Abstract
In the present study, we have investigated the enhanced synergistic and apoptotic activity of immunohybrid nanoparticles encapsulating oxaliplatin and covalently conjugated with TRAIL (Apo-2L/CD-253). Time-dependent cytotoxicity activity of nanoparticles was determined by MTT assay in HT-29 cells. Nuclear morphological changes and assessment of apoptotic ratio was analyzed by DAPI (4′6-diamidino-2-phenylindole) staining and annexin–propidium iodide (PI) assay. Cell-cycle analysis of oxaliplatin in HT-29 cell was analyzed by flow cytometry at 72 h. Furthermore, molecular mechanisms related to oxaliplatin-induced anticancer activity was explored by western blot analysis. Our study revealed appreciable time-dependent cytotoxicity, apoptotic, and synergistic activity of oxaliplatin immunohybrid nanoparticles
Introduction
Colorectal cancer comes along as the fourth leading cause of death among the cancers worldwide accounting for almost half a million deaths. Although various treatment strategies were available and followed, still they lack required therapeutic activity attributing to various limitations. Chemotherapy, surgery, and radiotherapy are the conventional approaches used for the treatment of cancer, out of which, chemotherapy is the widely employed approach. Chemotherapy for colorectal cancer has its share of limitations such as site in-specificity, improper biodistribution, side effects to healthy tissues (such as neurotoxicity, hemotological toxicity, alopecia and so forth,) that limits its clinical efficacy (Cassidy and Misset Citation2002). Folforinox (comprising oxaliplatin, 5-fluorouracil, and irinotecan) is the first line chemotherapy for the treatment of colorectal cancer (Tummala et al. Citation2015). These limitations of the mostly followed conventional treatment crave for the better, safer formulation with site specificity and minimizing the side effects to healthy tissues, thereby enhancing its clinical and therapeutic efficacy.
Going by the recent researches, nanoparticulate approach has been widely used to enhance the properties of active pharmaceutical ingredients (Jaiswal et al. Citation2014). Furthermore, nanoparticles have the ability to modify blood circulation and tissue distribution (Alexis et al. Citation2008, Bertrand and Leroux Citation2012, Hosseini et al. Citation2015) along with protecting the therapeutic agents (Whitehead et al. Citation2009). This property of nanoparticles acts as a boon in the field of oncology, as anticancer drugs exhibit wide range of toxicities. Additionally, ligand-mediated targeting (active targeting) has been employed to explore the enhanced interactions of the tumor cells with nanoparticles, thereby increasing internalization of the drugs without altering the overall distribution (Alexis et al. Citation2008, Byrne et al. Citation2008, Peer et al. Citation2007).
This active targeting approach benefits the API in targeting specifically to the tumor cells attributing to the selection of ligand (antibody) that is specific to a particular protein expressed in the tumors during colorectal cancer (Gupta et al. Citation2015). The ligand can be conjugated to the nanoparticle surface by either amine modification, sulfide modification, carbohydrate modification or may be noncovalent conjugation depending on the presence of functional groups of the nanoparticles and functionality of the antibody (Hashimoto et al. Citation1984, Heath & Martin Citation1986, Leserman et al. Citation1984, Torchilin Citation1984). Pacitaxel-loaded immunonanoparticles targeted for HER-2 protein had shown improved efficacy in treating disseminated ovarian cancers overexpressing HER-2 cells (Cirstoiu-Hapca et al. Citation2010).
Oxaliplatin (oxalato(transl-1,2-diaminocyclohexane) is a potent antitumor compound used for the first-line chemotherapy belonging to third-generation antineoplastics and is showing the above-mentioned limitations of chemotherapy (Tummala et al. Citation2015). Oxaliplatin, as potential it can be, but still lacks desirable therapeutic efficiency partly attributed to the side effects to healthy cells that minimizes the drug distribution to the target site (Krishnaiah and Satyanarayana Citation2001, Michor et al. Citation2005, Tummala et al. Citation2014, Tummala et al. Citation2014). CD-253 (tumor necrosis factor-related apoptosis-inducing ligand or Apo-2L or TRAIL) and TRAIL death receptor antibodies distinguished themselves evidently as successful biologically targeted antitumor proteins. It belongs to the tumor necrosis factor family that can induce apoptosis in only human cancer cells thereby excluding healthy cells (Holoch and Griffith Citation2009, Walczak et al. Citation1999). Generally, TRAIL overexpression during cancer correlates with multiplication of cells attributed to the interference for TRAIL-induced apoptosis.
The present study hypothesizes the site specificity of immunonanoparticles with enhanced efficacy and apoptotic activity. Lipid polymer hybrid nanoparticles can be considered as an ideal platform in this regard attributing to their stability, longer circulation time, controlling the release of the drugs. Moreover, chitosan, which was used as a polymer, is biocompatible, and hence the lipids are used for lipid film coating. This nanoformulation was hypothesized for better site specificity, apoptotic activity with increased anticancer activity. We have previously reported the oxaliplatin immunohybrid nanoparticles characterization along with its cellular uptake into HT-29 cells and MCF-7 cells. This study comes as a sequential to the former study to further investigate the apoptotic activity of the formulated oxaliplatin hybrid nanoparticles (OHPNPs) and oxaliplatin immunohybrid nanoparticles (OIHNPs). Cell-cycle analysis studies were conducted for these formulations for better analysis of cells DNA content during various phases by using flow cytometry. Cellular morphological changes in HT-29 cells were emphasized by conducting fluorescence studies using DAPI. Mechanistic basic studies using western blotting was performed for the evaluation of antiapoptotic and caspase-dependent protein expressions.
Materials and methods
Materials
Oxaliplatin was obtained as a free sample from Cipla Pharmaceuticals, Bangalore, India. 2-(4-Amidinophenyl)-6-indolecarbamidine dihydrochloride solution (DAPI) used for nuclear staining was purchased from Beyotime Institute of Biotechnology, Japan. Acridine orange, ethidium bromide, and MTT (3-(4,5-dimethylthiazolyl-2)-2, 5-di-phenyltetrazolium bromide) were purchased from Sigma Aldrich, Mumbai, India. Annexin V-FITC plus propidium iodide (PI) double-staining kit was purchased from Invitrogen (Carlsbad, CA). All cell culture medium and human colorectal cancer cell line (HT-29) were procured from the National institute of health sciences (NIH), India. Analytical grade reagents were purchased from Sigma-Aldrich, Mumbai. All the samples were prepared using de-ionized Milli Q water.
Preparation of oxaliplatin immunohybrid nanoparticles
Oxaliplatin immunonanoparticles were already prepared and characterized for their apoptotic activity in our earlier studies (Tummala et al. Citation2015). Briefly, oxaliplatin polymeric nanoparticles were prepared using chitosan as polymer by solvent emulsification evaporation method. Cholesterol, soyalecithin, 1,2-distearoyl-sn-glycero-3-phosphoethanolamine-N-[maleimide(polyethylene glycol)-3400] (DSPE-PEG-3400-mal) and 1,2-distearoyl-sn-glycero-3-phosphoethanolamine-N-[methoxy (polyethylene glycol)-2000] (DSPE-PEG-2000) were selected in a ratio of 15:50:3:15 as lipids for the preparation of lipid film that can be used for coating. Thin lipid film was formed using rotary vacuum evaporator in a round-bottom flask at reduced temperatures. Briefly, polymeric emulsion was then added to the lipid film by ultrasound method that ensures the coating of the lipid film around the polymeric nanoparticles leading to the formation of oxaliplatin hybrid nanoparticles (OHNPs).
Briefly, 600 μg of thiolated anti-CD253 (using Traut’s reagent) and OHNPs micelle solution containing DSPE–PEG–MAL (15 mg/mL) were kept at 4 °C incubated overnight. The mixture was further then neutralized with 1 NaOH to pH 7.5 and kept aside from one day followed by centrifugation them for 15 min at 15,000 rpm and washing them thrice with distilled water before collecting oxaliplatin immunohybrid nanoparticles (OIHNPs). The thiolated antibody used was already characterized for its thiol group at 3000 cm−1 in FTIR Spectrophotometer (FTIR 8400 S, Shimadzu).
Cell culture
CD-253 receptor-positive human colorectal adenocarcinoma cell line (HT-29) was grown in RPMI-1640 medium as monolayer cultures supplemented with 10% FBS under standard cell culture conditions of 37 °C in humidified 5% carbon dioxide (CO2) atmosphere. CD-253 receptor-negative human breast cancer cell line (MCF-7) was grown in Eagle’s medium as monolayer cultures supplemented with 10% FBS under standard cell culture conditions of 37 °C in humidified 5% CO2.
Time-dependent effect on nanoparticles on cell viability
Cell viability assay was carried out to determine the cell viability of hybrid nanoparticles and immunonanoparticles on HT-29 cell growth. Briefly, cells were seeded in six-well plates at a density of 1 × 106 cells/well and were left undisturbed for 24 h to ensure proper attachment. Oxaliplatin, OHPNPs, OIHNPs were added at different concentrations to the well plates by replacing the culture medium. Cell viability was observed further at different time points till 72 h (24, 48, and 72 h). Cells treated with DMSO were taken as a negative control. About 5 mg/mL of MTT in phosphate buffer saline (10 μL) was added at fixed time intervals that were followed by incubating them for 4 h and culture solution was removed. Formazan crystals were then dissolved properly by addition of DMSO (100 μL) to each well separately while shaking the plates vigorously. Experiments were conducted in triplicate in parallel. Each well absorbance was determined at wavelength of 570 nm on a microplate reader (Thermo scientific, Pittsburgh, PA). IC50 of the samples were further calculated by SPSS software (Version 19.0).
DAPI staining
Cell morphology can be visualized by DAPI staining. Briefly, HT-29 cells were seeded in six-well plates at a density of 1 × 106 cells/well. Cells were kept intact as such for one day followed by exposure to oxaliplatin, OHNPs, and OIHNPs containing media. All the formulations were used at their respective IC50 concentrations. Cells, which were treated with DMSO, and cells left untreated were considered as negative controls. Cells were left undisturbed for 24 h followed by the addition of acetic acid in methanol with ratio of 1:3 v/v and then stained with DAPI (Sigma-Aldrich, Mumbai, India). Fluorescence microscope (Olympus IX51, USA) was then used to visualize the stained cells morphology with a 430–490-nm filter (n = 6) (Chuah et al. Citation2014).
Annexin–PI studies
Annexin–PI studies were performed for immuno and uncojugated nanoparticles to assess the apoptotic ratio using an annexin V-fluorescein isothiocynate (FITC), primarily an apoptosis detection kit. Various characteristics, such as nuclear fragmentation, condensation of chromatin, decreased cell size, majorly due to the endonuclease cleavage of DNA are highly associated with apoptosis. Propidium iodide (PI) is a fluorescent dye (red color) that can enter apoptotic cells, whereas annexin is a phospholipid protein having appreciable affinity toward phosphatidylsterine (PS) in the early apoptotic stage. Briefly, HT-29 cells at a density of 1 × 105 cells/well were plated in six-well plates and were left undisturbed for whole night to ensure proper attachment. Cells were treated precisely with IC50 concentrations of OIHNPs, OHNPs, and oxaliplatin, respectively. Cells were harvested and washed thrice with phosphate buffer before suspending them in 500 μL of 1x binding buffer followed by proper staining with 5 of annexin V-FITC and 10 μL of PI. The stained cells were left incubated for 45 min at room temperature. Differentiation of stained cells can be done by visualizing the fluorescence and then categorizing them into the respective sections. The categories comprise of viable, necrotic, early apoptotic, and late apoptotic cells and can be analyzed by FACscan flow cytometer (Beckton Dickinson). Degree of apoptosis was quantified by determining the percentage of annexin V-positive cells. Control cells were maintained aside separately for a time span of 48 h.
AO/EB assay
Additionally, apoptosis was further quantified by acridine orange (AO)/ethidium bromide (EB) studies for 72 h. Drug and formulations (OIHNPs and OHNPs) were used at IC50 concentrations for precise apoptotic quantification. Briefly, HT-29 cells were seeded in 35-mm culture plate and were left placid for 24 h prior to addition of all the samples. Cells were then thoroughly washed with PBS followed by staining them in combination of 5 mg/mL of EB and 50 mg/mL of AO for 15 min at room temperature. Cells at different stages were determined by inverted fluorescence microscope (DMI 4,000B; Leica Microsystems) at a magnification of 20×. Control cells were kept aside separately till 72 h that helps in further identifying the color changes. Cells with different colors observed were grouped as green (viable cells), bright green (preliminary phase of apoptosis), light orange (early apoptotic cells) and bright red (late apoptotic cells) (Nair et al. Citation2011).
Cell-cycle analysis
Cells (HT-29) were seeded in six-well plates at a density of 1 × 105 cells/well followed by incubation and treating them with IC50 concentrations of OIHNPs and OHNPs. Later, cells were trypsinized and washed with PBS prior to fixing with 70% ethanol. Then, the cells were incubated with 25 μg/mL of PI and 200 mg/mL of Rnase A for 45 min. Controls were also seeded separately and kept aside. IC50 concentration of the samples was analyzed for 24 h and 48 h by FACScan flow cytometer (Becton Dickinson, San Jose, CA). Stained cells were analyzed by flow cytometry cell sorter (Becton Dickinson, San Jose, CA). Studies were conducted in triplicate to minimize the errors (Zhang et al. Citation2012).
Western blotting
Whole cell lysates were prepared for western blot analysis using RIPA buffer (comprising 20 mM NaH2PO4, 50 mM NaF, 2 mM EDTA, 150 mM NaCl, 1% deoxycholic acid, 0.1% SDS, pH 7.2). Western blot analysis was performed in the presence of a protease inhibitor cocktail. About 40 μg of protein was precisely taken followed by resolving it using 10% sodium dodecyl sulf-polyacrylamide gel electrophoresis (SDS-PAGE). Antisurvivin, anticaspase-3, anti-Bcl-2, anti-Bax (Santa Cruz Biotechnology, Dallas, TX) were selected as antibodies and horseradish peroxidase-conjugated goat antirabbit IgG (Pierce Biotechnology, Rockford, IL) was selected as secondary antibody followed by application and detection using chemiluminescence agents. Quantitative protein expression (relative) was analyzed using Hitachi Gene tools CCD-BIO software with Hitachi scanning densitometry (Lin et al. Citation2005).
Results and discussion
The characterization of OIHNPs and OHPNPs were carried out and reported in our previous article. OHPNPs have an average particle size of 119 ± 0.10 nm measured by dynamic light scattering (DLS), polydispersity value less than 0.2. OIHNPs have an average particle size of 181 ± 0.01 nm measured by DLS with negative zeta potential attributing to the stability of the lipid polymer hybrid nanoparticles in serum. OIHNPs entrapment efficiency was determined to be 67 ± 0.09%. In vitro drug release for OIHNPs was carried out by dialysis bag method that showed initial burst release followed by sustained release of oxaliplatin (81 ± 0.02%) over a period of 48 h. The presence of conjugated antibody to the hybrid nanoparticles have assisted the formulation in delivering oxaliplatin specifically in more amount that was evident by the cellular uptake studies in HT-29 and MCF-7 cells in our previous studies. Synergistic activity of TRAIL (CD-253) antibody was also proven by the enhanced cytotoxicity and decreased viability of cells by MTT assay comparable to OHPNPs, oxaliplatin, and untreated control. Both conjugated and unconjugated nanoparticles have been evaluated for their change in mitochondria potential and were observed rather significant change in the membrane potential for OIHNPs. In the present study, extensive cellular and apoptotic studies were carried out to investigate the enhanced apoptotic activity of the OIHNPs using annexin-PI assay (using flow cytometry) and acridine-orange/ethidium bromide method. Anticancer activity of the formulations were evaluated for different time intervals till 72 h by MTT assay. DAPI studies were conducted to determine the apoptosis by morphological changes in the nucleus by using fluorescence microscope. Cell-cycle analysis was also carried out to investigate the impact of free oxaliplatin, OHPNPs, and OIHNPs on the cell cycle of HT-29 cells. Western blotting studies were also carried out to inspect the effect of the formulations on the various proteins (apoptotic and antiapoptotic), thereby helps in evaluating the mechanism of its activity in inducing apoptosis and reversing antiapoptotic mechanisms.
Time-dependent effect of OIHNPs and OHPNPs on cell vaibility
These formulated nanoparticles were evaluated for their effect on cell viability and reported in our previous article. In the present study, percentage cell viability was determined in a time-dependent manner for 3 days. Viability of the cells at 24 h, 48 h, and 72 h were determined and can be observed in . It can be clearly observed that both nanoparticles had shown decrease in cell viability with increase in the concentration comparable to oxaliplatin. Additionally, much significant differences was not observed at 24 h (day 1) and 48 h (day 2) treatments between OHPNPs and OIHNPs for all doses with oxaliplatin. However, significant differences in cell viability were observed at 72 h (day 3) comparable to oxaliplatin by clear observation of the . Percentage cell viability of oxaliplatin, OHNPs, and OIHNPs was found to be 82–35%, 74–25%, and 62–11% at 3 day. Anticancer activity of the oxaliplatin was found to be unaltered for 24 h, as significant differences were not observed between the oxaliplatin and nanoparticles. However, anticancer activity of OHPNPs and OIHNPs was found to be increased drastically enhancing the delivery of oxaliplatin through various strategies on second and third day. IC50 values of OIHNPs was found to be 2.8 μM that was equivalent to 0.79 μM of oxaliplatin comparable to oxaliplatin pure drug equivalent to 3.6 μM justifying the decrease in IC50 value. Further studies were performed by considering these concentrations for appropriate results.
Morphological changes of cells by DAPI studies
Cells were observed microscopically to determine the morphological changes during apoptosis such as nuclear fragmentation and irregular edges. Significant changes were observed for oxaliplatin, OHNPs, and OIHNPs that can be concluded by the nuclear fragmentation and irregular edges (). Moreover, negative controls (DMSO and untreated) cells did not show any signs of apoptosis that further more justifies the importance of oxaliplatin and formulated nanoparticles. Oxaliplatin has shown appreciable morphological changes, but minimal when compared with the hybrid and immunohybrid nanoparticles at their IC50 concentrations. Nuclear morphological changes evaluated using DAPI further justifies the better apoptotic activity of immunonanoparticles that can be attributed to the major number of cells with nuclear morphological changes ().
Annexin–PI studies
Apoptosis ratio was quantified for different formulations by flow cytometry that can be observed form . Cells that were quantified to different compartments with different percentages were grouped as lower left (LL) representing viable cells, upper left (UL) representing necrotic cells, lower right (LR) representing late apoptotic cells and upper right (UR) representing early apoptotic cells. Annexin V-FITC, having affinity for PS binds to it appreciably, whereas PI stains the apoptotic cells that help us in determining the apoptotic percentage. Cells were grouped into categories depending upon the apoptotic ratio as viable cells (annexin V-FITC and PI negative), early apoptotic cells (annexin V-FITC and PI negative) and late apoptotic cells (annexin V-FITC and PI positive). Control was observed in HT-29 cells that exhibited 91.2% of viable cells, thereby helping us in moving for further studies with the formulations. Oxaliplatin (pure drug) had shown 51.98% of cells in LL and 31.2% of cells in UR quadrants. OIHNPs showed significant apoptotic activity that can be evident from the 35.2% of early apoptotic cells and 49.52% of late apoptotic cells in the UR and LR quadrants, respectively. OHNPs showed good apoptotic activity, but not as appreciable comparable to conjugated nanoparticles, as can be evident from the more 30% of viable cells in the LL quadrant contrary to 9% for OIHNPs. Moreover, both nanoparticles had shown comparable enhanced activity with oxaliplatin that can be observed in . Annexin–PI studies had shown appreciable activity to the unconjugated nanoparticles also attributed to the delivery of the drug through enhanced permeation and retention effect, whereas receptor-mediated endocytosis in case of OIHNPs.
AO/EB studies
Fluorescence study was conducted over a period of 72 h for the effective determination of apoptotic activty of the samples with time. OIHNPs had shown significant apoptotic activity (71% at 72 h) comparable to oxaliplatin that can be observed in . Enhancement in the apoptotic activity was observed with increase in the time for immunohybrid nanoparticles in HT-29 cells, which was confirmed by the presence of numerous cells in red color (late apoptotic) and light orange color (early apoptotic) compared with green color (viable) cells. Moreover, oxaliplatin alone had shown apoptotic activity (43% at 72 h), but to a limited extent that can be evident from the numerous cells exhibiting green fluorescence along with minimal number of cells with red and light orange at the end of 72 h. OHNPs had shown a good antiapoptotic activity (56% at 72 h), still it lacks site specificity and synergistic activity of anti-TRAIL protein that differentiates the apoptotic activity of immunohybrid nanoparticles from them. Meanwhile, control cells clearly conform the untreated cells with numerous cells showing only green fluorescence (indicating viable cells) that can be attributed to the fact that more than 90% of the cells were selectively taken by AO, whereas EB only stains apoptotic cells, thereby affirming characteristic chromatin condensation. Cytochemical staining of all formulations along with control can be clearly observed in .
Figure 4. Fluorescent microscopic images of HT-29 colon cancer lines with immunonanoparticles, OHNPs, and pure drug (oxaliplatin) at 24 h and 72 h. Control was visualized by green fluorescence at all time intervals conforming its viability. Oxaliplatin had shown minimal fluorescence of light orange and green representing minimal apoptosis. OIHNPs had shown appreciable apoptosis attributing to the major part of the cells with red fluorescence.
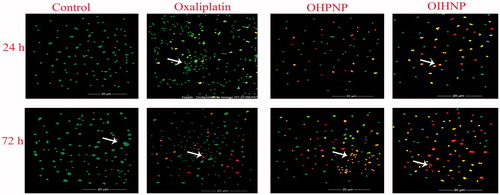
Cell-cycle arrest studies by flow cytometry
Cell-cycle analysis was carried out to explore the effects of oxaliplatin, OHNPs, and OIHNPs on HT-29 cell proliferation. Cell DNA contents were analyzed by flow cytometry to determine the distribution of HT-29 cells in various phases. Results were displayed as cellular DNA content frequency histograms. Furthermore, flow cytometry analysis was performed to determine any modifications in the cell cycle. Oxaliplatin immunonanoparticles when given at IC50 concentrations had shown a transitional S-phase delay (18.2%) followed by decreased G2/M phase at 24 h. Further, postincubation till 72 h had shown increased number of cells at G2/M phase (32.8%) rather than in S-phase (29.15%). Additionally, control cells had shown major number of cells in G0/G1 phase (72.13%) rather than in S-phase (18.2%) and G2/M phase (10.8%). OHNPs also had shown decreased number of cells in G0/G1 phase and increased number of cells in S-phase at 24 h that was followed by correlating the number of cells with G2/M phase (20.5%) at 72 h. Percentages of the cells in different phases can be observed in .
Figure 5. Cell-cycle analysis of HT-29 cells after 72 h of treatment with oxaliplatin, TRAIL, OHNPs, and OIHNPs. Nanoparticles had shown transition S-phase delay with cell-cycle arrest at G2 phase.
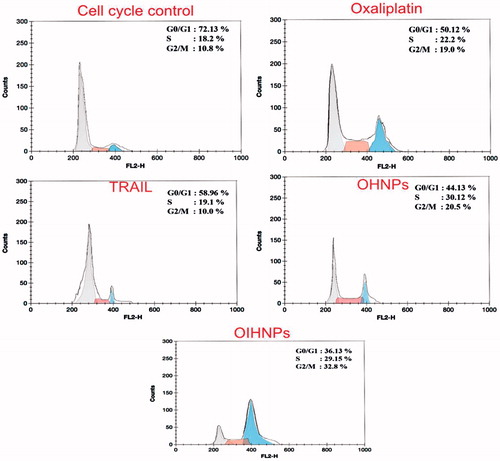
Postincubation studies were carried out to determine whether the inhibition of growth was cell-cycle phase specific and to determine the fate of the cells after the G2 phase. Generally, cell-cycle arrest in G2 phase prevents cells with damaged DNA from entering the next phase. Likewise, M phase blocks the trapped damaged cells that were escaped from the G2 phase (Smits and Medema Citation2001). Cells can be subjected to various ways after G2/M-phase depending upon their fate that includes apoptosis, permanent arrest (prolonged), and recovery after repair of DNA damage. Additionally, cell-cycle arrest in the G2 phase indicates survival and is an index of cytotoxicity. OIHNPs cytometry results comparable to OHNPs justifies the major number of cells in transitional S-phase at 24 h followed by G2/M cell-cycle arrest attributed to the apoptotic activity of antibody conjugated to the nanoparticles enhancing its activity than the unconjugated nanoparticles.
Western blotting studies
Before going in depth of the protein expression, oxaliplatin acts by forming intra- and interstrand crosslinking in DNA, which further prevents DNA replication and transcription, thereby causing cell death. So, various proteins were selected for the quantification of their protein expression by chemiluminescence reagents for all the samples. Furthermore, it also helps in evaluating the mechanism behind protein expression changes during cancer. Protein expression can be observed clearly for IC50 dose of all formulations in . OHNPs had shown significant increase in expression of caspase-3 and caspase-9 and decreased protein expression of survivin and Bcl-2. The reason behind the expression can be attributed to the mechanistic support of survivin inhibiting pro-apoptotic proteins caspase-3 and caspase-9 during cancer that caused inhibition of apoptosis. This justifies the fact that oxaliplatin inhibition of survivin that causes its decreased expression (Altieri Citation2003, Fujie et al. Citation2005)
Figure 6. Caspase-mediated apoptosis induced by oxaliplatin hybrid nanoparticles in comparison with oxaliplatin-treated HT-29 cells confirmed by western blot analysis of pro-apoptotic and antiapoptotic proteins.
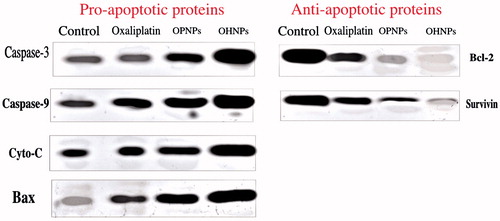
OHNPs and OPNPs had shown appreciable apoptotic activity, as can be observed from the decreased protein expression of bcl-2, which is primarily an antiapoptotic protein (Kirkin et al. Citation2004). But OHNPs had shown even appreciable antiapoptotic activity compared with OPNPs that conforms the appreciable properties of hybrid nanoparticles by incorporating both the advantages of polymeric nanoparticles and liposomes. In conclusion, nanoparticles had shown increased protein expression for caspase-3, caspase-9, Bax, and decreased protein expression for survivin and Bcl-2.
Conclusion
In summary, immunohybrid nanoparticles had shown enhanced apoptotic activity comparable to oxaliplatin and unconjugated nanoparticles. Cytotoxicity activity of the prepared nanoparticles were evaluated in our previous study, still its synergistic approach that helped in attaining enhanced antitumor activity needs to be highlighted. Time-dependent decrease in cell viability for immunonanoparticles comparable to unconjugated paves the preliminary method of confirmation of synergistic activity. Furthermore, fluorescence studies of the nucleus had proven the apoptotic phase of the nuclei stained with DAPI that was significant for immunonanoparticles. AO/EB and annexin–PI studies supported the increased antitumor activity of the prepared formulation by more number of cells in early apoptotic and late apoptotic phase. Cell-cycle analysis suggested the oxaliplatin arrest at G2/M phase with transitional S-phase delay at the end of 48 h. Western blotting studies helps us in analyzing the pathway behind oxaliplatin apoptotic activity by evaluating protein expression of pro-apoptotic and antiapoptotic proteins. It is concluded that nanoparticles prepared by this method using the same optimized ratios can be represented as potential drug delivery approach for effective delivery of the active pharmaceutical ingredient with synergistic activity for the better treatment of colorectal cancer.
Acknowledgements
Authors are thankful to Dr. M. N. Satish Kumar for his continuous support in guiding us throughout the work.
Disclosure statement
The authors report no conflicts of interest. The authors alone are responsible for the content and writing of this article.
References
- Alexis F, Pridgen E, Molnar LK, Farokhzad OC. 2008. Factors affecting the clearance and biodistribution of polymeric nanoparticles. Mol Pharm. 5:505–515.
- Altieri DC. 2003. Validating survivin as a cancer therapeutic target. Nat Rev Cancer. 3:46–54.
- Bertrand N, Leroux JC. 2012. The journey of a drug-carrier in the body: an anatomo-physiological perspective. J Control Release. 161:152–163.
- Byrne JD, Betancourt T, Brannon-Peppas L. 2008. Active targeting schemes for nanoparticle systems in cancer therapeutics. Adv Drug Deliv Rev. 60:1615–1626.
- Cassidy J, Misset JL. 2002. Oxaliplatin-related side effects: characteristics and management. Semin Oncol. 29:11–20.
- Chuah LH, Roberts CJ, Billa N, Abdullah S, Rosli R. 2014. Cellular uptake and anticancer effects of mucoadhesive curcumin-containing chitosan nanoparticles. Colloids Surf B. 116:228–236.
- Cirstoiu-Hapca A, Buchegger F, Lange N, Bossy L, Gurny R, Delie F. 2010. Benefit of anti-HER2-coated paclitaxel-loaded immuno-nanoparticles in the treatment of disseminated ovarian cancer: therapeutic efficacy and biodistribution in mice. J Control Release. 144:324–331.
- Fujie Y, Yamamoto H, Ngan CY, Takagi A, Hayashi T, Suzuki R, et al. 2005. Oxaliplatin, a potent inhibitor of survivin, enhances paclitaxel-induced apoptosis and mitotic catastrophe in colon cancer cells. JpnJ Clin Oncol. 35:453–463.
- Gupta A, Kaur CD, Saraf S, Saraf S. 2015. Formulation, characterization, and evaluation of ligand-conjugated biodegradable quercetin nanoparticles for active targeting. Artif Cells Nanomed Biotechnol. [Epub ahead of print]. doi: 10.3109/21691401.2015.1008503.
- Hashimoto Y, Endoh H, Sugawara M. 1984. Chemical methods for the modification of liposomes with proteins or antibodies. Liposome Technol. 3:41–50.
- Heath TD, Martin FJ. 1986. The development and application of protein-liposome conjugation techniques. Chem Phys Lipids. 40:347–358.
- Holoch PA, Griffith TS. 2009. TNF-related apoptosis-inducing ligand (TRAIL): a new path to anti-cancer therapies. Eur J Pharmacol. 625:63–72.
- Hosseini M, Haji-Fatahaliha M, Jadidi-Niaragh F, Majidi J, Yousefi M. 2015. The use of nanoparticles as a promising therapeutic approach in cancer immunotherapy. Artif Cells Nanomed Biotechnol. [Epub ahead of print]. doi: 10.3109/21691401.2014.998830.
- Jaiswal P, Gidwani B, Vyas A. 2014. Nanostructured lipid carriers and their current application in targeted drug delivery. Artif Cells Nanomed Biotechnol. 44:27–40.
- Kirkin V, Joos S, Zörnig M. 2004. The role of Bcl-2 family members in tumorigenesis. BBA-Mol Cell Res. 1644:229–249.
- Krishnaiah Y, Satyanarayana S. 2001. Colon-specific drug delivery systems. Advances in controlled and novel drug delivery. New Delhi, India: CBS Publishers and Distributors. pp. 89–119.
- Leserman LD, Machy P, Barbet J. 1984. Covalent coupling of monoclonal antibodies and protein A to liposomes: specific interaction with cells in vitro and in vivo. Liposome Technol. 3:29–40.
- Lin PH, Zui P, Zheng L, Na L, Danielpour D, Jie J. 2005. Overexpression of Bax sensitizes prostate cancer cells to TGF-beta induced apoptosis. Cell Res. 15:160–166.
- Michor F, Iwasa Y, Lengauer C, Nowak MA. 2005. Dynamics of colorectal cancer. Semin Cancer Bio. 15:484–493.
- Nair KL, Jagadeeshan S, Nair SA, Kumar GS. 2011. Biological evaluation of 5-fluorouracil nanoparticles for cancer chemotherapy and its dependence on the carrier, PLGA. Int J Nanomed. 6:1685–1697.
- Peer D, Karp JM, Hong S, Farokhzad OC, Margalit R, Langer R. 2007. Nanocarriers as an emerging platform for cancer therapy. Nat Nanotechnol. 2:751–760.
- Smits VA, Medema RH. 2001. Checking out the G(2)/M transition. 2M transition. BBA Gene Struct Expr. 1519:1–12.
- Torchilin VP. 1984. Immobilization of specific proteins on liposome surface: systems for drug targeting. Liposome Technol. 3:75–94.
- Tummala S, Gowthamarajan K, Satish Kumar M, Wadhwani A. 2015. Oxaliplatin immuno hybrid nanoparticles for active targeting: an approach for enhanced apoptotic activity and drug delivery to colorectal tumors. Drug Deliv. [Epub ahead of print]. doi: 10.3109/10717544.2015.1084400.
- Tummala S, Kumar MS, Gowthamarajan K, Prakash A, Rama K, Raju S, Mulukutla S. 2014. Preparation, physicochemical characterization and in vitro evaluation of oxaliplatin solid lipid nanoparticles for the treatment of colorectal cancer. Indo Amer J Pharm Res. 4:3579–3587.
- Tummala S, Kumar MS, Prakash A. 2014. Formulation and characterization of 5-Fluorouracil enteric coated nanoparticles for sustained and localized release in treating colorectal cancer. Saudi Pharm J. 23:308–314.
- Tummala S, Gowthamarajan K, Satish Kumar M, Praveen TK, Yamjala K, Tripuraneni NS, Prakash A. 2015. Formulation and optimization of oxaliplatin immun-nanoparticles using Box–Behnken design and cytotoxicity assessment for synergistic and receptor mediated targeting in the treatment of colorectal cancer. Artif Cells Nanomed Biotechnol. [Epub ahead of print]. doi: 10.3109/21691401.2015.1111226.
- Walczak H, Miller RE, Ariail K, Gliniak B, Griffith TS, Kubin M, et al. 1999. Tumoricidal activity of tumor necrosis factor–related apoptosis–inducing ligand in vivo. Nat Med. 5:157–163.
- Whitehead KA, Langer R, Anderson DG. 2009. Knocking down barriers: advances in siRNA delivery. Nat Rev Drug Discov. 8:129–138.
- Zhang XL, Hu AB, Cui SZ, Wei HB. 2012. Thermotherapy enhances oxaliplatin-induced cytotoxicity in human colon carcinoma cells. World J Gastroenterol. 18:646–653.