Abstract
The body is protected against the external environment by the skin due to its physical barrier nature. Stratum corneum composed of corneocytes surrounded by lipid region performs a major barrier function as it lies in the uppermost area of skin. Alteration in barrier function, increase in permeability, and disorganization of stratum corneum represent diseased skin. Drugs applied to the diseased skin should induce a local effect at the site of application or area close to it along with cutaneous absorption rather than percutaneous absorption. Conventional formulations like ointments, gels, and creams suffer from the drawback of limited local activity. For the enhancement of drug penetration and localization of the drug at the site of action approaches explored are liposomes, niosomes, ethosomes microparticles, and solid lipid nanoparticles. Vesicles composed of fatty acids like oleic acid and linoleic acid represent the new approach used for transdermal penetration and localization. In this review article, our major aim was to explore the applications of fatty acid vesicles for transdermal delivery of various bioactives.
Introduction
Skin can be a beneficial route for the administration of various bioactive molecules, but the permeation of bioactive molecules through this domain becomes difficult due to its barrier like nature. As far as structure of skin is concerned, it is composed of several distinct layers like stratum corneum, epidermis (composed of granulosum, spinosum, and basal layers), and dermis. Stratum corneum acts as a main physical barrier which consists of protein-enriched dead cells known as corneocytes and lipid-enriched intercellular domain having specified content of cholesterol, fatty acids, and long-chain ceramides (Elias Citation1983). Presence of desmosomes, cytoskeletal elements along with adherent junctions in the epidermis also, creates hindrance for transdermal delivery of various drug molecules (Proksch et al. Citation2008). For effective transdermal delivery of any drug molecule, it should penetrate the skin efficiently through the process of passive diffusion. Drug molecules applied to the skin may either follow transappendageal or transepidermal pathway for permeation purpose. On transappendageal route, drug molecules have to come across through the hair follicles or sebaceous glands. Pilosebaceous units may be drug target in this pathway for localized delivery (Knorr et al. Citation2009). In transepidermal pathway, drug molecules pass through either corneocytes or lipid matrix (known as transcellular pathway), or they can travel across the lipid domains between corneocytes (known as intercellular pathway). Intercellular route is the most common route for transepidermal penetration as it is followed by most of the drugs (Bouwstra and Ponec Citation2006). Lipid vesicles have become a center of attraction for pharmaceutical scientists to improve the transdermal delivery of various bioactive molecules from last one decade. Vesicular systems explored for improved transdermal delivery include liposomes, niosomes, elastic deformable vesicles, and ethosomes (Sinico and Fadda Citation2009). Vesicular systems have the capacity to release the drug in a controlled fashion along with the formation of a localized depot in the skin which enhances transdermal delivery. The efficacy of these vesicular systems is influenced by vesicle composition, preparation method as it affects the physicochemical behavior of vesicles (Elsayed et al. Citation2007). Fatty acids can act as potent skin penetration enhancers. Various drugs like indomethacin, acyclovir, naloxone, and nitroglycerine have shown increased transdermal penetration in the presence of fatty acids. This is due to reason that fatty acid cause disruption of densely packed lipids present in the extracellular space of stratum corneum (Aungst Citation1989). Therefore, the use of fatty acid vesicles has been suggested for the transdermal delivery of various bioactive molecules and they are the novel vesicular systems utilized for the purpose (Zakir et al. Citation2011). Fatty acid vesicle can follow intercellular and transappendageal pathway for transdermal penetration. The size of the fatty acid vesicles is the governing factor for the pathway that can be followed by the vesicles. If the vesicle size is 3–12 μm, then they follow transappendageal pathway and if less than 3 μm, then the intercellular pathway is followed (Ehdaie Citation2011) (). The present review describes the method of preparation, characterization, and utilization of fatty acid vesicles for transdermal drug delivery.
A briefing to fatty acids
The fatty acids are generally characterized as amphiphilic molecules having a chain of carbon atoms acting as nonpolar part and a terminal carboxylic group acting as polar part. The general formula for fatty acid is –CH3(CH2)nCOOH. Fatty acids may be saturated or unsaturated depending upon the existence of a number of double in their structure. Saturated fatty acids may have only single bonds in their structure while unsaturated fatty acid may have double or triple bonds. Unsaturated fatty acid may exist in cis or trans configuration depending upon the arrangement of double bond in space (Pohl et al. Citation2000). Presence of fatty acids in various body fluids and tissues has been notified and they can play important role in various biological functions. They may act as a source of energy for cells when present in cytoplasm. A significant amount of fatty acid may be present in the skin and they help for in making skin as a competent barrier (Placensia et al. Citation2007). Percentages of various fatty acids present in the skin are shown in . Fatty acids can act as fuel for various activities, whether mechanical or electrical as they influence the performance of cardiac cells (Kong and Rabkin Citation2002). Fatty acid vesicles are supposed to have distinct roles in protocells formation due to their capacity to grow, self assemble, and divide (Deamer et al. Citation2001).
Table 1. Major fatty acids present in skin (Zakir et al. Citation2011).
Fatty acid vesicles
Ability of unsaturated fatty acids like oleic acid and linoleic to form vesicles was observed by Gebicki and Hicks (Citation1973). Hargreaves and Deamer (Citation1978) reported that saturated fatty acids having carbon atoms 8–12 in number could also be assembled in the vesicular form. Fatty acid vesicles have high penetration through the hydrophobic layer like skin so they can be effectively used as carrier systems for various enzymes, drugs, or other macromolecules (Bouwstra and Honeywell-Nguyen Citation2005). Method of preparation of fatty acid vesicles is easy and they are cost-effective drug delivery systems. Phospholipidic vesicles like liposomes have a low monomer concentration in water due to their low solubility. This is the reason of low exchange between the vesicles of such type. While, the fatty acid vesicles due to their high solubility provide good partitioning in various natural membranes (Robinson et al. Citation2006).
Self assembling of fatty acids into vesicles and pH
Capacity of fatty acid molecules to get assembled into vesicles form depends upon pH. It was found in a research that ionization of the half carboxylic group could provide a strong base for the formation of stable fatty acid vesicles. This process occurs in the narrow pH range and this is one of the causes diverting attention of scientists from fatty acid vesicles to be used as drug carrier (Apel et al. Citation2002). Incorporation of alcohol having same chain length as that of fatty acid enhances the vesicles stability by widening the pH range. Stability of fatty acid vesicles increases in upper pH ranges using this method. Surfactant with a sulfonate head group can also be used for the enhancement of vesicles stability at upper pH range (Hargreaves and Deamer Citation1978). Modification of the size of the hydrophilic head group in fatty acid leads to widening of pH range for vesicle formation. Attachment of the polar bulky group in the fatty acid molecule can lower pH region for vesicle formation along with reduction in phase-transition temperature (Renoncourt et al. Citation2006). Existence of fatty acid vesicles may occur in the neutral or ionized form depending upon the pH. Vesicle stability depends upon the ratio of the two forms (Walde and Morigaki Citation2007).
Methods of preparation of fatty acid vesicles
Various methods of preparation of fatty acid vesicles are depicted in . There are three generalized methods of preparation of fatty acid vesicle, namely, thin film hydration method, fatty acid vesicle preparation by alcohol addition, and autopoetic process of formation (Zakir et al. Citation2011).
Figure 2. Methods of preparation of Fatty acid vesicles. The thin-film hydration is most widely used technique for the purpose (modified from Zakir et al. Citation2011).
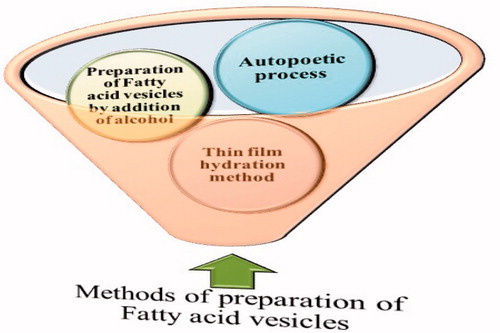
Thin-film hydration method
Thin-film hydration method is a pH-dependent process, and vesicle formation occurs in a narrow pH range. This method requires a very high concentration of the fatty acid. This method involves the addition of fatty acid in an organic solvent in a round bottom flask. Further, evaporation of the mixture is carried out until organic solvent evaporates completely. At the end, formation of thin film of fatty acid occurs which is hydrated with buffer solution of appropriate pH (Zakir et al. Citation2011). The various steps of the thin film hydration method are shown in .
Preparation of fatty acid vesicles by addition of alcohol
This is the new developed method in which addition of alcohol (having same chain length as that of fatty acid) leads to the formation of fatty acid vesicles. The various steps involved in this process are shown in . Fatty acid vesicles prepared by this method show good stability over a wide pH range. This is a time consuming process. To avoid this time consumption, fatty acid vesicles are prepared on the basis of the cooperative principle known as matrix effect. This method involves triggering of the rate of vesicle formation in the presence of pre-added fatty acid vesicles and liposomes in the system (Luisi et al. Citation2003).
Autopoetic process
Formation of fatty acid vesicles is possible even when aqueous solution of fatty acid is added to water-buffered solution because of the spontaneous pH change. When half of carboxylic acids present in fatty acid ionize, tendency of formation of vesicles generates in them (Luisi et al. Citation1998). Hydrocarbon chain encloses into a bilayer structure which is opposite to aqueous compartment. It causes minimization of interaction between hydrocarbon chain and water. Protonated and deprotonated groups, namely, HOOCR and RCOO–, show hydrogen-bond interaction (RCOO– ………. HOOCR) and provide stability to vesicles (Cistola et al. Citation1988).
Characterization of fatty acid vesicles
For the establishment of fatty acid vesicles as effective transdermal drug delivery system, they must be characterized appropriately. Various parameters of characterization of fatty acid vesicles are given below:
Vesicles morphology
Giant vesicle formation is observed when self assembling of fatty acid occurs at particular pH, temperature, and concentration. Adoption of the alcohol addition method leads to formation of vesicles of the size range 50 nm to several microns (Hanczyc et al. Citation2003). Fatty acid vesicles prepared through cooperative mechanism show reduction in size and polydispersity. But the size of vesicles taken initially governs these parameters (Zhang et al. Citation1996). Gebicki and Hicks (Citation1973) studied freeze-etched specimen of spheres made from oleic acid under an electron microscope (). They found sphere consisting of hydrophilic interior surrounded by one or more concentric layers of fatty acid membranes along with a collection of small spheres within the same envelope.
Figure 5. Electron micrographs of freeze-etched oleic acid spheres (× 41,650). (A) A central aqueous region surrounded by concentric membranes- the flat pitted area was cut by the microtome. (B) Smaller spheres enclosed by a common envelope. [Used with the permission Gebicki and Hicks Citation1973].
![Figure 5. Electron micrographs of freeze-etched oleic acid spheres (× 41,650). (A) A central aqueous region surrounded by concentric membranes- the flat pitted area was cut by the microtome. (B) Smaller spheres enclosed by a common envelope. [Used with the permission Gebicki and Hicks Citation1973].](/cms/asset/a88625d1-aa8f-4f19-b8c8-01884868f89b/ianb_a_1146729_f0005_b.jpg)
Edwards et al. (Citation1995) studied the effect of pH on the morphology of oleate vesicles in the presence of phosphatidylcholine vesicles using transmission electron microscopy (TEM; ). Fatty acids were found as long cylindrical micelles at pH 10. Unilamellar vesicles begin to form when pH reduced to 9 and there was formed of large clusters of aggregated vesicles at pH 8.
Figure 6. TEM images of oleate vesicles in presence of phosphatidylcholine vesicles at different pH, viz., (A) pH 10 (B) pH 9 (C) pH 8 [used with permission Edwards et al. Citation1995].
![Figure 6. TEM images of oleate vesicles in presence of phosphatidylcholine vesicles at different pH, viz., (A) pH 10 (B) pH 9 (C) pH 8 [used with permission Edwards et al. Citation1995].](/cms/asset/60606ad3-5c38-42b4-9d18-a88f390ab78e/ianb_a_1146729_f0006_b.jpg)
Entrapment efficiency
Rendón et al. (Citation2012) estimated entrapment efficiency of oleic acid vesicles through fluorescence measurement. Vesicles were hydrated using 0.2-M bicine and released content was estimated using the ANTS/DPX fluorescent probe system. Estimation was done at various pH, viz.,, 7.5, 8.5, and 10, and entrapment efficiency was found maximum at 8.5. Furthermore, Apel et al. (Citation2002) found that vesicles of decanoic acid/decanol could effectively encapsulate enzyme catalase and protect it from degradation by a protease. Incorporation of fatty acids as carriers in proniosomal hybrids along with an increase in their entrapment efficiency was observed by Bandyopadhyay and Johnson (Citation2007). The increase in entrapment efficacy was due to increase in vesicle size because of incorporation of fatty acids in bilayers.
Release of entrapped materials/drugs
Zakir et al. (Citation2010) studied the release of entrapped fluconazole from oleic acid vesicles using Franz diffusion cell having clamped polycarbonate membrane (pore size 50 nm) between donor and receptor compartment. Fatty acid vesicles released 40–60% entrapped drug within 24 hours in phosphate buffer saline (PBS; pH 7.4). Furthermore, Verma et al. (Citation2014) utilized dialysis bag method to study the release of entrapped clotrimazole in-vitro. After addition of a specified amount of vesicular dispersion in the dialysis membrane (Himedia, LA393–10MT), it was taken with 50 mL of PBS (pH 5.5). Oleic acid dispersion released 30–40% drug within 24 hours and showed zero-order release kinetics.
Permeation/flux studies
If a vesicular system is to be utilized for transdermal delivery then its permeation/flux study becomes an essential characterization parameter. El Maghraby et al. (Citation2001) performed Skin permeation/flux studies of 5-fluorouracil loaded ultradeformable lipid vesicles (liposomes) using an automated Franz diffusion cell. Abdominal Caucasian postmortem skin was sandwiched between receptor and donor compartment of the cell. Amount of drug permeated in case of ultradeformable lipid vesicles was near about 10 times higher compared with saturated aqueous solution of 5-fluorouracil (). The same technique may be applicable to the fatty acid vesicular system (Zakir et al. Citation2011). Zakir et al. (Citation2010) performed confocal laser scanning microscopy (CLSM) study to find out the depth of penetration of oleic acid vesicles in rat skin. Fluorescent probe is visualized in skin specimens by using the CLSM technique at different cross sections without cryofixing. For the purpose, Rhodamine-B-loaded vesicles were prepared for the study. The result of the study showed penetration of dye loaded vesicles upto 50–60 μm in rat skin.
Figure 7. Amount of 5-fluorouracil penetrated through human epidermal membrane after 12 (A) and 36 (B) hour of its application in ultra-deformable vesicles (▪) or saturated aqueous solution (▴), using receptors containing different concentrations of ethanol in aqueous sodium azide [used with permission El Maghraby et al. Citation2001].
![Figure 7. Amount of 5-fluorouracil penetrated through human epidermal membrane after 12 (A) and 36 (B) hour of its application in ultra-deformable vesicles (▪) or saturated aqueous solution (▴), using receptors containing different concentrations of ethanol in aqueous sodium azide [used with permission El Maghraby et al. Citation2001].](/cms/asset/11e67f9f-5557-4bf7-aa42-5effaf264c98/ianb_a_1146729_f0007_b.jpg)
Skin irritation study
Lopes et al. (Citation2006) prepared reverse hexagonal phase nano-dispersion of fatty acids (mono-olein and oleic acid) for skin delivery of ciclosporin A. For the skin irritation study of nano-dispersion of fatty acids histological changes in mice skin were observed after two days of application of a formulation. They evaluated skin for epidermal thickening, edema, and immunocyte infiltration in the dermis. Skin treated with nano-dispersion of fatty acid did not indicate any kind of such parameter and revealed nonirritating behavior of fatty acids toward skin (). Furthermore, Moreira et al. (Citation2010) performed skin irritation studies on poloxamer-based delivery system containing oleic acid loaded with lumiracoxib drug. Poloxamer (PF-127) gel containing oleic acid was applied on the rabbit skin and evaluated visually after 24, 72, and 120 hours. The formulation applied on the skin did not show any sign of irritation. The results of these studies show that vesicles composed of fatty acids may compensate the irritation of skin.
Figure 8. Analysis of the skin of untreated animals and animals subjected to topical application of saline or nanodispersion. (A) Light microscopy of untreated skin. (B) Light microscopy of skin treated with PBS. (C) Light microscopy of skin treated with the hexagonal phase nanodispersion containing oleic acid. (D) Thickness of the epidermis of untreated animals and those treated with saline or nanodispersion. Sections were microscopically analyzed using a 20 × objective; scale bar: 50 μm. The number of animals in each group was three [used with permission Lopes et al. Citation2006].
![Figure 8. Analysis of the skin of untreated animals and animals subjected to topical application of saline or nanodispersion. (A) Light microscopy of untreated skin. (B) Light microscopy of skin treated with PBS. (C) Light microscopy of skin treated with the hexagonal phase nanodispersion containing oleic acid. (D) Thickness of the epidermis of untreated animals and those treated with saline or nanodispersion. Sections were microscopically analyzed using a 20 × objective; scale bar: 50 μm. The number of animals in each group was three [used with permission Lopes et al. Citation2006].](/cms/asset/483fb956-f3fc-4932-a671-e33df824377f/ianb_a_1146729_f0008_c.jpg)
Stability studies
Maximum stability of fatty acid vesicles is observed within a narrow pH range. To enhance the stability and increase the pH range use of alcohol having same chain length as that of fatty acid has been suggested. In one study, it was found that the stability of vesicles made up of nonanoic acid increased over a wide pH range, i.e. upto pH of 11 by addition of nonanol (Zakir et al. Citation2011). Ahmad et al. (Citation2014) studies the effect of glycolipids on stability and electrophoretic mobility of decanoic acid vesicles. They found that the addition of small amounts of glycolipid reduced the critical vesicular concentration (CVC) of decanoic acid along with reduction in electrophoretic mobility. Presence of glycolipid also enhanced stability of decanoic acid vesicles by enhancing the bilayer curvature through hydrogen bond within the polar region.
Utility of drug-loaded fatty acid vesicles for transdermal delivery
Application of fatty acid vesicles for transdermal delivery of various therapeutic agents is shown in .
Figure 9. Applications of fatty acid vesicles for transdermal delivery. Various categories of drugs like antifungal, anticancer, antiosteoarthritic, and antiinflammatory drugs loaded in fatty acid vesicles have been investigated for transdermal delivery.
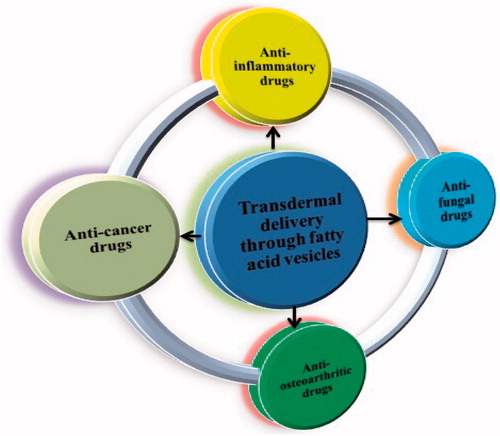
Transdermal delivery of antifungal drugs through fatty acid vesicles
Superficial fungal infections are becoming more prominent now-a-days especially due to their coupling with immunosuppressive drugs like AIDS (Zakir et al. Citation2010). Skin, nails, and eyes are the sites for superficial fungal infections (Agarwal and Katare Citation2002). They are less threatening compared with systemic fungal infections, but can be dangerous if not treated properly. Formulations like creams, powders, and gels are used to treat skin fungal infection, whether deep seated or on surfaces. Burning, redness, and swelling of skin are the side effects shown by these formulations. Since these formulations release drug molecules immediately, they can generate high impact allergy reactions due to stimulation of the immune system (López-Pinto et al. Citation2005). Invasive fungal infections like aspergillosis and candidiasis become more difficult to treat with these formulations because of less penetration power (Marly et al. Citation1998). To minimize these drawbacks of conventional formulations, novel formulations like niosomes, liposomes, ethosomes, microemulsions, nanoparticles, microspheres, and micelles have been explored for transdermal delivery of antifungal drugs (Kumar et al. Citation2014). Fatty acid vesicles are more advanced systems explored for the purpose. Zakir et al. (Citation2010) developed and characterized oleic acid vesicles of fluconazole for the transdermal delivery. Vesicles were prepared by a thin-film hydration method using oleic acid as a fatty acid principal component. Developed vesicles were characterized for size, size distribution, shape, in-vitro release, pH dependent and storage stability, skin irritation study, and ex-vivo skin permeation. The penetration behavior of vesicles was further evaluated and elucidated using confocal microscopic study. The vesicles possessed higher entrapment efficiency (44.11 ± 1.13%) with optimum vesicle size (527 ± 15 nm) and homogeneity in regard to size distribution (polydispersity index, i.e. PDI = 0.234 ± 0.016) at 7:3 oleic acid-to-fluconazole ratio. Vesicles were found stable in the refrigerated condition. Ex-vivo skin permeation and confocal microscopic studies suggested that oleic acid vesicles penetrate the stratum corneum upto 55–60 μm and retain the drug accumulated in the epidermal part of the skin. Later on Verma et al. (Citation2014) evaluated the therapeutic efficacy of clotrimazole-loaded oleic acid vesicles in the male guinea pigs transdermally. Oleic acid vesicles possessed high drug entrapment (49.5 ± 1.0%) and optimum size (455 ± 22 nm) along with good colloidal characteristics (polydispersity index = 0.210 ± 0.035 and zeta potential = −22.45 ± 0.25 mV) at 4:6 drug-to-oleic acid ratio. In-vitro drug release study showed sustained release of drug from the vesicular dispersion. In-vivo study confirmed prolonged release of drug from oleic acid vesicle up to five days, indicating its usefulness for long-term therapy compared with marketed formulation, namely, Candid-V Gel, Glenmark.
Transdermal delivery of anticancer drugs through fatty acid vesicles
Patients of skin cancer are increasing in European countries from last one decade (Sekulic et al. Citation2013). The most common type of skin cancer, basal cell carcinoma (BCC), is most widely observed in woman under age of 40 years (Wu and Stein Citation2013). US-FDA has approved 5-Fluorouracil (5-FU) for topical treatment of BCC (Love et al. Citation2009). It is used in the form of 5% cream (Efudex® cream) for treatment of BCC. This marketed formulation shows various side effects like itching, eczema, burning sensation, redness, and poor penetration in the skin (Chitwood et al. Citation2013). These side effects can be masked by encapsulating the drug inside the system like fatty acid vesicles. They can enhance the penetration of the drug and provide sustained release of drugs. Dhillon et al. (Citation2011) tried to encapsulate 5-Fluorouracil (5-FU) inside the oleic acid vesicles and evaluated them for localization of bioactive in the skin. Vesicles were prepared by a film hydration method using oleic acid as a fatty acid principal component. Developed vesicles were characterized for size, size distribution, shape, in-vitro release, pH dependent and storage stability, and skin and ex-vivo skin permeation. The vesicles possessed higher entrapment efficiency (64.0 ± 4.2%) with optimum vesicle size (632 ± 17 nm) and homogeneity in regard to size distribution (polydispersity index = 0.234 ± 0.016) at 7:3 oleic acid-to-5-FU ratio. In-vitro drug release study suggested sustained release of drug from the vesicles. The vesicles were fairly stable at refrigerated conditions. Ex-vivo skin permeation and Confocal microscopic studies suggested that oleic acid vesicles penetrated the stratum corneum and retained the drug accumulated in the epidermal part of the skin.
Transdermal delivery of antiinflammatory drugs through fatty acid vesicles
Chronic inflammatory diseases like rheumatoid arthritis (RA) have unknown etiology and complex multifactorial pathogenesis (Grassi et al. Citation1998). Preliminary treatment of RA involves the use of nonsteroidal antiinflammatory drug (NSAID). Currently, use of slow-acting disease modifying antirheumatic drugs (DMARDs) has been suggested for early treatment of RA because of their potential to prevent or reduce joint damage. Therefore, they are used early in the treatment of RA and usually no later than 3 months after the commencement of NSAID treatment (Ryan and Brooks Citation1999). Methotrexate (MTX) comes under the category of DMARDs used in the treatment of RA (Cronstein Citation1997). So, formulation and in-vitro evaluations of methotrexate (MTX)-loaded oleic acid vesicles were carried out by Sharma and Arora (Citation2012). The focus of this study was to localize MTX in the synovial joint to treat inflammation in RA. Developed vesicles were characterized for size, size distribution, shape, in-vitro release, pH dependent, and storage stability. Vesicular size, polydispersity index (PDI), and entrapment efficiency of optimized formulations were found 632 ± 17 nm, 0.262 ± 0.037, and 51.0 ± 4.2%, respectively. Interaction between MTX and oleic acid was investigated using differential scanning calorimetry. The MTX amount permeated through rat skin was three to 4-fold higher using oleic acid vesicles compared with those from plain drug solution or carbopol gel. At the end of the skin permeation assay it was found that using oleic acid vesicles, up to 50% of the administered dose was present in the skin. Thus, this system could be beneficial to reduce inflammation in RA. Furthermore, Mittal et al. (Citation2013) formulated oleic acid vesicular gel of dexamethasone and evaluated this gel using carrageenin-induced rat paw edema model for antiinflammatory activity. Formulation prepared with drug-to-oleic acid molar ratio 8:2 (size = 631 ± 15 nm, PDI = 0.534 ± 0.026, zeta potential= −6.8 ± 0.9 mV) produced the greatest number of vesicles and greater entrapment efficiency (65.2 ± 3.8%). The transdermal permeation was of vesicular gel found to be about 4.7 times higher than plain drug gel. Significant reduction of edema was observed in case of vesicular gel (0.5% w/w) compared with the same amount of commercial product (DecadronR cream, Merck) after 6 hours. So, oleic acid vesicles-based gel of dexamethasone could be more effective to treat inflammation compared with marketed gel.
Transdermal delivery of antiosteoarthritic drugs through fatty acid vesicles
Connective tissues and gastrointestinal mucosal membranes contain glucosamine acts as a natural component of glycoprotein found in them (Towheed et al. Citation2005). Collagen and proteoglycans synthesis in the human body is promoted by glucosamine supplementation. Both these components are vital for the joint rebuilding along with the formation of synovial fluid which lubricates the joints (Richy et al. Citation2003). So, use of glucosamine has been suggested in the treatment of osteoarthritis. Glucosamine is taken through oral route for treatment of osteoarthritis. Glucosamine has low oral bioavailability, short half life of 1 hour and very high-dosing frequency (500 mg thrice a day; Hughes and Carr Citation2002). Therefore, Sharma and Arora (Citation2013) prepared oleic acid vesicles of glucosamine sulfate and dispersed them in carbopol gel for topical delivery to treat osteoarthritis. Muscular concentration of the drug for vesicle-based gel on Albino rats (Sprauge Dawley) was 6-fold higher compared plain carbopol gel. Vesicular size, zeta potential, and entrapment efficiency of optimized formulations were found 632 ± 17 nm, −23.4 ± 0.7 mV, and 68.0 ± 4.2%, respectively. Carbopol gel loaded with oleic acid vesicles showed sustained release of glucosamine up to 15 days. So, this formulation could be considered as an effective depot formulation to treat osteoarthritis. represents a brief overview of research work done on fatty acid vesicles for transdermal delivery.
Table 2. Brief summary of research work done on fatty acid vesicles for transdermal delivery.
Clinical status and intellectual property rights related to fatty acid vesicles
Results of all research work carried out on fatty acid vesicles for transdermal delivery predict their efficacy and they should be evaluated clinically. But, currently no clinical data are available related to use of fatty acid vesicles for transdermal delivery. A few patents regarding the fatty acid vesicles are described in (http://www.freepatentsonline.com).
Table 3. Some patents related to fatty acid vesicles for drug delivery.
Problematic issues and future progress
Fatty acid vesicles can be explored as a suitable transdermal delivery system facilitating the bioactive molecules, to reach the lower layers of epidermis. However skin toxicity and low entrapment of hydrophilic drugs are main problematic issues regarding transdermal delivery of fatty acid vesicles. These issues should be encountered to enhance the efficacy of fatty acid vesicles (Mittal et al. Citation2013). This drug delivery system might be beneficial for skin diseases like fungal or bacterial, can act as a suitable delivery system for anesthetics, analgesics, and anticancer drugs. In the future clinical evaluation of fatty acid vesicles will govern their entrance into the market (Zakir et al. Citation2011).
Conclusions
Fatty acid vesicles show high therapeutic potential in the treatment of various skin disorders. They are capable of releasing the drugs in controlled or sustained fashion, thus minimizing the adverse effects of drugs like burning, itching, swelling, and other allergic reactions on the skin. In conditions like AIDS, fatty acid vesicles might be highly beneficial to treat the skin disorders because they do not activate the immune system due to controlled release of the drug. The chances of drug interactions with this delivery system are negligible which are more prominent in the case of oral drug used to treat skin disorders. Fatty acid vesicles are better alternative to liposomes for topical delivery because of their cheaper cost, high penetration power, and good entrapment efficiency. Although a clinical exploration of these systems is a challenge, still they can be considered as highly efficient and better nano-carriers for transdermal delivery of various bioactives.
Disclosure statement
The authors confirm that this article content has no conflicts of interest.
References
- Agarwal R, Katare OP. 2002. Preparation and in-vitro evaluation of miconazole nitrate loaded topical liposomes. Pharm Tech. 48–60. Available at: http://www.pharmtech.com/data/artclestandard/pharmecch/462002/38265/article.pdf).
- Ahmad N, Muhammad R, Tajuddin HA, Misran M. 2014. Effect of glycolipids on the stability and electrophoretic mobility of decanoic acid vesicles. Colloid Surf a Physicochem Eng Asp. 443:96–101.
- Apel CL, Deamer DW, Mautner MN. 2002. Self-assembled vesicles of monocarboxylic acids and alcohols: conditions for stability and for the encapsulation of biopolymers. Biochim Biophys Acta. 1559:1–9.
- Aungst BJ. 1989. Structure/effect studies of fatty acid isomers as skin penetration enhancers and skin irritants. Pharm Res. 6:244–247.
- Bandyopadhyay P, Johnson M. 2007. Fatty alcohols or fatty acids as niosomal hybrid carrier: effect on vesicle size, encapsulation efficiency and in vitro dye release. Colloids Surf B Biointerfaces. 58:68–71.
- Bouwstra JA, Honeywell-Nguyen PL. 2005. Vesicles as a tool for transdermal and dermal delivery. Drug Discov Today Technol Today. 2:67–74.
- Bouwstra JA, Ponec M. 2006. The skin barrier in healthy and diseased state. Biochim Biophys Acta. 1758:2080–2095.
- Chitwood K, Etzkorn J, Cohen G. 2013. Topical and intralesional treatment of nonmelanoma skin cancer: efficacy and cost comparisons. Dermatol Surg. 39:1306–1316.
- Cistola DP, Hamilton JA, Jackson D, Small DM. 1988. Ionization and phase behavior of fatty acids in water: application of the Gibbs phase rule. Biochemistry. 27:1881–1888.
- Cronstein BN. 1997. The mechanism of action of methotrexate. Rheum Dis Clin North Am. 23:739–755.
- Deamer DW, Lancet D, Segre D, Ban-Eli D. 2001. The lipid world. Orig Life Evol Biosph. 31:119–145.
- Dhillon V, Sharma S, Jain S, Sharma A, Arora S. 2011. Formulation characterization and evaluation of new topical 5-FU by drug entrapment in oleic acid vesicles. Am J Pharm Tech Res. 1:1–16.
- Edwards K, Silvender M, Karlson G. 1995. Aggregate structure in dilute dispersions of oleic acid/sodium oleate and oleic/sodium oleate/egg phophatidylcholine. Langmuir. 11:2429–2434.
- Ehdaie B. 2011. Enhanced Delivery of transdermal drugs through human skin with novel carriers. J Pharm Biomed Sci. 1:161–166.
- El Maghraby GM, Williams AC, Barry BW. 2001. Skin delivery of 5-fluorouracil from ultradeformable and standard liposomes in-vitro. J Pharm Pharmacol. 53:1069–1077.
- Elias PM. 1983. Epidermal lipids, barrier function and desquamation. J Invest Dermatol. 80:44–49.
- Elsayed MMA, Abdallah OY, Naggar VF, Khalafallah NM. 2007. Lipid vesicles for skin delivery of drugs: reviewing three decades of research. Int J Pharm. 332:1–16.
- Gebicki JK, Hicks M. 1973. Ufasomes are stable particles surrounded by unsaturated fatty acid membranes. Nature. 243:232–234.
- Grassi W, De Angelis R, Lamanna G, Cervini C. 1998. The clinical features of rheumatoid arthritis. Eur J Radiol. 27:S18–S24.
- Hanczyc MM, Fujikawa SM, Szostak JW. 2003. Experimental models of primitive cellular compartments: encapsulation, growth and division. Science. 302:618–622.
- Hargreaves WR, Deamer DW. 1978. Liposomes from ionic, single chain amphiphiles. Biochemistry. 17:3759–3768.
- Hughes R, Carr A. 2002. A randomized, double-blind, placebo-controlled trial of glucosamine sulphate as an analgesic in osteoarthritis of the knee. Rheumatology. 41:279–284.
- Knorr F, Lademann J, Patzelt A, et al. 2009. Follicular transport route-research progress and future perspectives. Eur J Pharm Biopharm. 71:173–180.
- Kong JF, Rabkin SW. 2002. Palmitate induced cardiac apoptosis is mediated through CPT-1 but not influenced by glucose and insulin. Am J Physiol Heart Circ Physiol. 282:H717–H725.
- Kumar L, Verma S, Bhardwaj A, Vaidya S, Vaidya B. 2014. Eradication of superficial fungal infections by conventional and novel approaches: a comprehensive review. Artif Cells Nanomed Biotechnol. 42:32–46.
- Lopes LB, Ferreira DA, de Paula D, Garcia MT, Thomazini JA, Fantini MC, Bentley MV. 2006. Reverse hexagonal phase nanodispersion of monoolein and oleic acid for topical delivery of peptides: in vitro and in vivo skin penetration of cyclosporin. Pharm Res. 1332–1342.
- López-Pinto JM, González-Rodríguez ML, Rabasco AM. 2005. Effect of cholesterol and ethanol on dermal delivery from DPPC liposomes. Int J Pharm. 298:1–12.
- Love WE, Bernhard JD, Bordeaux JS. 2009. Topical imiquimod or fluorouracil therapy for basal and squamous cell carcinoma: a systematic review. Arch Dermatol. 145:1431–1438.
- Luisi PL, Rasi S, Mavelli F. 2003. Cooperative micelle binding and matrix effect in oleate vesicle formation. J Phys Chem B. 107:14068–14076.
- Luisi PL, Walde P, Blocher M, Blochliger E. 1998. Matrix effect in the size distribution of fatty acid vesicles. J Phys Chem B. 102:10383–10390.
- Marly EMJ, Kuijk-Meuwissen V, Mougin L, Junginger HE, Bouwstra JA. 1998. Application of vesicles to rat skin in-vivo: a confocal laser scanning microscopy study. J Control Release. 56:189–196.
- Mittal R, Sharma A, Arora S. 2013. Ufasomes mediated cutaneous delivery of dexamethasone: formulation and evaluation of anti-inflammatory activity by carrageenin-induced rat paw edema model. J Pharm. 2013:680580.
- Moreira TS, de Sousa VP, Pierre MB. 2010. A novel transdermal delivery system for the anti-inflammatory lumiracoxib: influence of oleic acid on in-vitro percutaneous absorption and in vivo potential cutaneous irritation. AAPS Pharm Sci Tech. 11:621–629.
- Placensia L, Norlen L, Bagatoli LA. 2007. Direct visualization of lipid domains in human skinstratum corneum’s lipid membranes: effect of pH and temperature. Biophys J. 93:3142–3155.
- Pohl EE, Peterson U, San J, Pohl P. 2000. Changes of intrinsic membrane potentials induced by flip-flop of long chain fatty acids. Biochemistry. 39:1834–1839.
- Proksch E, Brandner JM, Jensen JM. 2008. The skin: an indispensable barrier. Exp Dermatol. 17:1063–1072.
- Rendón A, Carton DG, Sot J, García-Pacios M, Montes LR, Valle M, et al. 2012. Model systems of precursor cellular membranes: longchain alcohols stabilize spontaneously formed oleic acid vesicles. Biophys J. 102:278–286.
- Renoncourt A, Bauduin P, Nicholl E, Touraud D, Verbavatz JM, Dubois M. 2006. Spontaneous vesicle formation of an industrial single chain surfactant at acidic pH and at room temperature. Chemphyschem. 7:1892–1896.
- Richy F, Bruyere O, Ethgen O, Cucherat M, Henrotin Y, Reginster JY. 2003. Structural and symptomatic efficacy of glucosamine and chondroitin in knee osteoarthritis: a comprehensive meta-analysis. Arch Intern Med. 163:1514
- Robinson BH, Walde P, Rogerson ML, Bucakm S. 2006. Kinetic studies of the interaction of fatty acids with phosphatidylcholine vesicles (liposomes). Colloids Surf B Biointerfaces. 48:24–34.
- Ryan L, Brooks P. 1999. Disease-modifying antirheumatic drugs. Curr Opin Rheumatol. 11:161–166.
- Sekulic A, Mangold AR, Northfelt DW, LoRusso PM. 2013. Advanced basal cell carcinoma of the skin: targeting the hedgehog pathway. Curr Opin Oncol. 25:218–223.
- Sharma A, Arora S. 2012. Formulation and in-vitro evaluation of ufasomes for dermal administration of methotrexate. ISRN Pharm. 2012:873653.
- Sharma A, Arora S. 2013. Dermal delivery of glucosamine sulphate: formulation, characterization and performance evaluation. World J Pharm Pharm Sci. 2:6448–6462.
- Sinico C, Fadda AM. 2009. Vesicular carriers for dermal drug delivery. Expert Opin Drug Deliv. 6:813–825.
- Towheed TE, Maxwell L, Anastassiades TP, Shea B, Houpt J, Robinson V. 2005. Glucosamine therapy for treating osteoarthritis. Cochrane Database Syst Rev. 2: CD002946.
- Verma S, Bhardwaj A, Vij M, Bajpai P, Goutam N, Kumar L. 2014. Oleic acid vesicles: a new approach for topical delivery of antifungal agent. Artif Cells Nanomed Biotechnol. 42:95–101.
- Walde P, Morigaki K. 2007. Fatty acid vesicles. Curr Opin Colloid Interface Sci. 12:75–80.
- Wu TP, Stein JA. 2013. Nonmelanoma skin cancer in young women. J Drugs Dermatol. 12:568–572.
- Zakir F, Vaidya B, Goyal AK, Malik B, Vyas SP. 2010. Development and characterization of oleic acid vesicles for the topical delivery of fluconazole. Drug Deliv. 17:238–248.
- Zakir F, Vaidya B, Malik B, Vyas SP. 2011. Fatty acid vesicles: promising drift in colloidal drug carriers. In: Vyas SP, Murthy RSR, Narang RK, Eds. Nanocolloidal Carriers: Site Specific and Controlled Drug Delivery. New Delhi: CBS publishers. pp. 201–218.
- Zhang F, Kamp F, Hamilton JA. 1996. Dissociation of long and very long chain fatty acids from phospholipid bilayers. Biochemistry. 35:16055–16060.