Abstract
In the present study, silver nanoparticles (AgNPs) were synthesized by using aqueous root extracts of Rheum palmatum and characterized by various spectroscopic methods. The nanoparticles were found to be in hexagonal and spherical shapes. The average particle size was found to be 121 ± 2 nm with zeta potential values of −21.6 mv by dynamic light scattering (DLS) method. Gas chromatography–mass spectrometry (GC-MS) analysis of R. palmatum revealed 35 compounds. The synthesized AgNPs showed significant activity against Staphylococcus aureus and Pseudomonas aeruginosa with IC90 values of 15 μg/ml and IC50 values of 7.5 μg/ml, respectively. The protein leakage level was high and morphological changes occurred in bacteria treated with AgNPs.
Introduction
Nanotechnology is the field that deals with design, manipulation, production, and application of materials ranging in nanometer scale. Nanoparticles (NPs) are widely applied in medical and pharmaceutical fields. They are also used in fast-moving consumer goods, such as shampoos, soaps, detergents, cosmetic products, and toothpaste (Kim and Song Citation2010). Moreover, silver nanoparticles (AgNPs) are reported to possess a wide range of properties, including optical, electrical conductivity, catalytic, sensing and bactericidal effect (Abou El-Nour et al. Citation2010, Rodrıguez-León et al. Citation2013, Sharma et al. Citation2009). There are many methods for the synthesis of NPs, including laser ablation, electron irradiation, gamma irradiation, and use of chemicals, as reducing and capping agents. The main problems with these methods are expensive, use of toxic chemicals that are not safe to environment and human health. Plants are used for synthesis of NPs and have advantages over physical and chemical processes. In recent years, synthesis of AgNPs using plant extracts have been increasingly reported (Arokiyaraj et al. Citation2014, Chitsazi et al. Citation2016, Prakash et al. Citation2013, Veerasamy R et al. Citation2010, Umashankari et al. Citation2012) because, they are easily available, simple, environmentally friendly and have wide range of secondary metabolites that act as reducing agents (Bhattacharya and Gupta Citation2005, Savithramma et al. Citation2011).
Rheum palmatum L. (Polygonaceae) is one of the important medicinal plants commonly known as Chinese rhubarb, distributed in western China, northern Tibet, and the Mongolian Plateau. In Chinese medicine, it has been used as laxative, antiphlogistic, in the treatment of indigestion and other disorders. It has been reported to possess antibacterial and antiproliferative activity (Aly and Gumgumjee Citation2011, Kang et al. Citation2008). In the present study, we report the green synthesis of stable bioactive AgNPs using an aqueous root extract of R. palmatum. The synthesized AgNPs were characterized by ultraviolet-visible spectroscopy, X-ray diffraction spectroscopy (XRD), scanning electron microscope (SEM), energy-dispersive X-ray spectroscopy (EDAX), transmission electron microscope (TEM), dynamic light scattering (DLS), zeta potential, and Fourier transform infrared spectroscopy (FTIR). The antibacterial activity of green synthesized AgNPs was studied with S. aureus and P. aeruginosa. Further, the leakage of protein and morphological changes of bacterial cells treated with AgNPs were observed by scanning electron microscope.
Materials and Methods
Microorganisms
Staphylococcus aureus (ATCC 25923) and Pseudomonas aeruginosa (ATCC 27853) were obtained from the Korean culture center of microorganisms, Republic of Korea. These strains were sub cultured time to time in order to regulate the viability and were maintained on MHA agar slants in the microbiology laboratory and stored at 4 °C for further study.
Preparation of extracts and synthesis of AgNPs
Roots of R. palmatum were purchased from the Kyueng dong market, Republic of Korea and washed frequently with Milli-Q deionized water. Two grams of powder was mixed with 100 ml of Milli-Q deionized water and incubated at 40 °C for 24 h. After incubation, the aqueous extract was filtered with Whatman No.1 filter paper. Then, 5 ml of extract was mixed with 100 ml of silver nitrate (2 mmol) solution. The reaction mixture was kept undisturbed at room temperature until the conversion of colorless solution into reddish brown color which indicated the formation of AgNPs (). The particles were then centrifuged and stored in screw-capped bottles for further characterization and antibacterial activity.
Gas chromatography–mass spectrometry analysis (GC-MS)
Rheum palmatum extract was analyzed by a Thermo Scientific Trace 1310 (Gas chromatograph) system with a Thermo ISQ LT mass selective detector. The machine was equipped with a TG-5MS (Mass spectroscopy) column (30 × 0.25 mm (5%-phenyl)–methylpolysiloxane capillary column, film thickness × 0.25 μm), 220 °C temperature injector and 250 °C temperature transfer line. The oven temperature was programmed as follows: initial temperature; 50 °C for 5 min and then increase 4 °C/min up to 250 °C. The carrier gas used was helium at a flow rate of 1.0 ml/min. The amount of sample injected was 1 μl and the ionization energy was 70 eV. Identification of individual components was based on their retention time and by comparison of their mass spectral pattern with the NIST library (National Institute of Standards and Technology).
Characterization of AgNPs
The reduction of silver ions was monitored by UV-vis spectrophotometer (Cyberlab UV 100, USA) in the wavelength ranging from 350–800 nm. The crystalline structure of the synthesized AgNPs was investigated by X-ray diffraction pattern using Bruker D8 advance diffractometer, operated at 40 kv and a current of 40 mA with Cu/kα radiation source (λ = 1.5412 Å) in the range of 5° to 90°, in 2θ angles with a scanning rate of 0.5 s/step. SEM and EDAX analysis were performed by using Oxford Inca Penta FeTX3 EDS instrument attached to Carl Zeiss EVO MA 15 scanning electron microscope with a line resolution 2.32 (in Å). The size and shape of the NPs were obtained on JEOL JEM 2100 HRTEM operating at 200 kv accelerating voltage. The samples were prepared by drop-casting 10 μl aliquots of AgNPs solution onto a carbon coated copper grid, which was placed on a piece of paper to get rid of excess solvent. The average particle size distribution and stability of the biosynthesized AgNPs were evaluated by using ELSZ-1000 zeta-potential and particle size analyzer (Otsuka Electronics Co., Ltd., Osaka, Japan). For FTIR analysis, the powdered AgNPs were recorded by FTIR spectrometer (Thermo Scientific Nicolet 6700, Waltham, MA) over the frequency of 4000–400 cm−1 at a resolution of 4 cm−1 by using a KBr pellet method.
Antibacterial assay
The antibacterial activity of green synthesized AgNPs was performed by well diffusion method (Irobi et al. Citation1994). S. aureus and P. aeruginosa bacterial cultures were sub-cultured in Muller Hinton broth and incubated at 37 °C for 14 h. Then, the cultures were swabbed onto petri plates containing Muller Hinton agar using sterile cotton swab. Wells of 6 mm in diameter were puncture onto the agar plates and 30 μg/ml of AgNPs solution and aqueous plant extract were loaded into the wells. The plates were incubated and the zone of inhibition of each well was measured. Streptomycin (30 μg/ml) was used as control to compare the effectiveness of AgNPs and plant extracts against tested bacteria. The minimum inhibitory concentration (MIC) was studied using twofold dilution method with initial test concentration of 30 μg/ml by following Rejiniemon et al. Citation2014. The MIC was calculated as the lowest concentration of the AgNPs inhibiting the visual growth of the test cultures on the agar plates. The experiments were conducted in triplicates.
Intracellular protein leakage
The bacterial cultures were treated with 30 μg/ml of AgNPs and incubated for 8 h at 37 °C. After incubation, the cells were centrifuged at 5000 rpm for 10 min and the supernatants were collected. To determine the intracellular protein leakage, the supernatant was assayed according to the method of Bradford (Citation1976). The assay mixture consisted of 1 ml of supernatant added with 0.5 M NaOH (2 ml) and 0.1 N folin (0.1 ml) phenol reagent and the absorbance was read at 550 nm after 10 min.
Scanning electron microscope observation
SEM (Carl Zeiss EVO MA 15) was used to observe the morphological changes of bacterial cells treated with AgNPs and control cell. Bacterial cells (106 CFU/ml) were treated with 15 μg/ml of AgNPs and incubated for 14 h. After incubation, the cells were centrifuged at 5000 RPM for 30 min. The obtained pellets were washed with phosphate-buffered saline (PBS) three times and prefixed with 10% formaldehyde for 30 min. The prefixed cells were dehydrated with ethanol (30, 50, 70, 80, 90, and 100%), air-dried and gold-coated using an ion sputter.
Statistical analysis
The data were expressed as mean ± standard deviation (SD) for antibacterial and protein leakage assays. The differences between control and treated groups in protein leakage assay were carried out by Student’s t-test. P < 0.05 was considered as significant.
Results and discussion
Phytochemical analysis
GC–MS analysis of R. palmatum showed the presence of 35 compounds and (was) compared with NIST/NBS spectral database (). The major compounds were 4–(4-hydroxyphenyl)-2-butanone (21.06%), diisooctyl phthalate (20.98%), hexanedioic acid, bis(2-ethylhexyl)ester (16.58%), and anthraquinone (7.95%). Earlier studies reported that the rhizomes of R. palmatum contain glycosides, anthraquinone, fatty acids, tannins and 108 volatile components (Miyazawa et al. Citation1996). In the present study, phenolic compound, ester, and anthraquinone were found to be in high levels. The present study hypothesizes that phenolic compound and anthraquinone present in the root extract might be responsible for the reduction of silver ions and formation of stable NPs. Jha et al. (Citation2009) have explained the anthraquinone in Bryophyllum sp. responsible for the formation of AgNPs by undergoing tautomerization. Similarly, there are several reports indicating the involvement of phenolic compounds as reducing and capping agent for AgNPs synthesis (Castanon et al. Citation2008, Pietta Citation2000, Vedpriya Citation2010).
Table 1. Phytocomponents identified in the extract of Rheuman palmatum by GC–MS.
Characterization
The UV-Vis spectrum is one of the imperative techniques to find out the reduced concentration of metal NPs in solution. In this study, UV-Vis analysis revealed the excitation of surface plasmon resonance (SPR) band at 440 nm (). This could be due to a smaller dimension, SPR usually arises from the presence of free electrons in the conduction band. displays the XRD pattern of silver nanoparticles, the observed diffraction peaks were indexed as (111), (200), (220), (311), and (222) reflections that are in agreement with a cubic crystal phase of Ag metal (JCPDS card No. 65–2871). The remaining unassigned peaks are due to the formation of the crystalline biosubstances that are compiled onto the surface of Ag particles. The line broadening of XRD peaks indicates, the formation of very fine Ag particles in the nanoscale regime, which is also in agreement with previous report (Manivasagan et al. Citation2013). The size of the AgNPs was analyzed by DLS method and the particle size was found to be in the range of 11 to 210 nm with an average size of 121.5 nm (). The zeta potential value was −21.65 mv, which confirms the high stability of AgNPs (). Elia et al. (Citation2014) have synthesized Au-NPs from Punica granatum and characterized them using DLS spectroscopy wherein the particles are in the range of 34–312 nm.
Figure 2. (a) UV-vis spectra of Rheum Palmatum and AgNPs, (b) XRD pattern of biosynthesized AgNPs (c) DLS profile of biosynthesized AgNPs (average size 121.5 nm) (d) zeta potential analysis (−21.6 mV) of biosynthesized AgNPs.
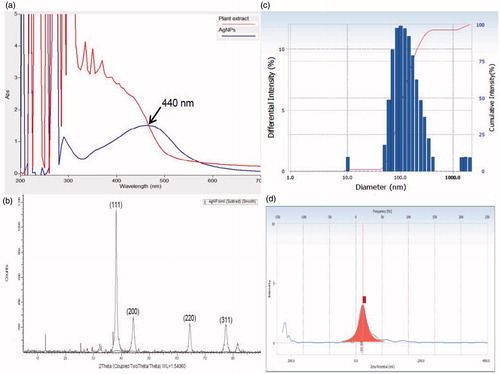
The morphology and size of the AgNPs were studied by using SEM and TEM techniques (). TEM images of AgNPs showed the particles distributed individually in different shapes, such as hexagon and spheres, with sizes ranging from 44–113 nm. Similarly, Padalia et al. (Citation2015) have reported the synthesis of AgNPs using marigold flower, where TEM analysis showed spherical and hexagonal shape particles in the range between 10 to 90 nm. Thus, the DLS, SEM, and TEM studies gave similar results for the size range of the nanoparticles. displays the clear elemental composition profile of the biosynthesized silver nanoparticles. EDAX profile displays powerful silver signals. The other weak signals are due to the incident of metabolites present in the extract of R. palmatum. The occurrence of the elemental silver can be discerned in the graph from EDAX investigation, which furthermore carries the XRD outcomes.
Figure 3. (a) SEM image of AgNPs synthesised using root extract of Rheum palmatum showing the particle size range from 84–130 nm, (b) EDAX, (c&d) TEM image of AgNPs synthesised using root extract of Rheum palmatum showing particle size ranges from 44 to 113 nm.
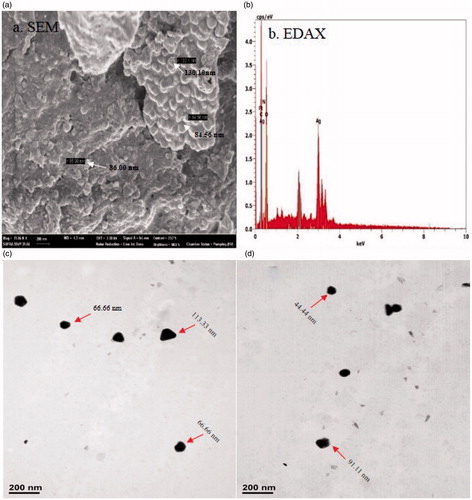
FTIR spectrum () of R. palmatum shows the band at 3420 cm −1 which is assigned to O–H stretching of phenolic compounds, fatty acids, and water. The band 2926 cm−1 is assigned to C–H stretching of methylene group in aliphatic hydrocarbons, esters, and fatty acids, 1697 cm−1 is assigned to C=O stretching of fatty acid, ketones, aldehyde, and esters, 1620 cm 1 is assigned to H–O–H bending of water, 1517 cm−1 is due to C=C stretching of aromatic compounds, 1449 cm−1 is assigned to C–O–H in-plane bending of fatty acids, 1227 cm−1 is assigned to C–O stretching of fatty acid and ester and 1037 cm−1 is due to C–O stretching of phenols, alcohols, and Si-O-Si asymmetric stretching of siloxanes.
Figure 4. FTIR spectra of (a) Rheum palmatum and (b) capped reducing phytoconstituents responsible for the synthesis of AgNPs.
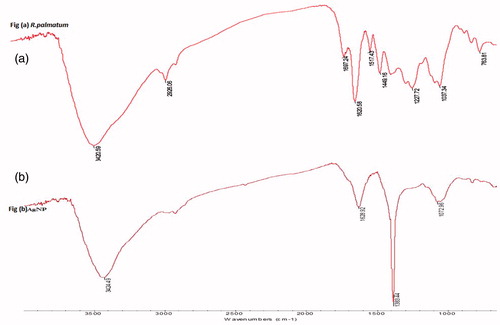
By comparing the infrared spectra of plant extract and AgNPs (), it is observed that the intensity of the peaks at 3420 cm−1, 2926 cm −1, 1620 cm−1, and 1037 cm−1 in plant extract has decreased and then shifted to higher wavenumbers in the infrared spectrum of silver nanoparticles. The band at 3424 cm−1 is assigned to O–H stretching of water, 2940 cm−1 is assigned to methyl C–H stretching of esters, 1628 cm−1 is assigned to H–O–H bending of water, 1383 cm−1 is assigned to methyl symmetrical C–H bending of esters, and band at 1072 cm−1 is assigned to C–O stretching of ester, carbohydrates, and Si–O–Si asymmetric stretching of siloxanes. The infrared peak at 2926 cm−1 which is related to C-H stretch of aliphatic hydrocarbons, esters, and fatty acids in the plant extract became less intense and shifted to 2940 cm−1. The band at 1620 cm−1 assigned to H–O–H bending of water became less intense and shifted to 1628 cm−1. The band at 1697 cm −1 which is assigned to C=O stretching of fatty acid, ketones, aldehyde, and esters disappeared. Further, the peak at 1383 cm−1 which is assigned to methyl symmetrical C–H bending of esters became sharp. It confirms that AgNPs synthesized from the R. palmatum extracts are stabilized by phytoconstituents through their free functional groups or residues (Song et al. Citation2009, Shankar et al. Citation2003).
Antibacterial activity
NPs have been noted for their antibacterial activity and our green synthesized AgNPs showed mean zone of inhibition against S. aureus (15 mm) and P. aeruginosa (13 mm) (). The IC90 and IC50 values were noted to be 15 μg/ml and 7.5 μg/ml for both strains. explains the possible antibacterial actions of green synthesized AgNPs: (i) may be due to the formation of free radicals causing cell wall damage, (ii) DNA damage, and (iii) protein synthesis inhibition (Hatchett and Henry Citation1996, Kim et al. Citation2011). Krishnaraj et al. (Citation2010) synthesized Ag-NPs using the leaf extract of Acalypha indica and 10 μg/ml was found to be minimum inhibitory concentration (MIC) against E. coli and V. Cholera. This was explained by the fact that the antibacterial activity was due to the change in membrane permeability. In the present study, we choose S. aureus and P. aeruginosa due to their importance on hospital-acquired infection and reported to be antibiotic resistances. These two pathogens are the causative agents for several infections, such as urinary tract infection, endocarditis, septicemia, and osteomyelitis (Tambekar and Dahikar Citation2011, Yoshikawa Citation2002).
Protein leakage
The amount of protein leakage upon treatment with AgNPs was quantified. The protein leakage from the NPs treated bacterial cells was higher when compared to the untreated one (). This indicates that NPs have enhanced the protein leakage by disrupting the bacterial membrane. This is in agreement with the results of Henie et al. (Citation2009) by bacterial membrane disruption and protein leakage by Psidium guajava leaf extracts.
Table 2. Quantification of protein leakage level in NPs treated bacterial species.
Morphological observation after treatment with AgNPs
The control cells of S. aureus appeared to be clear, cocci shaped without any change in the cell morphology, whereas the AgNPs treated cells showed membrane blebbing and distortion. The untreated cells of P. aeruginosa showed a rigid rod shaped structure. But the treated cells showed irregular shapes and rupture in bacterial cell wall (). A similar change in cell morphology was observed in AgNPs treated E. coli and S. aureus cells (Kim et al. Citation2011). This bactericidal effect may be due to binding of negative charge silver particles to the positive charge bacterial cell, causing cell membrane permeability and subsequently resulted in the leakage of the intracellular components causing cell death.
Figure 7. SEM observation of bacterial cells treated with AgNPs. The bacterial cells treated with AgNPs caused rupture of bacterial cell wall and shrinkage in cell shapes (Arrows indicate membrane blebbing and change in cell shapes). Whereas the untreated cells showed a rigid rod and cluster shaped cells without any changes on their surface.
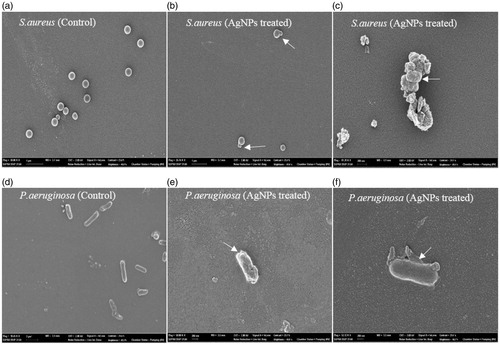
Conclusion
The present study reports the synthesis of AgNPs by using aqueous root extract of R. palmatum. The biosynthesis AgNPs were characterized by UV–Vis spectroscopy, XRD, TEM, and EDX. The particles are spherical and hexagonal shapes with an average size of 121.5 nm. The AgNPs showed excellent bactericidal effect against S. aureus and P. aeruginosa. Further, the possible antibacterial mechanism was studied by protein leakage and SEM studies. Thus the green synthesized AgNPs could be used as an alternative therapy to control the infection caused by S. aureus and P. aeruginosa.
Funding information
The authors would like to acknowledge their sincere appreciation to National Institute of Animal Science, RDA for its funding through the Research project NO (PJ010168).
Acknowledgements
We acknowledge Chang Hyun Lee, GBST, Seoul National University for his technical assistance.
Disclosure statement
The authors report no declaration of interest. The authors alone are responsible for the content and writing of the paper.
References
- Abou El-Nour KMM, Eftaiha AA, Al-Warthan A, Ammar RAA. 2010. Synthesis and applications of silver nanoparticles. Arab J Chem. 3:135–140.
- Aly MM, Gumgumjee NM. 2011. Antimicrobial efficacy of Rheum palmatum, Curcuma longa and Alpinia officinarum extracts against some pathogenic microorganisms. Afr J Biotechnol. 10:12058–12063.
- Arokiyaraj S, Arasu MV, Vincent S, Prakash NKU, Choi SH, Oh YK, Choi KC, Kim KH. 2014. Rapid green synthesis of silver nanoparticles from Chrysanthemum indicum L and its antibacterial and cytotoxic effects: an in vitro study. Int J Nanomedicine. 9:379–388.
- Bhattacharya D, Gupta RK. 2005. Nanotechnology and potential of microorganisms. Crit Rev Biotechnol. 25:199–204.
- Bradford MM. 1976. A rapid and sensitive method for the quantitation of microgram quantities of protein utilizing the principle of protein-dye binding. Anal Biochem. 72:248–254.
- Castanon MGA, Nino-Martinez N, Martinez-Gutierrez F, Martinez-mondeza JR, Ruiz F. 2008. Synthesis and antibacterial activity of silver nanoparticles with different size. J Nanopart Res. 10:1343–1348.
- Chitsazi MR, Korbekandi H, Asghari G, Najafi RB, Badii A, Iravani S. 2016. Synthesis of silver nanoparticles using methanol and dichloromethane extracts of Pulicaria gnaphalodes (Vent.) Boiss. Aerial parts. Artif Cells Nanomed Biotechnol. 44:328–333.
- Elia P, Zach R, Hazan S, Kolusheva S, Porat Z, Zeiri Y. 2014. Green synthesis of gold nanoparticles using plant extracts as reducing agents. Int J Nanomedicine. 9:4007–4402.
- Hatchett DW, Henry S. 1996. Electrochemistry of sulfur adlayers on the low-index faces of silver. J Phys Chem. 100:9854–9859.
- Henie EFP, Zaiton H, Suhaila M. 2009. Bacterial membrane disruption in food pathogens by Psidium guajava leaf extracts. Int Food Res J. 16:297–311.
- Irobi ON, Moo-Young M, Anderson WA, Daramola SO. 1994. Antimicrobial activity of bark extracts of Bridelia ferruginea (Euphorbiaceae). J Ethnopharmacol. 43:185–190.
- Jha AK, Prasad K, Prasad K, Kulkarni AR. 2009. Plant system: nature's nanofactory. Colloids Surf B Biointerfaces. 73:219–223.
- Kang SC, Lee CM, Choung ES, Bak JP, Bae JJ, Yoo HS, Kwak JH, Zee OP. 2008. Anti-proliferative effects of estrogen receptor-modulating compounds isolated from Rheum palmatum. Arch Pharm Res. 31:722–726.
- Kim BS, Song JY. 2010. Biological synthesis of gold and silver nanoparticles using plant leaf extract and antimicobial application in biocatalysis and biomolecular engineering. In: Ching TH, Shaw JF, Eds. Hoboken, NJ: Wiley, pp. 447–457.
- Kim HS, Lee HS, Ryu DS, Choi SJ, Lee DS. 2011. Antibacterial Activity of Silver-nanoparticles Against Staphylococcus aureus and Escherichia coli. J Microbiol Biotechnol. 39:77–85.
- Krishnaraj C, Jagan EG, Rajasekar S, Selvakumar P, Kalaichelvan PT, Mohan N. 2010. Synthesis of silver nanoparticles using Acalypha indica leaf extracts and its antibacterial activity against water borne pathogens. Colloids Surf B: Biointer. 76:50–56.
- Manivasagan P, Venkatesan J, Senthilkumar K, Sivakumar K, Kim SK. 2013. Biosynthesis, antimicrobial and cytotoxic effect of silver nanoparticles using a novel Nocardiopsis sp. MBRC-1. Bio Med Res Int. 2013:287638.
- Miyazawa M, Minamino Y, Kameoka H. 1996. Volatile components of the rhizomes of Rheum palmatum L. Flavour Frag J. 11:57–60.
- Padalia H, Moteriya P, Chanda S. 2015. Green synthesis of silver nanoparticles from marigold flower and its synergistic antimicrobial potential. Arab J Chem. 8:732–741.
- Pietta PG. 2000. Flavonoids as antioxidants. J Nat Prod. 63:1035–1042.
- Prakash P, Gnanaprakasam P, Emmanuel R, Arokiyaraj S, Saravanan M. 2013. Green synthesis of silver nanoparticles from leaf extract of Mimusops elengi, Linn. for enhanced antibacterial activity against multi drug resistant clinical isolates. Colloid Sur B Biointer. 108:255–259.
- Rejiniemon TS, Arasu MV, Duraipandiyan V, Ponmurugan K, Al-Dhabi NA, Arokiyaraj S, Agastian P, Choi KC. 2014. In-vitro antimicrobial, antibiofilm, cytotoxic, antifeedant and larvicidal properties of novel quinone isolated from Aegle marmelos (Linn.) Correa. Ann Clin Microbiol Antimicrob. 13:48.
- Rodríguez-León E, Iñiguez-Palomares R, Elena-Navarro R, Herrera-Urbina R, Tánori J, Iñiguez-Palomares C, Maldonado A. 2013. Synthesis of silver nanoparticles using reducing agents obtained from natural sources (Rumex hymenosepalus extracts). Nano Res Lett. 8:318.
- Savithramma N, Rao ML, Devi PS. 2011. Evaluation of antibacterial efficacy of biologically synthesized silver nanoparticles using stem barks of Boswellia ovalifoliolata Bal. and Henry and Shorea tumbuggaia Roxb. J Bio Sci. 11:39–45.
- Shankar SS, Ahmad A, Sastry M. 2003. Geranium leaf assisted biosynthesis of silver nanoparticles. Biotechnol Prog. 9:1627–1631.
- Sharma VK, Yngard RA, Lin Y. 2009. Silver nanoparticles: green synthesis and their antimicrobial activities. Adv Colloid Interface Sci. 145:83–96.
- Song JY, Jang HK, Kim BS. 2009. Biological synthesis of gold nanopaticles using Magnolia kobus and Diopyros kaki leaf extract. Proc Biochem. 44:1133–1138.
- Tambekar DH, Dahikar SB. 2011. Antibacterial activity of some Indian Ayurvedic preparations against enteric bacterial pathogens. J Adv Pharm Technol Res. 2:24–29.
- Umashankari J, Inbakandan D, Ajithkumar TT, Balasubramanian T. 2012. Mangrove plant, Rhizophora mucronata (Lamk, 1804) mediated one pot green synthesis of silver nanoparticles and its antibacterial activity against aquatic pathogens. Aquat Biosyst. 8:11.
- Vedpriya A. 2010. Living Systems:eco-friendly nanofactories. Dig J Nanomat Biostr. 5:9–21.
- Veerasamy R, Xin TZ, Gunasagaran S, Xiang TFW, Yang EFC, Jeyakumar N, Dhanaraj SA. 2010. Biosynthesis of silver nanoparticles using mangosteen leaf extract and evaluation of their antimicrobial activities. J Saudi Chem Soc. 15:113–120.
- Yoshikawa TT. 2002. Staphylococcus aureus infections and antibiotic resistance in older adults. Clin Infect Dis. 34:211–216.