bis amine/MWCNTs at the equivalent drug concentration (1–10 μg/mL). MTT assay was used to measure the cell viability at given time intervals. The absorbance of each well was measured by microplate reader (ELISA) with wavelength at 266 nm (Yoong et al. Citation2014).
Cell uptake study
Folate receptors overexpressed MCF-7 cells were cultured on glass cover slips placed into tissue culture plates and incubate the cell overnight. After overnight growth, supernatants from the culture of 5-FU-loaded MWCNTs and FA-PEG bis amine/MWCNTs at 37 °C were added. Upon incubation for 2 h, cells were washed and observed under fluorescence microscopy (FSM) after excitation at 266 nm.
In vivo study
The Wistar rats of either sex (200–250 g) were used for present animal studies in accordance with the guidelines by Committee for the Prevention, Control and Supervision of Experimental animals (CPCSEA) ISFCP/IAEC/CPCSEA/Meeting No 15/2015/Protocol No.261 from ISF College of Pharmacy, Moga Punjab, India. All the experimental protocols were approved by the Institutional Animal Ethics Committee.
Analysis of pharmacokinetic profile after dosing
The pharmacokinetic parameters were calculated after i.v. administration of free 5-FU and 5-FU/MWCNTs and 5-FU-FA-PEG bis amine/MWCNTs nanoformulations with the calculated i.v. dose (3.0 mg/kg body weight dose). The blood samples were collected from the retro-orbital plexus of eyes into the Hi-anti-clot blood collecting vials at predetermined time point’s up to 24 h. Supernatant was collected after centrifugation, trichloroacetic acid (TCA) in methanol (10% w/v) was added, vortexed, and ultracentrifuged (Rami, New Delhi). The clear supernatant was collected after centrifugation and 5-FU concentration was determined by high-performance liquid chromatography (HPLC) method and different pharmacokinetic parameters were calculated by Kinetica, Version 5.0 (Mattos et al. Citation2013, Mehra et al. Citation2014, Singh et al. Citation2008).
Biodistribution study
The in vivo biodistribution of the 5-FU/MWCNTs, 5-FU-FA-PEG bis amine/MWCNTs formulations and free 5-FU were studied on wistar rats. The free 5-FU, 5-FU/MWCNTs, and 5-FU-FA-PEG bis amine/MWCNTs conjugates were administered i.v. through tail vein route according to body weight of animals. Rats were carefully sacrificed after predetermined time intervals 6, 12, and 24 h for the collection of organs such as liver, lung, kidney, and spleen immediately. Organs were homogenized after addition of chloroform (CHCl3) and methanol (CH3OH) mixture and ultracentrifuged at 2000 rpm for 25 min. After centrifugation, collect the solid-dried residue. Concentration of 5-FU were determined by HPLC (Shimadzu, C18, Japan) method, wherein mobile phase consisted of water/acetonitrile (90:10; v/v) (Singh et al. Citation2008).
Statistical analysis
Data were expressed as the means with 95% confidence intervals. Statistical test were performed with one-way analysis of variance (ANOVA) test. For all tests, P values less than 0.05 (P < 0.05) were considered to be statistically significant.
Results and discussion
Pristine CNTs are not suitable due to intrinsic aqueous insolubility and existence of impurities. The procured pristine MWCNTs from Sigma Aldrich Pvt. Ltd. were purified in an oven, microwave and afterward strong acid treatment (H2SO4:HNO3) (separate the impurities metallic or amorphous) followed by NH4OH and H2O2 and cut (shorten nanotubes) prior to use. and show the FTIR of oven which shows the peaks of OH and NH stretching at 3447.12 and 2945.58 cm−1, respectively, and acid-treated MWCNTs depicts the peaks of COOH (3062.11 cm−1), asymmetric stretching of C = O (1633.58 cm−1) and C–O stretch (1289.12 cm−1) and shows the particle size of purified CNTs. The longer nanotubes are unable to enter most of the cancerous cells and may be toxic. Oxidation is one of the most common techniques for hydrophilic functionalization of the nanotubes through strong oxidizing acids.
Table 1. Effects of sonication on particle size and polydispersity index on functionalization of CNTs.
The dispersion characteristics of MWCNTs (0.50 mg) in different surfactants (sodium lauryl sulfate, Tween-80, and Triton X-100) are shown in . The surface of the MWCNTs was modified using different surfactants, in presence of sonication. Sodium lauryl sulfate (SLS), showed the maximum dispersion of MWCNTs, CNTs were sediment in Tween-80. The dispersion of MWCNTs in different surfactants after 48 h is as follows:
The FTIR spectrum of procured FA is shown in . FTIR spectrum of the activated folic acid shows the peaks at 1651, 1712, and 1557 cm−1 which shows the presence of CH stretching, NH stretching, and asymmetric stretching of C = O, respectively. Folic acid and PEG bis amine conjugate was prepared from PEG bis amine by successive conjugation with activate folic acid. The FA-PEG bis amine conjugate was obtained as a pale yellow liquid. FTIR spectrum of conjugate demonstrates that folic acid was indeed covalently linked to the PEG via amide bond (). FTIR depicts the peaks of amide I C = O (1651 cm−1), amide II (1i bond) (1644.63 cm−1), amide III (2i bond). FTIR spectrum of FA-PEG bis amine/MWCNT shows the peaks of aromatic compounds indicated the presence of FA and C–O stretch of ether linkage shown in .
The drug-loading efficiency is the significant, precondition characteristic in development and characterization of targeted drug delivery system. The drug entrapment efficiency was studied following a modified dialysis diffusion technique and was found to be significantly higher. The entrapment of 5-FU in MWCNTs was monitored by UV/Visible absorption to confirm indirectly, the drug entrapment in MWCNTs and FA-PEG bis amine, during which the characteristic absorption peak of 5-FU at 266.61 nm was shifted, indicative of interaction between 5-FU and MWCNTs. The entrapment efficiency was found to be 99.8% in MWCNTs and 99.5% in FA-PEG bis-amine MWCNTs and 5-FU-MWCNTs.
The in vitro behavior of 5-FU/MWCNTs and 5-FU-FA-PEG bis amine/MWCNTs in phosphate buffer (pH 7.4) and acetate buffer (pH 5.0) using dialysis bag method is shown in , which displayed an initial faster followed by sustained pattern. In the acidic pH-sustained release pattern due to the ionization of 5-FU, our release profile was in good agreement with previously published reports (Bhadra et al. Citation2003, Mehra and Jain Citation2013, Mehra et al. Citation2014, Ren et al. Citation2012,Yoong et al. Citation2014).
Figure 7. % Cumulative release from: (A) 5-FU-MWCNTs (B) 5-FU-FA-PEG-MWCNTs formulations in PBS 7.4 and acetate buffer 5.0.
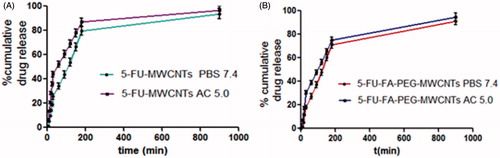
The methylthiazole tetrazolium (MTT) cytotoxicity assay was performed by the cleavage of tetrazolium salt [{3–(4,5 dimethyl thiazole-2 yl)-2,5-diphenyl tetrazolium bromide} (MTT)] to a blue formazan derivative by living cells. The MTT assay was performed to determine the cancer-targeting propensity of the 5-FU laden developed optimized nanotubes formulation using MCF-7 (human breast cancer) cell line and compared with FA-PEG bis amine-MWCNTs and free 5-FU solution. The cytotoxicity assay clearly demonstrated that upon increasing the concentration from 0.01 to 10 μg/mL. The percent viability of the cancerous cells was decreased owing to apoptosis by intercalating 5-FU (). Our cytotoxicity results are in accordance with the previous published reports.
Figure 8. Percent cell viability of MCF-7 cell after treatment with free 5-FU, 5-FU/MWCNTs and 5-FU/MWCNTs (n = 3).
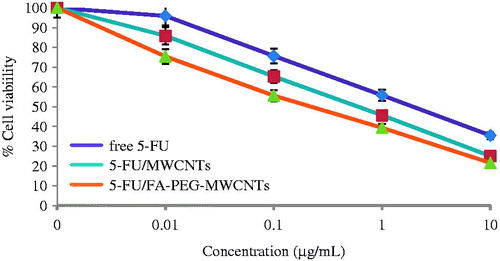
The qualitative cellular uptake of 5-FU and 5-FU-loaded MWCNTs formulations is studied and presented in . The 5-FU-FA-PEG bis amine/MWCNTs shows higher fluorescence intensity as compared with other formulation and control group. Similarly, higher cell uptake fluorescence intensity was observed for 5-FU/FA-PEG bis amine-MWCNTs, as compared with 5-FU/MWCNTs and free 5-FU. The observed higher fluorescence intensity clearly suggests the higher uptake of the 5-FU/FA-PEG bis amine-MWCNTs formulations, possibly due to the receptor-mediated endocytosis (RME) as well nanoneedle-specific (passive diffusion) mechanism.
Figure 9. Qualitative cell uptake of the free 5-FU, 5-FU/MWCNTs and 5-FU-FA-PEG-MWCNTs in MCF-7 cells.
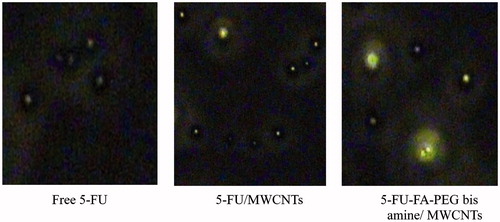
In vivo bioavailability and pharmacokinetic studies of free 5-FU, 5-FU-MWCNTs, and 5-FU-FA-PEG bis amine-MWCNTs were conducted on Wistar rats. Following i.v. dosage of sample, blood samples were collected at specified time points and 5-FU concentration was determined in plasma by HPLC method. Results obtained are plotted with plasma concentration versus time as shown in . Pharmacokinetic parameters were determined using noncompartment modeling as summarized in . The elimination half-life (t1/2) of 5-FU-FA-PEG bis amine/MWCNTs, 5-FU/MWCNTs, and free 5-FU was found to be 16.4754, 8.6439, and 5.6192, while MRT was found to be 17.0036, 10.3746, and 5.2347, respectively. Thus, indicating an overall increase in bioavailability of 5-FU due to the targeted delivery through ligands conjugated MWCNTs.
Figure 10. Biodistribution patterns of free 5-FU, 5-FU-MWCNTs and 5-FU-FA-PEG bis amine-MWCNTs in different organs.
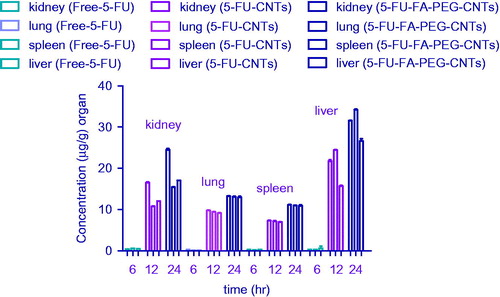
Table 2. Pharmacokinetic parameters of free 5-FU, 5-FU-MWCNTs, and 5-FU-FA-PEG bis amine-MWCNTs.
A comparative biodistribution study was performed among the free 5-FU, 5-FU-MWCNTs, and 5-FU-FA-PEG bis amine-MWCNTs formulations administered i.v. to compare the amount of 5-FU that reaches different tissue like liver, spleen, kidney, and lungs at different time intervals. represents the tissue distribution of free 5-FU, 5-FU-MWCNTs, and 5-FU-FA-PEG bis amine-MWCNTs after i.v. administration in rats. Tissue biodistribution study was conducted to evaluate the drug delivery at the different sites of interest like liver, spleen, kidneys, and lungs. The free 5-FU was primarily accumulated progressively in liver up to 6-h postinjection. After 12 h, only 0.51 ± 0.09 mg/g of drug concentration was found in liver, whereas spleen, kidney, and lungs were found to have drug levels up to 0.25 ± 0.03, 0.39 ± 0.01, and 0.51 ± 0.02 mg/g, respectively. When 5-FU-MWCNTs and 5-FU-FA-PEG bis amine-MWCNTs was administered, appreciably lesser amounts were present in spleen and lungs. The purified MWCNTs that are devoid of free terminal functional groups, readily goes into the liver. According to previously published report, after i.v. injection, CNTs are mainly excreted by urine and feces. The 5-FU-MWCNTs and 5-FU-FA-PEG bis amine-MWCNTs is mainly accumulated in liver. The appreciable amount of drug from the developed CNTs formulation was found in liver. No significant accumulation of 5-FU-MWCNTs and 5-FU-FA-PEG bis amine-MWCNTs was observed in spleen and lungs. As the folate conjugation was carried out on the carboxylated MWCNTs, the dispensability and hydrophilicity of MWCNTs were enhanced significantly as revealed by dispersion and loading experimentation. In general, 5-FU-MWCNTs and 5-FU-FA-PEG bis amine-MWCNTs formulation was administered by i.v. route; the concentration of drug was comparatively lesser in all the tissues than the free 5-FU in the initial 6 h, slight increase in 12 h and reduction due to the clearance in 12-h postinjection. This outcome could be attributed to the fact that 5-FU-MWCNTs and 5-FU-FA-PEG bis amine-WWCNT released appreciable drug in the systemic circulation than in any other tissue. The MWCNTs are nonbiodegradable in nature and known to be excreted by biliary pathway from the liver through the bile duct, intestine, which ends up in feces.
Conclusion
The multiwalled carbon nanotubes (MWCNTs) have been continuously attracting increasing consideration in targeted and controlled drug delivery. As we know that the functionalized CNTs provide greater stability as well as loading efficiency and better and alternative platform in biomedical applications including targeted drug delivery. In the present investigation, our main aim was to develop, evaluate, and investigate the cancer-targeting propensity of the 5-FU-loaded functionalized MWCNTs (PEGylation) using human breast cancer cells. The in vitro, ex vivo and in vivo studies clearly revealed that the 5-FU/PEG-MWCNTs showed promising potential in cancer targeting and effectively kill cancerous cells. The pharmacokinetics studies also showed that the developed MWCNTs formulations are long circulating (stealth) in nature and increases dispersibility of CNTs. Finally, it can conclude that the 5-FU-loaded FA-PEG bis amine/MWCNTs formulation showed efficient 5-FU release at desired site.
Disclosure statement
The authors report no conflicts of interest. The authors alone are responsible for the content and writing of this article.
References
- Agarwal A, Gupta U, Asthana A, Jain NK. 2009. Dextran conjugated dendritic nanoconstructs as potential vectors for anti-cancer agent. Biomaterials. 30:3588–3596.
- Allard-Vannier E, Cohen-Jonathan S, Gautier J, Herve-Aubert K, Munnier E, Souce M, et al. 2012. Pegylated magnetic nanocarriers for doxorubicin delivery: a quantitative determination of stealthiness in vitro and in vivo. Euro J Pharm Biopharm. 81:498–505.
- Bethune D, Klang C, Vries D, Gorman M, Savoy G, Vazquez RJ, Beyers R. 1993. Cobalt-catalysed growth of carbon nanotubes with single-atomic-layer walls. Nature. 363:605–607.
- Bhadra D, Bhadra S, Jain S, Jain N. 2003. A PEGylated dendritic nanoparticulate carrier of fluorouracil. Int J Pharm. 257:111–124.
- Cao N, Feng SS. 2008. Doxorubicin conjugated to D-alpha-tocopheryl polyethylene glycol 1000 succinate (TPGS): conjugation chemistry, characterization, in vitro and in vivo evaluation. Biomaterials. 29:3856–3865.
- Chiang I, Brinson B, Huang A, Willis P, Bronikowski M, Margrave J, Smalley R, Hauge R. 2001. Purification and characterization of single-wall carbon nanotubes (SWNTs) obtained from the gas-phase decomposition of CO (HiPco process). J Phys Chem B. 5:8297–8301.
- Das M, Datir SR, Singh RP, Jain S. 2013. Augmented anticancer activity of a targeted, intracellularly activatable, theranostic nanomedicine based on fluorescent and radiolabeled, methotrexate-folic acid-multiwalled carbon nanotube conjugate. Mole Pharm. 10:2543–2557.
- Datsyuk V, Kalyva M, Papagelis K, Parthenios J, Tasis D, Siokou A, Kallitsis I, Galiotis C. 2008. Chemical oxidation of multiwalled carbon nanotubes. Carbon. 46:833–840.
- De la Cruz EF, Zheng Y, Torres E, Li W, Song W, Burugapalli K. 2012. Zeta potential of modified multi-walled carbon nanotubes in presence of poly (vinyl alcohol) hydrogel. Int J Electrochem Sci. 7:3577–3590.
- Hirlekar R, Yamagar M, Garse H, Vij M, Kadam V. 2009. Carbon nanotubes and its applications: a review. Asian J Pharm Clinl Res. 2:17–27.
- Huang H, Yuan Q, Shah J, Misra R. 2011. A new family of folate-decorated and carbon nanotube-mediated drug delivery system: synthesis and drug delivery response. Adv Drug Delivery Rev. 63:1332–1339.
- Huczko A. 2002. Synthesis of aligned carbon nanotubes. Appl Phys A. 74:617–638.
- Iijima S, Ichihashi T. 1993. Single-shell carbon nanotubes of 1-nm diameter. Nature. 363:603–605.
- Iijima S. 1991. Helical microtubules of graphitic carbon. Nature. 354:56–58.
- Jain AK, Dubey V, Mehra NK, Lodhi N, Nahar M, Mishra DK, Jain NK. 2009. Carbohydrate-conjugated multiwalled carbon nanotubes: development and characterization. Nanomed Nanotechnol Biol Med. 5:432–442.
- Kayat J, Mehra NK, Gajbhiye V, Jain NK. 2016. Drug targeting to arthritic region via folic acid appended surface engineered multi-walled carbon nanotubes. J Drug Target. 24:318–327.
- Li CC, Lin JL, Huang SJ, Lee JT, Chen CH. 2007. A new and acid-exclusive method for dispersing carbon multi-walled nanotubes in aqueous suspensions. Colloid Surface A. 297:275–281.
- Lodhi N, Mehra NK, Jain NK. 2013. Development and characterization of dexamethasone mesylate anchored on multi walled carbon nanotubes. J Drug Target. 2013 21:67–76.
- Mattos ACD, Khalil NM, Mainardes RM. 2013. Development and validation of an HPLC method for the determination of fluorouracil in polymeric nanoparticles. Brazilian J Pharma Sci. 49:117–126.
- Mehra NK, Jain AK, Lodhi N, Raj R, Dubey V, Mishra D, et al. 2008. Challenges in the use of carbon nanotubes for biomedical applications. Crit Rev Ther Drug Carr Syst. 25:169–206.
- Mehra NK, Jain K, Jain NK. 2015. Pharmaceutical and biomedical application of surface engineered carbon nanotubes. Drug Discov Today. 20:750–756.
- Mehra NK Jain N. 2013. Development, characterization and cancer targeting potential of surface engineered carbon nanotubes. J Drug Target. 21:745–758.
- Mehra NK, Jain NK. 2015a. Cancer targeting propensity of folate conjugated surface engineered multi-walled carbon nanotubes. Colloid Surf B. 132:17–26.
- Mehra NK, Jain NK. 2015b. One platform comparison of estrone and folic acid anchored surface Engineered MWCNTs for doxorubicin delivery. Mol Pharm. 12:630–643.
- Mehra NK, Jain NK. 2016. Multifunctional hybrid-carbon nanotubes: new horizon in drug delivery and targeting. J Drug Target. 24:294–308.
- Mehra NK, Palakurthi S. 2015. Interactions between carbon nanotubes and bioactives: a drug delivery perspective. Drug Discov Today. doi: 10.1016/j.drudis.2015.11.011.
- Mehra NK, Verma AK, Mishra P, Jain N. 2014. The cancer targeting potential of d-α-tocopheryl polyethylene glycol 1000 succinate tethered multi walled carbon nanotubes. Biomaterials. 35:4573–4588.
- Mittal V, Patel S, Sheth S. 2010. Development and characterization of folate targeted nanoparticle drug delivery system. Int J Pharm Bio Sci. 1:1–2.
- Pruthi J, Mehra NK, Jain NK. 2012. Macrophages targeting of amphotericin B through mannosylated multiwalled carbon nanotubes. J Drug Target. 20:593–604.
- Ren J, Shen S, Wang D, Xi Z, Guo L, Pang Z, et al. 2012. The targeted delivery of anticancer drugs to brain glioma by PEGylated oxidized multi-walled carbon nanotubes modified with angiopep-2. Biomaterials. 33:3324–3333.
- Sharma P, Mehra NK, Jain K, Jain NK. 2015. Biomedical applications of carbon nanotubes: a critical review. Curr Drug Deliv. In press.
- Shi X, Wang SH, Shen M, Antwerp ME, Chen X, Li C, et al. 2009. Multifunctional dendrimer-modified multiwalled carbon nanotubes: synthesis, characterization, and in vitro cancer cell targeting and imaging. Biomacromolecules. 10:1744–1750.
- Singh P, Gupta U, Asthana A, Jain NK. 2008. Folate and folate-PEG-PAMAM dendrimers: synthesis, characterization, and targeted anticancer drug delivery potential in tumor bearing mice. Bioconjug Chem. 19:2239–2252.
- Spinato C, Giust D, Vacchi IA, Menard-Moyon C, Kostarelos K, Bianco A. 2016. Different chemical strategies to aminate oxidized multi-walled carbon nanotubes for siRNA complexation and delivery. J Mater Chem B. 4:431–441.
- Tavakolifard S, Biazar E, Pourshamsian K, Moslemin MH. 2015. Synthesis and evaluation of single-wall carbon nanotubes-paclitaxel-folic acid conjugate as an anti-cancer targeting agent. Artif Cells, Nanomed Biotechnol. doi: 10.3109/21691401.2015.1019670.
- Yoong SL, Wong BS, Zhou QL, Chin CF, Li J, Venkatesan T, et al. 2014. Enhanced cytotoxicity to cancer cells by mitochondria-targeting MWCNTs containing platinum(IV) prodrug of cisplatin. Biomaterials. 35:748–759.
- Yudianti R, Onggo H, Sudirman YS, Iwata T, Azuma JI. 2011. Analysis of functional group sited on multi-wall carbon nanotube surface. Open Mater Sci J. 5:242–247.
- Zheng Y, Cai Z, Song X, Chen Q, Bi Y, Li Y, Hou S. 2009. Preparation and characterization of folate conjugated N-trimethyl chitosan nanoparticles as protein carrier targeting folate receptor: in vitro studies. J Drug Target. 17:294–303.