Abstract
In order to increase stabilities and controlled/sustained released of T4 phages were encapsulated within alginate beads which were then coated with chitosan, polyethylene imine (PEI). Quite high loading capacities (over 90%) were achieved in these pH-sensitive microbeads. Coating with those polycations increased significantly stability both in “simulated gastric fluid” and bile salts especially in the case of PEI coating. The tests conducted in “simulated intestinal fluid” demonstrated that phages were released from the beads which were active at basic pH in which the release rates were smaller in case of chitosan. PEI concluded to be a better coating then chitosan.
Introduction
The number of antibiotic-resistant bacteria is increasing rapidly at global level especially in the developing countries, therefore alternative therapeutic approaches to replace antibiotics is becoming one of the urgent-most important issues/priorities in public health – worldwide (World Health Organization Citation2012). According to the statistical data, millions of people are infected each year by pathogenic bacteria including Escherichia coli Salmonella, Staphylococcus, Campylobacter, Listeria.
Bacteriophages are considered as an important alternative to antibiotics (Joerger Citation2003, Housby and Mann Citation2009, Soothill et al. Citation2004, Weber-Dabrowska et al. Citation2003). A number of different phage preparations have been developed and commercialized as pharmaceutical formulations in Eastern Europe (mainly Russia and Georgia) (Sulakvelidze et al. Citation2001, Sulakvelidze and Kutter Citation2005). They have been used not only for human but also for the treatment of animals (like calves and lambs) (Bach et al. Citation2009, Smith and Huggins Citation1983, Sulakvelidze and Barrow Citation2005) and plants (such as tomato, spinach, broccoli, etc.) (Abuladze et al. Citation2008).
One of the main concerns in phage therapy using oral pharmaceutical formulations (usually emulsion of phages) is the loss of “antibacterial activity” during the passage via gastrointestinal (GI) tract in which they faced to quite low pH in stomach and destructive enzymes, bile, etc. (Chibani-Chennoufi et al. Citation2004, Joerger Citation2003, Ma et al. Citation2008, Smith et al. Citation1987, Wang and Sabour Citation2010). Therefore, an oral delivery system – “sustained release” – is needed to carry/protect phages from the harsh stomach conditions and release in the intestine safely/actively which was also the main rational of this study.
Alginate is a bio-based polysaccharide extracted from algae – has been one of the most widely studied biopolymer for encapsulation of bioactive agents by different rather simple techniques for the GI-sustained release formulations (Cook et al. Citation2012, Heidebach et al. Citation2012, Puapermpoonsiri et al. Citation2009). Alginate gels – cross-linked networks – exhibit a gel phase transition at around pH 2.5–3 means that they do collapse (shrinks) at values lower than this pH but get rapidly swollen in higher pH values – which is very typical and make them very suitable encapsulation material for the GI tract delivery systems. In addition, they are highly biocompatible, biodegradable having almost no any side effects – no toxicity (George and Abraham Citation2006, Lee and Heo Citation2000, Li et al. Citation2007, Wee and Gombotz Citation1998). However, stability of alginate gel beads at low pH values is not perfect (Mortazavian et al. Citation2008). In order to further stabilization – increase the stability of alginate networks, two major strategy applied: (i) blending with other natural polymers or materials such as starch, whey protein, glycerol, etc. (Hansen et al. Citation2002, Rajam et al. Citation2012, Sultana et al. Citation2000); (ii) coating with a second upper layer – as also applied in this present study (Burgain et al. Citation2011, Krasaekoopt et al. Citation2004).
Alginate is a polyanion – negatively charged polysaccharide – it easily interacts with a polycation and form a rather strong polyelectrolyte complex – a polyelectrolyte capsule simply an alginate solution is added into a solution – drop wise – containing a mixture of calcium chloride and chitosan (Overgaard et al. Citation1991) or alginate beads performed by cross-linking with calcium ions are soaked in a chitosan solution for further coating (McKnight et al. Citation1988, Murata et al. Citation1993). Recently, coating with chitosan (Ma et al. Citation2008), or poly-L-lysine (Cui et al. Citation2000), loading with buffering materials such as calcium carbonate have been reported to provide improved stability of phages (Ma et al. Citation2012).
In this study, as schematically described in , T4 phages were first encapsulated within alginate beads by following the classical technique – dropping the phage emulsions into a CaCl2 bath for cross-linking of alginate network with calcium cations then coated with two alternative polycations, chitosan (similar approach given in the related literature) and branched polyethylene imine (PEI), that is widely used in preparation of nonviral vectors (carrier of plasmid DNA) in transfection studies (Pişkin et al. Citation2004), and it is used for the first time for phage encapsulation formulations to form polyelectrolyte complex with alginate. Here we demonstrate the effects of chitosan and PEI on the stability and release of T4 phage in simulated body fluid – simulating GI tract.
Experimental
Escherichia coli (E. coli K12) and its specific bacteriophages (T4) were obtained from ATCC (11303 and 11303-B4, respectively). The Luria-Bertani (LB) liquid medium was prepared by dissolving 25 g of LB powder into 1 L of distilled water and this non-pathogenic strain was cultured in the LB medium at 37 °C in a rotary shaker (200 rpm) until reaching the exponential growth phase with an optical density at 600 nm (OD600) 0.2–0.5. They were centrifuged at 6000 rpm for about 5 min and the pellets obtained were washed a few times and re-suspended in phosphate-buffered saline (PBS; 140 mM NaCl, 2.7 mM KCl, 0.1 mM Na2HPO4, and 1.8 mM KH2PO4, pH 7.2). Different concentrations of this suspension were prepared by dilution and then they were plated in LB agar – which was prepared by dissolving 6 g agar in 400 mL of LB media – to estimate total bacterial (viable) counts (color-forming units, CFU).
T4 phages were amplified using the bacterial suspension prepared in the previous step which was briefly as follows – as described also in the related literature (Clokie and Kropinski Citation2009, Kutter and Sulakvelidze Citation2004, Sambrook et al. Citation1989): 100 μL of E. coli K12 (108 CFU/mL) and 100 μL of T4 phage (108 PFU/mL) were mixed and then incubated at room temperature for 15 min and then added to LB media. The mixture was incubated for 6 h at 37 °C in a shaking incubator (200 rpm). Chloroform was added to final 10% (v/v) and the solution was kept at 4 °C for 20 min. For purification, the medium was first ultra-filtered through a sterile 0.22 μm filter and then centrifuged at 4 °C (∼12,000 g) for 20–30 min. The purified phages were re-suspended in sterile PBS buffer (see above) or in SM buffer composed of 0.1% gelatin, 100 mM NaCl, 8 mM MgSO4, 50 mM Tris-HCl, pH 7.5) and stored at 4 °C until use and phage concentration denoted as “plaque forming unit per milliliter” (PFU/mL) was determined as follows (Clokie and Kropinski Citation2009): phage emulsions with different concentrations were prepared by dilution of the initial phage suspension, 100 μL from each one and 400 μL of E. coli suspension were mixed and added to LB medium (semi-liquid – agar 7.5 g/L) and incubated at 37 °C for 24 h, and the lysis plagues were counted. The phage with a concentration of 108 PFU/mL was stored at 4 °C until use.
The performance (activity) of T4 phages propagated in the previous steps for infection and destruction of the bacteria (E. coli here) was evaluated by a culture method (Clokie and Kropinski Citation2009). Plates containing the target bacteria – E. coli – on agar broth were prepared. The phages were placed on the agar in the plates which were then incubated at 37 °C overnight. Note that the developing E. coli lawn plates were originally turbid. However, E. coli was destroyed by the phages and a transparent zone were formed due to lysis of the bacteria which was measured to exhibit the effectiveness of the phages.
Microencapsulation of phages within alginate beads
Phages were encapsulated within alginate beads by following a rather classical approach which is briefly as follows (Gåserød et al. Citation1998, Krasaekoopt et al. Citation2004): A mixture containing phages (about 108 PFU/mL) and 2.0% (w/v) sodium alginate (Sigma-Aldrich, Munich, Germany) prepared in 50 mM Tris HCl with a pH of 7.5 was added dropwise using a syringe pump into 100 mM CaCl2 solution to form the beads. They were incubated about 30 min for fully cross-linking the calcium alginate bead walls by gently shaking at room temperature. The wet beads were stored at 4 °C.
In order to increase the stability (prevent degradation of T4 phages) and controlled release of the encapsulated phages, the alginate beads hardened (cross-linked) with CaCl2 solution in the previous step were further treated with two different polycations, namely chitosan (Fluka – low viscosity, 75–85 amination degree, Japan), and branched PEI with a molecular weight of 20 kDa (Sigma-Aldrich, Germany) solutions. The alginate beads were incubated in the chitosan solutions (0.5–1%, wt/vol) for about 20–30 min to coat the surface of the beads with an alginate/chitosan layer (by polyanion/polycation complex formation) as described in detail by Gåserød et al. (Citation1998). As an alternative coating to increase the stability of Ca+2-alginate beads, branched PEI was used instead of chitosan. This is the first time in literature for further hardening/controlling of the microcapsule wall network structure. Briefly, the alginate prepared in the first step were placed into PEI solution (0.5–1%, wt/vol) and incubated at room temperature for about 20–30 min.
In order to observe the formation and swelling behavior of the three different alginate microcapsules carrying phages described above – with naked eye – different pigments were added into the initial solutions.
To obtain phage loadings, the microcapsules loaded with phages prepared in the previous steps were treated with a solution 50 mM sodium citrate, 0.2 M sodium bicarbonate, and 50 mM Tris-HCl, pH 7.5, for about 10 min by gently shaking at room temperature (Liu et al. Citation2002, Ma et al. Citation2008, Xue et al. Citation2004). The phages released from the beads were determined by titration as described above. Phage titer was expressed in PFU per gram of wet alginate beads and the average values and standard deviations (SD) of three repeating tests were found. The “phage-loading efficiency” (PLE) was calculated from the following equation:
Phage stability and release
In order to simulate behavior of both free and encapsulated phages in GI system, they were incubated in the “simulated gastric fluid” (SGF) and bile salts and in the “simulated intestinal fluid” (SIF) as described in the “United States Pharmacopeial Convention” (Citation2004).
Phage stability
First, stability of free and microencapsulated T4 phages was investigated in SGF which was prepared by dissolving 320 mg pepsin in 100 mL of 0.2% (wt/vol) NaCl solution at pH 2.5 – adjusted with 1 M HCl. 100 μl of phage suspension (108 PFU/mL) or 1 g fresh alginate microcapsules were placed into culture vessels containing about 10 mL SGF at 37 °C for 2 h – samples were taken at pre-determined time intervals. Samples containing free phages were collected, diluted, and immediately assayed for phage survival. For the microcapsules, the incubation was terminated by placing the microcapsules carrying phages in 10 mL SM buffer (pH 7.5). Phage survivals (the percentage of the phages is still active) were assayed as described above and results were presented in mean ± SD.
A similar protocol was applied for the determination of effects of bile salts on free and encapsulated phages in alginate beads – except incubations were conducted in the simulated bile containing 1% (wt/vol) porcine bile extract (Sigma-Aldrich, Germany) at 37 °C for 2 h.
Phage release in SIF
Phage release experiments were performed in “SIF” as described in the “United States Pharmacopeial Convention” (Citation2004). About 200 mg of fresh alginate microcapsules were incubated in 50 mL SIF – which consisted of 10 mg/mL pancreatin (Sigma-Aldrich, Germany) in 50 mM KH2PO4 at pH 6.8 – by gently shaking for upto 2 h. About 100 μL samples were withdrawn from the medium at selected time intervals (replaced with fresh medium), and the amount of active phages remain in the microbeads were determined as described above. The cumulative amount of phages released was plotted against time to demonstrate the phage release kinetics. The released phage was titrated as described previously by Gill et al. (Citation2006).
Results and discussion
Phages in alginate microbeads
Some representative pictures of the alginate-based microbeads are given in . Note that they were also labeled with different colors just for demonstration of microbeads with different wall chemistries. The initial recipes (amount of sodium alginate and T4 phage) were all same in all these microcapsules. Note that considering practical use and also remarks saying that “small size alginate beads are not effective to protect their content from low pH” we have decided to apply conditions and recipe to produce larger beads around one millimeter for encapsulation of T4 phages in this study (Hansen et al. Citation2002). The dropping speed and agitation in the collecting vessel (containing calcium chloride) were the same, therefore the sizes were almost the same which were around 900–950 microns (the mean) and size distribution was quite narrow (SD ±50). Further treatment with chitosan or PEI at the second step increased only the capsule wall structure had no significant effect on the microbead size.
Figure 2. Representative pictures of phages encapsulated within alginate beads and further coated with chitosan or PEI.
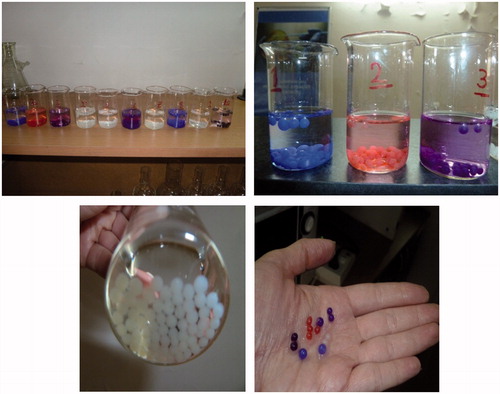
The PLE in all formulations were over 90 ± 5%, which shows that the first step is quite successful and further treatments in the second step (chitosan or PEI coatings) had no observable adverse effect on the phages encapsulated. These values are similar in alginate encapsulation even those small active molecules – usually quite high entrapment efficiencies, comparing to other encapsulation techniques.
The free T4 was found quite sensitive to acidic pH (2.5), its activity dropped below the detection limit 103 PFU/mL less than short incubation times however at the physiological pH of 7.4, there was less than 5% decrease in the activity in about 2 h at 37 °C. Note that pH sensitivities of different phages may be different.
shows the activity changes with time (120 min) in SGF media at 37 °C for 2 h. Note that encapsulation of phages in the alginate capsules did reduce the activity loss significantly comparing to the free phage, as also demonstrated in the similar studies where phages or other bioactive agents – such as bifidobacteria (Lee and Heo Citation2000) have been encapsulated within alginate gels. The simple explanation is that the Ca+2–alginate network (wall) slowdowns the acidic SGF medium transfer – the protons diffusion into the microcapsules preventing immediate/sudden exposure of the naked phages which causes activity loss. Tang et al. (Citation2005) have demonstrated that diffusion of acids through alginate networks is significantly lower than in an aqueous medium – which supports this explanation.
Figure 3. Free phage activity at room temperature (A). The activity changes with time (120 min) in SGF media at 37 °C: (B) Ca+2–alginate beads; (C) Ca+2–alginate beads coated with a chitosan/alginate layer; and (D) Ca+2–alginate coated with a PEI/alginate layer.
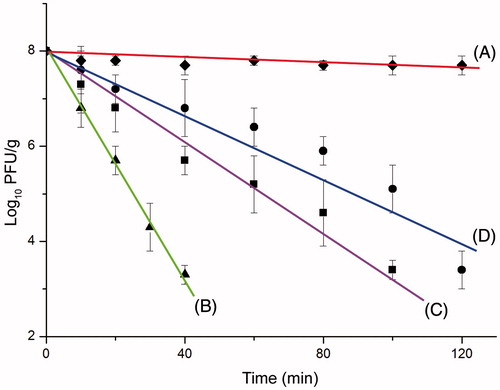
Chitosan and PEI coatings were quite effective on protection, which may be due to formation of thicker, stronger and more tight network (low permeability) of the microcapsule walls. DeGroot and Neufeld (Citation2001) and Gåserød et al. (1998) have also illustrated that drying and chitosan coating of Ca+2–alginate beads resulted in a very compact structure with a significantly reduced pore size, retarding proton diffusion into alginate matrix. Note also that both polycations have some kind of buffering effect on surrounding medium layers of microcapsules which also positively effects phage safety. This was the rational of this study for the selection of PEI for coating. Its buffering effects have been well documented in the use of as transfection agent (nonviral vector) in animal cells. After the vector carrying the gene modification plasmid DNA is taken in endosomes PEI prevents pH drop and save the plasmid from degradation by lysosemic enzymes. It seems that the branched PEI more successfully interacts with alginate and a quite high number of positively charged amino groups still stay on the surface which cause buffering around the alginate beads.
Phage stability in bile salts
Stabilities both free and microencapsulated T4 phages in bile salts were also studied. Note that the titers of free T4 phage in 1% bile salts after 2 h of incubation decreased by about 1.44 ± 0.12 log10 PFU/mL, similar bile acid sensitivities were reported by others (Ma et al. Citation2008). In the case of Ca+2–alginate encapsulation, the tolerance of the phages in this immobilized form reduced – in 2 h incubation decrease was about 0.56 ± 0.14 log10 PFU/g alginate beads. However, the viability of phages in alginate beads coated with chitosan or PEI was almost the same after 2 h incubation in bile salts.
There are different reports about the tolerance of phages to bile acids, for instance Vibrio vulnificus phage is very resistant while the Staphylococcal phage K is less tolerant (Koo et al. Citation2000, Ma et al. Citation2008). T4 was also sensitive to bile acids, as presented above. However, especially polycationic coatings on microcapsules carrying phages increases stability of phages against bile salts as we have observed in this study. As also presented in the related literature, this may be explained as follows: most probably the negatively charged bile acids are interacted with polycations on the microcapsule surfaces formed insoluble complexes which in turn increased the mass transfer resistance at the surface preventing the bile salts enter the capsule and therefore their deleterious effects on phages (Krasaekoopt et al. Citation2004, Ma et al. Citation2008, Murata et al. Citation1999). Krasaekoopt et al. (Citation2004) also reported that the chitosan-coated alginate beads provided better protection for probiotic bacteria in bile salt solution than either poly-L-lysine or alginate.
Phage release
shows representative pictures of alginate beads in SIF, they do swell first and totally degraded as seen on the figure.
gives representative phage-release curves for three different phage carrying alginate microcapsules: (A) only Ca+2–alginate wall; (B) further treated with chitosan; (C) further treated with branched PEI. As seen here, release from Ca+2–alginate is rather fast – about 80–90% of the phages were released in about 6 h, and release was completed in about 12 h. However, the release was significantly slow downed in case of polycation-treated alginate beads. Interestingly that was faster in case of PEI coating. In general, this is a typical behavior that one can expect and can be explained/discussed as follows.
Figure 5. Phage release from alginate-based beads carrying T4 phages: (A) Ca+2–alginate beads; (B) Ca+2–alginate beads coated with a chitosan/alginate layer; and (C) Ca+2–alginate coated with a PEI/alginate layer.
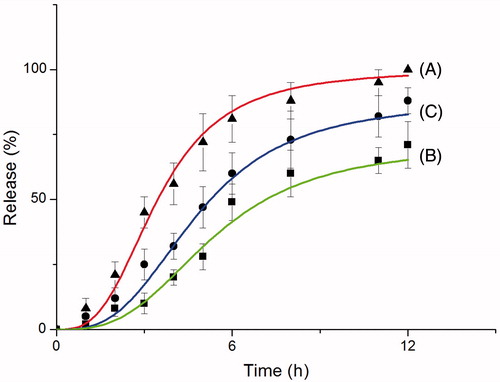
The main rational of using alginate microcapsules (beads) is their pH responsive properties. In acidic media (like gastric fluid at around pH 2.0–2.5) alginate gels are in compact (collapse) form and the structure of the Ca+2–alginate network is rather tight and therefore does not allow high transfer rates (rather a low diffusion barrier). However, when the pH is increased to higher values such as in small intestine medium (SIF applied in this study), the matrix starts to swell (even quite rapidly) due to ion-exchange process between the monovalent ions (e.g., Na+1) within the medium with the Ca+2 on the alginate gel wall and the two valence ions Ca+2 that is cross-linking the alginate polyanions. The network (microcapsule wall) starts to disintegrate and finally the content is released by erosion (Kikuchi et al. Citation1999, Ma et al. Citation2012). This is the well-known pH-dependent behavior of alginate capsules that has been the rational of preparing many alginate-based sustained release systems to be used in the GI tract. Note that – as expected – both the size and wall structure significantly affects this disintegration process. Smaller bead size means high surface area and therefore faster transport for ion-exchange and therefore disintegration is expected.
In the studies here, size of the beads were large around 1 mm, however – following the related literature – alginate beads carrying phages – have been further treated with polycations namely chitosan (there are a number of studies with this natural-based polymer) and branched PEI (which is the first time used in this study) to control swelling behaviour of Ca+2–alginate beads. The main aim was to increase the stability of phages – protect them from the acidic medium in gastric fluid and allow them to disintegrate and release of their content (T4 phage here) within bowels. The polyanionic alginate capsules are expected to form polyelectrolyte structure with polycationic polymers (chitosan and PEI). It is a rather tight network because of multipoint ionic interaction between these two oppositely charged polymers. Therefore, it is expected that the whole process swelling-disintegration-erosion will be different as we have observed here, most probably due to branched structure of PEI, diffusion of monovalent cations was faster and therefore disintegration and release of phages was also faster.
Note that polyelectrolyte wall encapsulating the phages is a network structure and release of the content depends on the tightness of this network. Most probably due to very tight structure of alginate beads at acidic pH, there will be no phage release, however when the disintegration starts at small intestine media the network structure become loose and allow both inward and outward diffusion, that ions and small molecules can easily diffuse in and out. Note that T4 is a rather large entity, cannot penetrate through the capsule wall that easily. Note also that the pore size of alginate gel network can be around down to 5 nm (Andresen et al. Citation1977) which is quite tight and does not allow any phage release in the initial disintegration process in bowel fluids. Therefore, the release of T4 is rather sustained longer periods that higher disintegration (network tightness decrease) even erosion as we have observed.
Conclusion
In this study, T4 phages were first encapsulated within alginate beads by following the classical technique – dropping the phage emulsions in sodium alginate solutions into a calcium chloride bath for cross-linking of alginate with calcium cations to form alginate beads which allowed us to reach loadings over 90%. In order to further stabilize these beads in GI tract conditions – by preventing from low pH and enzymatic attack for the degradation of phages – these beads were coated with two alternative polycations, chitosan (a rather conventional one) and branched PEI, that is widely used in preparation of nonviral vectors in transfection studies including our own studies (Piskin et al. Citation2004). Coating with those polycations increased significantly stability of phages both in “SGF” and bile salts especially in the case of PEI coating. The swelling behavior and phage release tests were conducted in “SIF”. These studies nicely demonstrated that phage could be released from the beads at basic pH. The release rates were higher in the case of PEI coating. It was concluded that PEI is better coating material than chitosan both for increasing stability at low pH values and release its content rather easily in intestinal conditions which were originally aimed in the design of this study. Studies related to PEI-coated alginate beads not only for phage immobilization but also encapsulation of other pH-sensitive molecules, e.g., vitamins or entities such as probiotic bacteria are under investigation.
Funding information
This study is supported by EU-FP7-IAPP-NanobacterphageSERS project (project no: 286464). E.P. is supported by Turkish Academy of Sciences as honorary member.
Acknowledgements
We would like to thank Eliava BioPreparations Ltd., Georgia for initiation of this study and supply of phages for the initial studies.
Disclosure statement
The authors report no conflicts of interest. The authors alone are responsible for the content and writing of this article.
References
- Abuladze T, Li M, Menetrez MY, Dean T, Senecal A, Sulakvelidze A. 2008. Bacteriophages reduce experimental contamination of hard surfaces, tomato, spinach, broccoli, and ground beef by Escherichia coli O157: H7. Appl Environ Microbiol. 74:6230–6238.
- Andresen IL, Skipnes O, Smidsrod O, Ostgaard K, Hemmer Per CHR. 1977. Some biological functions of matrix components in benthic algae in relation to their chemistry and the composition of seawater. Cell Chem Technol. 48:361–381.
- Bach SJ, Johnson RP, Stanford K, McAllister TA. 2009. Bacteriophages reduce Escherichia coli O157: H7 levels in experimentally inoculated sheep. Can J Anim Sci. 89:285–293.
- Burgain J, Gaiani C, Linder M, Scher J. 2011. Encapsulation of probiotic living cells: from laboratory scale to industrial applications. J Food Eng. 104:467–483.
- Chibani-Chennoufi S, Sidoti J, Bruttin A, Kutter E, Sarker S, Brüssow H. 2004. In vitro and in vivo bacteriolytic activities of Escherichia coli phages: implications for phage therapy. Antimicrob Agent Chemother. 48:2558–2569.
- Clokie MRJ, Kropinski AM, Eds. 2009. Bacteriophages: Methods and Protocols, Volume 1: Isolation, Characterization, and Interactions. New York City (NY): Humana Press, a part of Springer Science + Business Media.
- Cook MT, Tzortzis G, Charalampopoulos D, Khutoryanskiy VV. 2012. Microencapsulation of probiotics for gastrointestinal delivery. J Control Release. 162:56–67.
- Cui JH, Goh JS, Kim PH, Choi SH, Lee BJ. 2000. Survival and stability of bifidobacteria loaded in alginate poly-l-lysine microparticles. Int J Pharm. 210:51–59.
- DeGroot AR, Neufeld RJ. 2001. Encapsulation of urease in alginate beads and protection from α-chymotrypsin with chitosan membranes. Enzyme Microb Technol. 29:321–327.
- Gåserød O, Smidsrød O, Skjåk-Bræk G. 1998. Microcapsules of alginate-chitosan-I. A quantitative study of the interaction between alginate and chitosan. Biomaterials. 19:1815–1825.
- George M, Abraham TE. 2006. Polyionic hydrocolloids for the intestinal delivery of protein drugs: alginate and chitosan-a review. J Control Release 114:1–14.
- Gill JJ, Pacan JC, Carson ME, Leslie KE, Griffiths MW, Sabour PM. 2006. Efficacy and pharmacokinetics of bacteriophage therapy in treatment of subclinical Staphylococcus aureus mastitis in lactating dairy cattle. Antimicrob Agent Chemother. 50:2912–2918.
- Hansen LT, Allan-Wojtas PM, Jin YL, Paulson AT. 2002. Survival of Ca-alginate microencapsulated Bifidobacterium spp. in milk and simulated gastrointestinal conditions. Food Microbiol. 19:35–45.
- Heidebach T, Först P, Kulozik U. 2012. Microencapsulation of probiotic cells for food applications. Crit Rev Food Sci Nutr. 52:291–311.
- Housby JN, Mann NH. 2009. Phage therapy. Drug Discov Today. 14:536–540.
- Joerger RD. 2003. Alternatives to antibiotics: bacteriocins, antimicrobial peptides and bacteriophages. Poult Sci. 82:640–647.
- Kikuchi A, Kawabuchi M, Watanabe A, Sugihara M, Sakurai Y, Okano T. 1999. Effect of Ca2+-alginate gel dissolution on release of dextran with different molecular weights. J Control Release. 58:21–28.
- Koo J, DePaola A, Marshall DL. 2000. Effect of simulated gastric fluid and bile on survival of Vibrio vulnificus and Vibrio vulnificus phage. J Food Protect. 63:1665–1669.
- Krasaekoopt W, Bhandari B, Deeth H. 2004. The influence of coating materials on some properties of alginate beads and survivability of microencapsulated probiotic bacteria. Int Dairy J. 14:737–743.
- Kutter E, Sulakvelidze A, Eds. 2004. Bacteriophages: Biology and Applications. Boca Raton (FL): CRC Press.
- Lee KY, Heo TR. 2000. Survival of Bifidobacterium longum immobilized in calcium alginate beads in simulated gastric juices and bile salt solution. Appl Environ Microbiol. 66:869–873.
- Li XY, Jin LJ, McAllister TA, Stanford K, Xu JY, Lu YN, et al. 2007. Chitosan-alginate microcapsules for oral delivery of egg yolk immunoglobulin (IgY). J Agricult Food Chem. 55:2911–2917.
- Liu XD, Yu WY, Zhang Y, Xue WM, Yu WT, Xiong Y, et al. 2002. Characterization of structure and diffusion behaviour of Ca-alginate beads prepared with external or internal calcium sources. J Microencapsul. 19:775–782.
- Ma Y, Pacan JC, Wang Q, Sabour PM, Huang X, Xu Y. 2012. Enhanced alginate microspheres as means of oral delivery of bacteriophage for reducing Staphylococcus aureus intestinal carriage. Food Hydrocoll. 26:434–440.
- Ma Y, Pacan JC, Wang Q, Xu Y, Huang X, Korenevsky A, Sabour PM. 2008. Microencapsulation of bacteriophage felix O1 into chitosan-alginate microspheres for oral delivery. Appl Environ Microbiol. 74:4799–4805.
- McKnight CA, Ku A, Goosen MFA, Sun D, Penney C. 1988. Synthesis of chitosan-alginate microcapsule membranes. J Bioact Compat Polym. 3:334–355.
- Mortazavian AM, Azizi A, Ehsani MR, Razavi SH, Mousavi SM, Sohrabvandi S, Reinheimer JA. 2008. Survival of encapsulated probiotic bacteria in Iranian yogurt drink (Doogh) after the product exposure to simulated gastrointestinal conditions. Milchwissenschaft. 63:427–429.
- Murata Y, Maeda T, Miyamoto E, Kawashima S. 1993. Preparation of chitosan-reinforced alginate gel beads—effects of chitosan on gel matrix erosion. Int J Pharm. 96:139–145.
- Murata Y, Toniwa S, Miyamoto E, Kawashima S. 1999. Preparation of alginate gel beads containing chitosan salt and their function. Int J Pharm. 176:265–268.
- Overgaard S, Scharer JM, Moo‐Young M, Bols NC. 1991. Immobilization of hybridoma cells in chitosan alginate beads. Can J Chem Eng. 69:439–443.
- Piskin E, Dinçer S, Türk M. 2004. Gene delivery: intelligent but just at the beginning. J Biomater Sci Polym. 15:1181–1202.
- Puapermpoonsiri U, Spencer J, van der Walle CF. 2009. A freeze-dried formulation of bacteriophage encapsulated in biodegradable microspheres. Eur J Pharm Biopharm. 72:26–33.
- Rajam R, Karthik P, Parthasarathi S, Joseph GS, Anandharamakrishnan C. 2012. Effect of whey protein–alginate wall systems on survival of microencapsulated Lactobacillus plantarum in simulated gastrointestinal conditions. J Funct Foods. 4:891–898.
- Sambrook J, Fritsch EF, Maniatis T. 1989. Molecular Cloning: A Laboratory Manual. Vol. 3. Cold Spring Harbor (NY): Cold Spring Harbor Laboratory Press, p. 2344.
- Smith HW, Huggins MB. 1983. Effectiveness of phages in treating experimental Escherichia coli diarrhoea in calves, piglets and lambs. J Gen Microbiol. 129:2659–2675.
- Smith HW, Huggins MB, Shaw KM. 1987. Factors influencing the survival and multiplication of bacteriophages in calves and in their environment. J Gen Microbiol. 133:1127–1135.
- Soothill J, Hawkins C, Änggår E, Harper D. 2004. Therapeutic use of bacteriophages. Lancet Infect Dis. 4:544–545.
- Sulakvelidze A, Alavidze Z, Morris JG. 2001. Bacteriophage therapy. Antimicrob Agent Chemother. 45:649–659.
- Sulakvelidze A, Barrow P. 2005. Phage therapy in animals and agribusiness. In: Kutter E, Sulakvelidze A, Eds. Bacteriophages: Biology and Application. Boca Raton (FL): CRC Press, pp. 335–380.
- Sulakvelidze A, Kutter E. 2005. 14 bacteriophage therapy in humans. In: Kutter E, Sulakvelidze A, Eds. Bacteriophages: Biology and Application. Boca Raton (FL): CRC Press, pp. 381–436.
- Sultana K, Godward G, Reynolds N, Arumugaswamy R, Peiris P, Kailasapathy K. 2000. Encapsulation of probiotic bacteria with alginate–starch and evaluation of survival in simulated gastrointestinal conditions and in yoghurt. Int J Food Microbiol. 62:47–55.
- Tang M, Dettmar P, Batchelor H. 2005. Bioadhesive oesophageal bandages: protection against acid and pepsin injury. Int J Pharm. 292:169–177.
- United States Pharmacopeial Convention. 2004. The United States Pharmacopeia, 27th ed. Rockville (MD): United States Pharmacopeial Convention, p. 2728.
- Xue WM, Yu WT, Liu XD, He X, Wang W, Ma XJ. 2004. Chemical method of breaking the cell-loaded sodium alginate/chitosan microcapsules. Chem J Chin Univ. 25:1342–1346.
- Wang Q, Sabour PM. 2010. Encapsulation and controlled release of bacteriophages for food animal production. In: Sabour P, Griffiths M, Eds. Bacteriophages in the Control of food-and Waterborne Pathogens. Washington (DC): ASM Press, pp. 237–255.
- Weber-Dabrowska B, Mulczyk M, Górski A. 2003. Bacteriophages as an efficient therapy for antibiotic-resistant septicemia in man. Transplant Proc. 35:1385–1386.
- Wee SF, Gombotz WR. 1998. Protein release from alginate matrices. Adv Drug Deliv Rev. 31:267–285.
- World Health Organization. 2012. The Evolving Threat of Antimicrobial Resistance. Options for Action. Geneva: World Health Organization.