Abstract
In the present work, the partitioning behavior of menadione-induced superoxide dismutase (SOD; EC 1.15.1.1), an antioxidant enzyme that has various applications in the medical and cosmetic industries, from the white rot fungus Phanerochaete chrysosporium has been characterized on different types of aqueous two-phase systems (ATPSs) (poly(ethylene glycol)/polypropylene glycol (PEG/PPG)-dextran, PEG-salt and PPG-salt). PEG-salt combinations were found most optimal systems for the purification of SOD. The best partition conditions were found using the PEG-3350 24% and K2HPO4 5% (w/w) with pH 7.0 at 25 °C. The partition coefficient of total SOD activity and total protein concentration observed in this system were 0.17 and 6.65, respectively, with the recovery percentage as 78.90% in the bottom phase and 13.17% in the top phase. The highest purification fold for SOD from P. chrysosporium was found as 6.04 in the bottom phase of PEG 3350%24 – K2HPO4%5 (w/w) system with pH 7.0. SOD purified from P. chrysosporium was determined to be a homodimer in its native state with a molecular weight of 60 ± 4 kDa. Consequently, simple and only one step PEG-salt ATPS system was developed for SOD purification from P. chrysosporium.
Introduction
The phenomenon of protein partitioning in aqueous two-phase systems (ATPSs) was first introduced in 1956 by the pioneering work of Albertsson (Citation1956). It has been established that ATPS forms when combinations of hydrophilic solutes (polymers or polymer and certain salts) display incompatibility in aqueous solution above their critical concentrations (Palomares Citation2004, Walter Citation2012). Generally speaking, the researchs in ATPS can be categorized into two main areas, including the elucidation of the mechanistic molecular understanding of solute partitioning in ATPS (Baskir et al. Citation1989, Graber et al. Citation2000, Kavakçıoğlu and Tarhan Citation2013, Lue and Blankschtein Citation1996, Zaslavsky Citation1994) and the practical implementation of the technique for the recovery of biological products from different sources (Antov et al. Citation2006, de Araújo et al. Citation2011, Gavasane and Gaikar Citation2003, Minami and Kilikian Citation1998, Nalinanon et al. Citation2009, Pan et al. Citation2001, Wu et al. Citation2001, Xu et al. Citation2002). It should be stated that due to the numerous, complex, and interrelated interactions among the system components and biomolecules, to get a general rule with respect to solute partition is not possible and desirable partition can be achieved with the manipulation of system by changing the composition, pH, and ionic strength. Although additional knowledge is needed to fully understand this phenomenon, it has been proved that practical application of ATPS for the recovery of biological products from different sources generates economic, robust, easy to scale and biocompatible extraction process with short process time and low-energy consumption (Hatti-Kaul Citation2001).
Superoxide dismutase (SOD) is the first line of antioxidant defence against reactive oxygen species (ROS) generated by respiration and, among eukaryotic organisms; it is the only enzyme that can detoxify superoxide (O2•‐) and prevents the formation of other more potent oxidants, such as peroxynitrite and hydroxyl radical (Bowler et al. Citation2001). It has been stated that the enzyme reduces fibrosis (Warner Citation1994) and plays an important roles in degenerative diseases, aging, and longevity (Nelson et al. Citation2005). Hence, from an industrial point of view, SOD has found wide usage in cosmetic and supplementary products. Besides, this enzyme is useful in prolonging the survival time of organs isolated for transplantation (Prieels et al. Citation1991), preservation of perishable materials, such as foodstuff, laundry compositions, for the removal of Amadori and Maillard products (Bafana et al. Citation2011), and also biosensors for (Tian et al. Citation2002). In fact, there are several techniques for SOD purification from various sources, including human lung, plants, different bacterial strains, rat testis and chicken liver as ultrafiltration, extraction with organic solvents and chromatography. But these techniques are time consumer and cause low recovery yield because the high number of step is required (Duke and Salin Citation1985, Hayakawa et al. Citation1985, Marklund Citation1982, Mruk et al. Citation1998, Öztürk-Ürek and Tarhan Citation2001, Spiegelhalder et al. Citation1993). The design and development of rapid, simple and more efficient bioprocesses for protein purification are needed because of the increasing demand to biomolecule production. ATPS is effective for the primary recovery of proteins, nucleic acids, low-molecular-weight compounds and cells, organelles and virus-like particles with the advantages, such as biocompatibility, low costs, ease to scale-up, rapid phase separation, easy manipulation, possibility for continuous operation, and suspended solids removal (Andrews and Asenjo Citation2010, Benavides et al. Citation2008). Therefore, aqueous two-phase systems (ATPS) is one of the alternative for the recovery of SOD. The only known research on ATPS partition of SOD was conducted by Simental-Martínez et al. (Citation2014). In this study, the highest purification fold was 4.0 ± 0.17 with 85.2 ± 3.5 U/mg for SOD from Kluyveromyces marxianus without considering ultrafiltration step.
In the present work, it was aimed to purify the SOD in the crude extract from Phanerochaete chrysosporium, known as a type of basidiomycete, has the ability of the abundant aromatic polymer lignindegradation, composting and removal of pentachlorophenol in soil (Chen et al. Citation2015a, Chen et al. Citation2015b, Fernandez-Fueyo et al. Citation2012, Martinez et al. Citation2014, Chen et al. Citation2016), after the induction of the enzyme activity with menadione by using different types of ATPS (ATPSs) (poly(ethylene glycol)/polypropylene glycol (PEG/PPG)-dextran, PEG-salt and PPG-salt) and manipulating the systems in terms of molecular weights of polymers, compositions of the components, pH and concentrations of neutral salts.
Materials and methods
Materials
PEG with average molecular masses of 1000, 2000, 3350, 6000, and 8000; PPG with average molecular masses of 425, 1000, 2700, and dextran with average molecular mass 100,000 g/mol were purchased from Sigma Chemical Co. (St. Louis, MO) and used without further purification. All other used chemical reagents were of analytical grade. Distilled and deionized water was used.
Methods
Media and growth conditions of P. Chrysosporium
Cultures of P. chrysosporium (German Collection of Microorganisms and Cell Cultures, DSMZ-1547) were maintained in potato dextrose agar (PDA) medium (pH 5.6) as described by Beever and Bollard (Citation1970). Sterilization of the medium was carried out by autoclaving at 121 °C for 20 min. Inoculation was performed at 28 °C for seven days in Petri dishes.
P. chrysosporium was cultured in modified Tien and Kirk liquid medium (Tien and Kirk Citation1984) (g/L); KH2PO4 (2.0), CaCl2 (0.114), MgSO4 (0.7), NH4Cl (0.12), glucose (2.0), thiamin-HCl (1.10−3), Tween 80 (0.05), FeSO4.7H2O (70 μg), ZnSO4.7H2O (46 μg), MnSO4·2H2O (35 μg); CoCl2.6H2O (7 μg). The liquid medium was sterilized by autoclaving at 121 °C for 20 min. Incubation was carried out at 28 °C for 10 days and with agitation at 150 rpm in the 250-mL erlenmayer flasks containing 90 mL liquid medium and 10 mL spore suspension (OD650; 0.500).
SOD activity induction with menadione as an exogenous factor
After 10 days culture, menadione (0.75 mM) was added to the growth medium that containing stationary phases P. chrysosporium in 250-ml Erlenmayer flask. Incubation was performed at 28 °C with agitation at 150 rpm for 6 h. The menadione-treated cells were harvested and washed several times with 20 mM potassium phosphate buffer (pH 7.4) at 4 °C, and then, crude extracts were prepared.
Preparation of crude extract from P. Chrysosporium
The harvested cells were resuspended in 20 mM potassium phosphate buffer (pH 7.4) in a volume equal to three times of the wet weight of the cells. The optimized homogenization procedure was performed for 3 min at 9000 rpm with 30-s interval. The cell debris in the homogenate was removed by centrifugation at 15,000 rpm and for 15 min at 4 °C. The crude extract was not frozen before use.
Superoxide dismutase activity assay
The SOD enzyme activity was determined by spectrophotometry at 490 nm. The SOD activity assay was based on the measurement of autoxidation of 6-hydroxydopamine (6-OHDA), which is inhibited by SOD (Crosti et al. Citation1987). One unit is the amount of SOD required to inhibit the initial rate of 6-OHDA autoxidation by 50%.
Total protein assay
Samples withdrawn from each phase were diluted 10-fold with a known amount of distilled water and total protein contents were measured with Bradford protein assay (Bradford Citation1976). BSA was used as a standard. An identically diluted solution of the corresponding phase from a system containing no catalase extract was used as a blank.
Preparation of aqueous two-phase systems
ATPSs were prepared by mixing required components at the desired pH in 15-mL graduated tubes. The final weight of the systems was adjusted to 5 g by the addition of double-distilled deionized water. Enzyme extract was added to the system as the final component to avoid possible precipitation and denaturation risks. The tubes were mixed by vortexing for 60 s to allow redistribution of the components. Complete phase separation was achieved by low-speed batch centrifugation at 2500 rpm for 5 min. The systems were left standing at 25 °C for 1 h after phase separation to reach equilibrium. The equilibrium state was characterized by the absence of turbidity in both the top and bottom phases. After the phases were carefully separated, both phases were appropriately diluted for the determination of SOD activities and total protein amounts. To correct for polymer and salt interference in the total protein assays, a blank system was prepared for each set of conditions, and the samples were read against the blanks. All partition experiments were conducted in triplicate, and the averages were used in the calculations.
Calculations of the partition parameters
The partition coefficient is described as the ratio of the total enzyme activity or protein concentration in the top phase to that in the bottom phase.
Ke = At/Ab, and Kp = Ct/Cb, where At and Ab are the total enzyme activities, and Ct and Cb are the total protein concentrations of the top and bottom phases, respectively.
The specific activity of the enzyme (SA, expressed in U/mg protein), the purification fold (PF), and the recovery (R) in the top and bottom phases were also calculated according to the given equations to evaluation efficiency of the systems:
SAt = At/Ct and SAb = Ab/Cb
PFt = (SA)t/(SA)i and PFb = (SA)b/(SA)i
Rt = At/Ai and Rb = Ab/Ai
Ai and SAi are the activity and the specific activity of the initial enzyme, respectively.
The partition experiments were carried out in triplicate, and the average results are the values reported in the present work.
Native molecular weight determination of superoxide dismutase from P. Chrysosporium
In order to determine the native molecular weight of SOD from P. chrysosporium, standard protein mixture instead of sample was applied to the sephadex G-100 gel filtration column (bed volume, 25 mL). The column was eluted (0.6 mL/min) with 0.15 MNaCl in 5 mM potassium phosphate buffer (pH 7.4). In the column, albumin (66 kDa), carbonic anhydrase (29 kDa), cytochrome c (12.4 kDa), and aprotinin (6.5 kDa) were used as molecular weight markers with blue dextran (200 kDa) for determining the void volume. The molecular weight for SOD was estimated from the calibration curve.
Electrophoretic procedures
SDS–PAGE of crude extract, top and bottom phases was performed according to the method of Laemmli and Favre (Citation1973). Protein solutions were mixed at 1:4 (v/v) ratio with the SDS–PAGE sample buffer (0.125 M Tris–HCl (pH 6.8), 4% SDS, 20% glycerol, 10% β-mercaptoethanol) and boiled for 5 min. The samples were loaded onto the gel made of 4% stacking and 12% separating gels and subjected to electrophoresis at a constant current of 150 V per gel using a Protean II Cell apparatus (Bio-Rad Laboratories, Richmond, CA). After electrophoresis, the gel was stained overnight with Coomassie Brilliant Blue R-250. Protein patterns were visualized after washing the gel with 40% (v/v) methanol and 10% (v/v) acetic acid solution for several times to remove Coomassie particles until clear background was observed. Spectra multicolor broad range protein ladders (10 to 260 kDa) were used as SDS–PAGE molecular weight standards.
Results and discussion
ATPSs provide a powerful method for the separation and purification of biomolecules mainly enzyme and proteins. In this study, it was aimed to characterize the partition behaviour of SOD from P. chrysosporium in terms of its Ke, Kp, PF, %R values for different types of ATPSs (PEG/PPG – dextran ≈100,000 g/mol, PEG-salt and PPG-salt). To induce the initial SOD activity in P. chrysosporium, cells were treated with menadione, which is known as a superoxide anion radical producer and consequently inducer of SOD, in the range of 0.1–0.75 mM for 1–8 h (Tongul and Tarhan Citation2014). The observed highest activity was found as 372 U/mg, with the treatment of 0.75 mM menadione at 6th h. Through this induction step, the initial activity of the SOD extract that would applied to ATPSs reached to 5.4-fold of control.
In the case of biomolecule partition/purification with ATPSs, the selection of phase system type and the investigation of certain parameters as optimal pH and ionic strength are significant steps. In order to select phase system type, different polymer – polymer and polymer – salt systems were examined in terms of their compatibility for selective partition of SOD (). As shown in , total enzyme activities in both top and bottom phases of polymer – salt systems were significantly higher in comparison with polymer – polymer systems. It was observed that SOD activity gradually decreased with the increases in molecular weights of PEG and PPG in both phases of the polymer – polymer systems. Consequently, PEG – dextran and PPG – dextran systems were not found suitable for the purification of SOD due to low recovery percentages compared to polymer – salt systems.
Table 1. Effects of molecular weight of PEG and system composition in PEG–K2HPO4 on menadione induced SOD partitioning at 25 °C
As is known to all, choosing the phase forming components types to the distributions of the target and residual biomolecules is the second most important step following the selection of the phase system type as polymer/salt (Hatti-Kaul Citation2001). Polymer/salt systems especially using sulfates or phosphates have proved particularly useful in sequential protein separation schemes because of their low cost and ease of handling. In this type of systems, two-phase formation is based on the reduction effect of the low-molecular-weight solute on polymer solubility in water. As stated earlier, phosphates and sulfates are the most appropriate and commonly used inorganic salts in enzyme purification with ATPS (Gautam and Simon Citation2006, Klomklao et al. Citation2005, Porto et al. Citation2008, Su and Chiang Citation2006, Yücekan and Önal Citation2011). On the other hand, it was stated in some papers that citrates could use as a substitute for inorganic salts because the fact that inorganic salts lead to high phosphate or sulfate concentrations in the effluent streams and therefore to environmental concern (Nitsawang et al. Citation2006). Unfortunately, we could not study with the citrate and sulfate salts due to problem encountered in the measurement of the enzyme activity because these salts hindered the autoxidation of 6-OHDA, the substrate of the SOD (data not shown). On the other hand, it was observed that viscosities of the PPG – salt systems were too high and this situation led to loss of SOD activity in a significant manner when compared with PEG – salt systems (). Additionally, PEG was selected as a polymer component of the polymer – salt systems because of having lower cost and approaching equilibrium easier in comparison with PPG.
In this research, the effects of the (1) molecular weight of PEG and composition of the system; (2) pH and finally (3) concentrations of neutral salts as an additional component on the distribution of menadione-induced SOD from P. chrysosporium in PEG – K2HPO4 systems between top and bottom phases were investigated.
Effects of PEG molecular weight and system composition on the partitioning behaviors of menadione-induced SOD from P. Chrysosporium
Although the mechanism governing the partition of biomolecules in ATPS is not fully understood, it is believed that the surface properties are probably the most important factors affecting the separation performance (Gautam and Simon Citation2006, Hatti-Kaul Citation2001, Klomklao et al. Citation2005, Nitsawang et al. Citation2006, Porto et al. Citation2008, Rahimpour and Baharvand Citation2009, Su and Chiang Citation2006, Yücekan and Önal Citation2011). The partitions of the target protein and the other molecules in the extract primarily depend on the PEG molecular weight and the system composition because these parameters influence the protein partition by changing the number of van der Waals, hydrophobic, hydrogen bond and ionic interactions. It was also emphasized in the study conducted by Qiang Lin et al. that among a broad array of the factors, the influence of phase-forming polymers should be concerned firstly, including polymer concentration and molecular weight (Han and Lee Citation1997). The effect of polymer concentration on biomaterial partitioning is obvious, and it is straightforward to design partitioning experiments to test trends with polymer concentration. However, the polymer molecular weights influence partitioning both by altering the phase diagram and by changing the polymer–protein interaction; thus, the testing of the trend with polymer molecular weight is less straightforward. Moreover, researchers cannot isolate the effect of polymer molecular weight from the effect of polymer concentration (Lin et al. Citation2003). Thereby, it can be concluded that these two factors should be considered simultaneously for modelling of protein partitioning in ATPS. Accordingly, in order to select a suitable molecular weight of PEG and also phase composition for the initial purification of menadione-induced SOD from P. chrysosporium, ATPSs were performed with five different molecular weights of PEG (MW; 1000, 2000, 3350, 6000, and 8000) and with different PEG and salt concentrations (3.5–22%) in the beginning of the research. Other parameters including phase-forming salt type, enzyme extract amount added to the systems and total system weights were kept constant. The pH values of the systems were the original and were about 9.5. The prepared systems were listed in and together with detailed results.
It is known that the miscibility of PEG below 1000 g/mol increases too much, which leads to higher concentrations for inducing a two-phase system. It was also emphasized by another group that when the smaller PEG size used, the more polymer is needed in order to enter into the two-phase region in the phase diagram (Forciniti et al. Citation1991). The another problem with small PEG sizes is low selectivity arising from contaminants that mainly accumulate in the top phase together with target biomolecule. Sharjahan et al stated that lower molecular weight PEG is not a good option for purification as the low molecular weight of the polymer may draw all proteins to the top phase that will cause poor separation and low purification factors of the target protein (Johansson et al. Citation2008). On the other hand, we also observed that molecular weights above 8000 g/mol caused to strongly increased viscosity which is not suitable for ATPS. From this point of view, molecular weights of PEG were chosen in the range of 1000–8000 g/mol.
In the beginning of the research, PEG 10%–K2HPO4 20% and PEG 15%–K2HPO4 15% systems were prepared with five different molecular weights of PEG as stated earlier. As can be seen from , while SOD activity in the polymer-rich top phase generally decreased with increasing concentration and also molecular weight of PEG, the separate comparison of each PEG molecular weight showed the activity increases in the salt rich bottom phase with decreasing K2HPO4 concentration. Theoretically, high Kp value along with high Ke indicates that most of proteins as well as target one were more partitioning to the top phase, while high Ke with low Kp implies that especially the target enzyme was more partitioned into the top phase. According to the results, while Ke values were close to 1 except the systems prepared by using 10% of PEG 1000 and both concentrations of PEG 6000 and 8000, Kp values were under 1 in all of the systems. Therefore, the results can be interpreted as that while target protein SOD almost equally distributed between top and bottom phases especially in the PEG 2000 and 3350 systems, total proteins predominantly accumulated in the bottom phase of all the systems. In addition, it was clear that both the target and total proteins predominantly distributed in the bottom phases of PEG 6000 and 8000 systems in particular. This situation can be the result of volume excluding effect of polymers with high molecular weights. On the other hand, that even the maximum Rb value of these PEG 6000 and 8000 systems was found as 23.28% clearly showed the denaturation effects, most likely due to the high salt concentrations.
As shown in , to prevent enzyme denaturation, the systems were prepared by lowering the salt concentrations from 15–20% to 3.5–8% and keeping the PEG amounts at minimum levels required to formation of two phases in the second step of the study. Unlike the systems presented in , Ke values were generally under 1 in all of the systems, which shows the bottom phase distribution of the target enzyme. According to the results, it was determined that while Ke values slightly decreased with increasing concentrations of polymer and decreasing concentrations of salt in the PEG 1000 and 2000 systems, the significant increases were observed in higher PEG molecular weights including 3350 and 6000 g/mol (). Although there are differences among the species, generally, the molecular weight of SOD is in the range of 30–70 kDa (Ali et al. Citation2014, Benavides et al. Citation2008, Lee et al. Citation1981, Moran et al. Citation2003). It can be thought that unlike the proteins with high molecular weights, relatively small protein SOD more preferred the top phase with increasing molecular weight and also concentration of PEG due to the hydrophobic interactions. However, the observed denaturation effect especially in PEG 6000 and PEG 8000 systems may be due to the high viscosity of these systems. On the other hand, when considered in terms of Kp values, it can be said that the selectivities of the initial systems improved by manipulating system composition. The maximum PFb was found as 1.51 in PEG 3350%20 – K2HPO4%5 system with the Ke of 0.19, Kp of 0.68, and Rb of 89.98%.
Table 2. Effects of molecular weight of PEG and system composition in PEG–K2HPO4 on menadione-induced SOD partitioning at 25 °C
Effects of PEG concentration and pH on the partitioning behaviors of menadione-induced SOD from P. Chrysosporium in PEG 3350 – K2HPO4%5 systems
Because the best result was obtained in the PEG 3350%20 – K2HPO4%5 system, this system was analysed further more in terms of polymer composition and pH under the conditions which salt amount was kept constant (). In these systems, pH values were adjusted to pH 7.5 ± 0.4 by using 1 M HCl or 1 M NaOH. As can be seen from , it can be said for all investigated systems that adjustment of pH values approximately to 7.5 caused to distribution of target enzyme almost equally between phases and consequently SOD activity increases in the top phase when compared with the systems in their original pH values. In addition, high Kp values along with low Ke were obtained via pH adjustment and also optimization of polymer concentration. It was observed that although SOD activities were slightly decreased in the bottom phase with the increasing percentages of polymer up to 24%, PEG 3350%24 – K2HPO4%5 – pH 7.67 system was found as the best system in terms of its PFb value of 3.39 due to high selectiveness with the Ke and Kp values of 0.74 and 5.15, respectively.
Table 3. Effects of PEG 3350 concentration and system pH on menadione induced SOD partitioning at 25 °C
In summary, it is obvious that (1) lowering pH values approximately to 7.5 resulted in increased SOD activities and accumulation of total proteins in the top phase (2) increasing PEG 3350 percentage up to 24% contributed the top-phase distribution of total proteins together with slight increases in Ke values. From all these points of view, PEG 3350%24 – K2HPO4%5 system was chosen for further optimization of the SOD purification in terms of pH. The chosen ATPS was prepared in the pH values ranging from 9.00 to 6.50. According to the results shown in , SOD activities gradually increased in the top phase of the systems up to pH 7.75 and redecreased in higher pH with the value of 36.19 U in pH 9.00. On the other hand, maximum SOD activity in the bottom phase was found for pH 7.00 system as 146.75 U. In addition, the best PFb as 6.04 was obtained in this system due to high selectivity to the target enzyme. It can be said that at neutral pH, SOD partition better behaviours compared to acidic or alkaline conditions. pH changed the partition behaviour of SOD as evidenced by lower purification folds on systems with pH 6.5 and 9.0 compared to pH 7.0. The assumption that as the pH increased above the isoelectric point (pI) of a protein, it became negatively charged, its interaction with PEG became stronger and the partition coefficient increased has been stated in numerous studies. However, that Ke values did not show increasing trend with the increase in pH from 6.5 to 9.0 may be the indication of dominant hydrophilicity of the target protein. This presumption was also supported in the study investigating the partition behaviour of glucose-6-phospate dehydrogenase (G6PDH) in ATPS (Johansson et al. Citation2008). The researchers stated that although the G6PDH was strongly negatively charged in studied conditions, Ke values were under 1 because hydrophilicity of the protein led to an effective repulsion from the PEG phase.
Table 4. Effects of system pH on menadione induced SOD partitioning at 25 °C
The selected PEG 3350%24 – K2HPO4%5 (pH 7.00) system was investigated for the evaluation of the effect of neutral salts (NaCl and KCl) on SOD partitioning. Adjustment of salt content in the system was made by addition of salts (solid form) into the system to obtain concentrations of 3, 5, 7, 9, and 11% (w/w). Unfortunately, the result of PF suggests that addition of neutral salts could not improve the partitioning of SOD in the bottom phase of the selected system (data not shown). Based on all these results, it was decided to choose PEG 3350%24 – K2HPO4%5 (pH 7.00) system as the optimized ATPS for the initial purification of P. chrysosporium superoxide dismutase.
Native molecular weight and protein pattern of Phanerochaete chrysosporium superoxide dismutase partitioned with ATPS
The molecular weight of the native form of the SOD was found to be 60 ± 4 kDa by using Sephadex G-100 gel filtration chromatography (). On the other hand, as seen from , bottom phase (lane 4) contained a major band with a molecular weight (MW) of 30 ± 2.5 kDa as the monomer subunit. It can be certainly said that although the crude P. chrysosporium extract contained various proteins having different molecular weights (line 2), considerable amount of contaminating proteins were eliminated after ATPS purification.
According to the results, the initial SOD activity of the crude extract from P. chrysosporium was induced with 0.75 mM menadione from about 69 to 372 U/mg and reached to 2246.88 U/mg with 6.04-fold purification by one step PEG 3350%24 – K2HPO4%5 (pH 7.00) system. When available literature has taken into consideration, there is only a paper on ATPS partition of SOD (Fernandez-Fueyo et al. Citation2012). In the study conducted by Simental-Martinez et al., SOD from clarified extract of K. marxianus was purified 4.0 ± 0.17-fold after PEG 3350 18.8% – phosphate 15% with 0.5 M NaCl ATPS fractionation and specific activity was reached to 85.2 ± 3.5 (Simental-Martínez et al. Citation2014). When these results were compared with our results, purification fold and the last specific activity were enhanced 1.51- and 26.4-fold.
On the other hand, in the researches on SOD purification by using traditional chromatographic steps, it was determined that initial SOD activities were range from 1.8 to 150.2 U/mg in the crude extracts from different resources such as human and mouse lung, rat liver, Neurospora crassa, Streptomyces spp., Escherichia coli, Helicobacter pylori, Crithidia fasciculata, Kluyveromyces marxianus, and piper betle leaf (Fattman et al. Citation2000, Fernandez-Fueyo et al. Citation2012, Hayakawa et al. Citation1985, Keele et al. Citation1970, Le Trant et al. Citation1983, Liu et al. Citation2015, Misra and Fridovich Citation1972, Mruk et al. Citation1998, Reiss and Gershon Citation1976, Youn et al. Citation1996). Menadione-induced SOD activity of the crude extract from P. chrysosporium was about 2.48-fold higher than the value observed in Streptomyces spp. extract which was the highest one among the investigated literature (Liu et al. Citation2015). Additionally, it should also be stated that even after the complex purification processes including two or more time-consuming chromatographic steps aiming at isolated SOD from bacteria as Escherichia coli, Helicobacter pylori, Nocardia asteroides, and Desulfovibrio gigas, fungi as Neurospora crassa and tissues as rat liver purified SOD activities were close or lower than ATPS purified SOD from P. chrysosporium (Beaman et al. Citation1983, Dos Santos et al. Citation2000, Johansson et al. Citation2008, Reiss and Gershon Citation1976, Spiegelhalder et al. Citation1993, Yost and Fridovich Citation1973). On the other hand, there were also outstanding results in the range of 10,930–64,200 U/mg but particularly in the limited number of researches using at least five or more chromatographic and required dialysis steps (Baum et al. Citation1983, Marklund Citation1982). In comparison, the ATPS developed in this study could remove the contaminant protein while concentrating SOD, omitting the steps of precipitation and chromatography used by the others to remove the contaminants.
Figure 1. (A) Elution profile of SOD from Sephadex G-100 column; (B) Molecular weight calibration curve; standard proteins (–•–) and ATPS purified SOD (–○–).
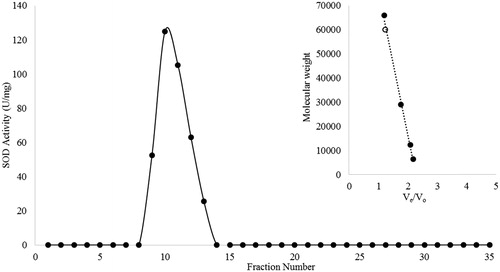
Figure 2. SDS–PAGE profiles of P. chrysosporium SOD purified by partitioning in ATPS. Lane 1: molecular weight markers (15–260 kDa) (10 μg), lane 2: top phase of the optimized system (25 μg), lane 3: crude SOD extract (25 μg) and lane 4: bottom phase of the optimized system (25 μg).
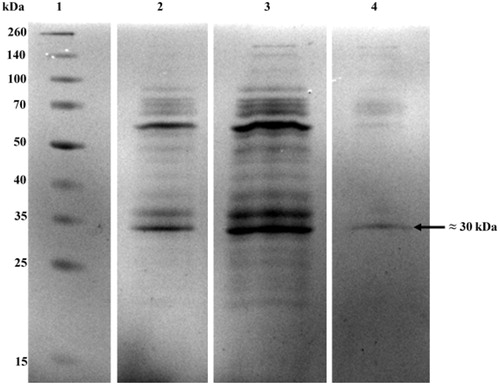
From these points of view, compared to many other purification studies, the results as 6.04 purification fold and 2246.88 U/mg last specific activity obtained from developed one step PEG-salt ATPS purification after menadione induction of SOD in P. chrysosporium were absolutely attractive.
Conclusions
The partitioning and purification of SOD from P. chrysosporium in various ATPSs have been reported in this paper. The optimized system for the purification of SOD was PEG 3350 24% – K2HPO4 5% without NaCl addition at pH 7.0 with Ke 0.17; Kp 6.65; Rb 78.90 and PFb 6.04. From the studied systems, it can be concluded for the partition of SOD in PEG–phosphate systems that high PF values in the bottom phases could be achieved by keeping K2HPO4 concentration low and pH close to neutral pH values. Addition of non-phase forming salt NaCl/KCl could not improve purification folds. The highest purification fold was obtained as 6.04 in the bottom phase of the optimized PEG 3350%24 – K2HPO4%5 (pH 7.00) system. Consequently, it can be certainly concluded that this novel approach can be used for the purification of SOD as an alternative method due to its efficiency when compared with traditional ones.
Disclosure statement
The authors report no conflicts of interest. The authors alone are responsible for the content and writing of this article.
References
- Albertsson PÅ. 1956. Chromatography and partition of cells and cell fragments. Nature. 177:771–774.
- Ali SM, Ling TC, Muniandy S, Tan YS, Raman J, Sabaratnam V. 2014. Recovery and partial purification of fibrinolytic enzymes of Auricularia polytricha (Mont.) Sacc by an aqueous two-phase system. Sep Purif Technol. 122:359–366.
- Andrews BA, Asenjo JA. 2010. Theoretical and experimental evaluation of hydrophobicity of proteins to predict their partitioning behavior in aqueous two phase systems: a review. Sep Sci Technol. 45:2165–2170.
- Antov MG, Peričin DM, Dašić MG. 2006. Aqueous two-phase partitioning of xylanase produced by solid-state cultivation of Polyporus squamosus. Process Biochem. 41:232–235.
- Bafana A, Dutt S, Kumar S, Ahuja PS. 2011. Superoxide dismutase: an industrial perspective. Crit Rev Biotechnol. 31:65–76.
- Baskir JN, Hatton TA, Suter UW. 1989. Protein partitioning in two phase aqueous polymer systems. Biotechnol Bioeng. 34:541–558.
- Baum JA, Chandlee JM, Scandalios JG. 1983. Purification and partial characterization of a genetically-defined superoxide dismutase (SOD-1) associated with maize chloroplasts. Plant Physiol. 73:31–35.
- Beaman BL, Scates SM, Moring SE, Deem R, Misra HP. 1983. Purification and properties of a unique superoxide dismutase from Nocardia asteroides. J Biol Chem. 258:91–96.
- Beever R, Bollard E. 1970. The nature of the stimulation of fungal growth by potato extract. J Gen Microbiol. 60:273–279.
- Benavides J, Aguilar O, Lapizco-Encinas BH, Rito-Palomares M. 2008. Extraction and purification of bioproducts and nanoparticles using aqueous two-phase systems strategies. Chem Eng Technol. 31:838–845.
- Bowler RP, Arcarolı J, Crapo JD, Ross A, Slot JW, Abraham E. 2001. Extracellular superoxide dismutase attenuates lung injury after hemorrhage. Am J Respir Crit Care Med. 164:290–294.
- Bradford MM. 1976. A rapid and sensitive method for the quantitation of microgram quantities of protein utilizing the principle of protein-dye binding. Anal Biochem. 72:248–254.
- Chen M, Qin X, Li J, Zeng G. 2016. Probing molecular basis of single-walled carbon nanotube degradation and nondegradation by enzymes based on manganese peroxidase and lignin peroxidase. RSC Adv. 6:3592–3599.
- Chen M, Xu P, Zeng G, Yang C, Huang D, Zhang J. 2015a. Bioremediation of soils contaminated with polycyclic aromatichydrocarbons, petroleum, pesticides, chlorophenols and heavy metals by composting: applications, microbes and future research needs. Biotechnol Adv. 33:745–755.
- Chen M, Zeng G, Lai C, Li J, Xu P, Wu H. 2015b. Molecular basis of laccase bound to lignin: insight from comparative studies on the interaction of trametes versicolor laccase with various lignin model compounds. RSC Adv. 5:52307–52313.
- Crosti N, Servidei T, Bajer J, Serra A. 1987. Modification of the 6-hydroxydopamine technique for the correct determination of superoxide dismutase. J Clin Chem Clin Biochem. 25:265–266.
- de Araújo RFF, Porto TS, Martins DBG, Dutra RF, Porto ALF, de Lima Filho JL. 2011. Partitioning of lactate dehydrogenase from bovine heart crude extract by polyethylene glycol–citrate aqueous two-phase systems. Fluid Phase Equilibr. 301:46–50.
- Dos Santos WG, Pacheco I, Liu MY, Teixeira M, Xavier AV, LeGall J. 2000. Purification and characterization of an iron superoxide dismutase and a catalase from the sulfate-reducing Bacterium Desulfovibrio gigas. J Bacteriol. 182:796–804.
- Duke MV, Salin ML. 1985. Purification and characterization of an iron-containing superoxide dismutase from a eucaryote, Ginkgo biloba. Arch Biochem Biophys. 243:305–314.
- Fattman CL, Enghild JJ, Crapo JD, Schaefer LM, Valnickova Z, Oury TD. 2000. Purification and characterization of extracellular superoxide dismutase in mouse lung. Biochem Bioph Res Co. 275:542–548.
- Fernandez-Fueyo E, Ruiz-Dueñas FJ, Ferreira P, Floudas D, Hibbett DS, Canessa P et al. 2012. Comparative genomics of Ceriporiopsis subvermispora and Phanerochaete chrysosporium provide insight into selective ligninolysis. Proc Natl Acad Sci. 109:5458–5463.
- Forciniti D, Hall CK, Kula MR. 1991. Influence of polymer molecular weight and temperature on phase composition in aqueous two-phase systems. Fluid Phase Equilibr. 61:243–262.
- Gautam S, Simon L. 2006. Partitioning of β-glucosidase from Trichoderma reesei in poly (ethylene glycol) and potassium phosphate aqueous two-phase systems: influence of pH and temperature. Biochem Eng J. 30:104–108.
- Gavasane MR, Gaikar VG. 2003. Aqueous two-phase affinity partitioning of penicillin acylase from E. coli in presence of PEG-derivatives. Enzyme Microb Tech. 32:665–675.
- Graber TA, Taboada ME, Cartón A, Bolado S. 2000. Liquid-liquid equilibrium of the poly (ethylene glycol) + sodium nitrate + water system at 298.15 K. J Chem Eng Data. 45:182–184.
- Han JH, Lee CH. 1997. Effects of salts and poly (ethylene glycol)-palmitate on the partitioning of proteins and Bacillus subtilis neutral protease in aqueous two-phase systems. Colloid Surface B. 9:109–116.
- Hatti-Kaul R. 2001. Aqueous two-phase systems. Mol Biotechnol. 19:269–277.
- Hayakawa T, Kanematsu S, Asada K. 1985. Purification and characterization of thylakoid-bound Mn-superoxide dismutase in spinach chloroplasts. Planta. 166:111–116.
- Johansson HO, Magaldi FM, Feitosa E, Pessoa A. 2008. Protein partitioning in poly(ethylene glycol)/sodium polyacrylate aqueous two-phase systems. J Chromatogr A. 1178:145–153.
- Kavakçıoğlu B, Tarhan L. 2013. Initial purification of catalase from Phanerochaete chrysosporium by partitioning in poly (ethylene glycol)/salt aqueous two phase systems. Sep Purif Technol. 105:8–14.
- Keele BB, McCord JM, Fridovich I. 1970. Superoxide dismutase from Escherichia coli B. A new manganese-containing enzyme. J Biol Chem. 245:6176–6181.
- Klomklao S, Benjakul S, Visessanguan W, Simpson BK, Kishimura H. 2005. Partitioning and recovery of proteinase from tuna spleen by aqueous two-phase systems. Process Biochem. 40:3061–3067.
- Laemmli UK, Favre M. 1973. Maturation of the head of bacteriophage T4. I. DNA packaging events. J Mol Biol. 80:575–599.
- Le Trant N, Meshnick SR, Kitchener K, Eaton JW, Cerami A. 1983. Iron-containing superoxide dismutase from Crithidia fasciculata. Purification, characterization, and similarity to Leishmanial and trypanosomal enzymes. J Biol Chem. 258:125–130.
- Lee YM, Ayala FJ, Misra HP. 1981. Purification and properties of superoxide dismutase from Drosophila melanogaster. J Biol Chem. 256:8506–8509.
- Lin DQ, Wu YT, Mei LH, Zhu ZQ, Yao SJ. 2003. Modeling the protein partitioning in aqueous polymer two-phase systems: influence of polymer concentration and molecular weight. Chem Eng Sci. 58:2963–2972.
- Liu YC, Lee MR, Chen CJ, Lin YC, Ho HC. 2015. Purification of Cu/Zn superoxide dismutase from Piper betle leaf and its characterization in the oral cavity. J Agr Food Chem. 63:2225–2232.
- Lue L, Blankschtein D. 1996. A liquid-state theory approach to modeling solute partitioning in phase-separated solutions. Ind Eng Chem Res. 35:3032–3043.
- Marklund SL. 1982. Human copper-containing superoxide dismutase of high molecular weight. Proc Natl Acad Sci USA. 79:7634–7638.
- Martinez D, Larrondo LF, Putnam N, Gelpke MDS, Huang K, Chapman J et al. 2014. Genome sequence of the lignocellulose degrading fungus Phanerochaete chrysosporium strain RP78. Nature Biotechnol. 22:695–700.
- Minami NM, Kilikian BV. 1998. Separation and purification of glucoamylase in aqueous two-phase systems by a two-step extraction. J Chromatogr B Biomed Sci Appl. 711:309–312.
- Misra HP, Fridovich I. 1972. The purification and properties of superoxide dismutase from Neurospora crassa. J Biol Chem. 247:3410–3414.
- Moran JF, James EK, Rubio MC, Sarath G, Klucas RV, Becana M. 2003. Functional characterization and expression of a cytosolic iron-superoxide dismutase from cowpea root nodules. Plant Physiol. 133:773–782.
- Mruk D, Cheng CH, Cheng YH, Mo MY, Grima J, Silvestrini B, Cheng CY. 1998. Rat testicular extracellular superoxide dismutase: its purification, cellular distribution, and regulation. Biol Reprod. 59:298–308.
- Nalinanon S, Benjakul S, Visessanguan W, Kishimura H. 2009. Partitioning of protease from stomach of albacore tuna (Thunnus alalunga) by aqueous two-phase systems. Process Biochem. 44:471–476.
- Nelson SK, Bose S, Rizeq M, McCord JM. 2005. Oxidative stress in organ preservation: a multifaceted approach to cardioplegia. Biomed Pharmacother. 59:149–157.
- Nitsawang S, Hatti-Kaul R, Kanasawud P. 2006. Purification of papain from Carica papaya latex: aqueous two-phase extraction versus two-step salt precipitation. Enzyme Microb Technol. 39:1103–1107.
- Öztürk-Ürek R, Tarhan L. 2001. Purification and characterization of superoxide dismutase from chicken liver. Comp Biochem Physiol B Biochem Mol Biol. 128:205–212.
- Palomares MR. 2004. Practical application of aqueous two-phase partition to process development for the recovery of biological products. J Chromatogr B. 807:3–11.
- Pan IH, Yao HJ, Li YK. 2001. Effective extraction and purification of beta-xylosidase from Trichoderma koningii fermentation culture by aqueous two-phase partitioning. Enzyme Microb Technol. 28:196–201.
- Porto TS, e Silva GM, Porto CS, Cavalcanti MTH, Neto BB, Lima-Filho JL, et al. 2008. Liquid–liquid extraction of proteases from fermented broth by PEG/citrate aqueous two-phase system. Chem Eng Process. 47:716–721.
- Prieels JP, Maschelein C, Heilporn M. U.S. Patent No. 5,010,007. Washington, DC: U.S. Patent and Trademark Office 1991.
- Rahimpour F, Baharvand AR. 2009. Phase equilibrium in aqueous two-phase systems containing poly (propylene glycol) and sodium citrate at different pH. World Acad Sci Eng Technol. 59:150–153.
- Reiss U, Gershon D. 1976. Rat-liver superoxide dismutase. Purification and age-related modifications. Eur J Biochem. 63:617–623.
- Simental-Martínez J, Rito-Palomares M, Benavides J. 2014. Potential application of aqueous two-phase systems and three-phase partitioning for the recovery of superoxide dismutase from a clarified homogenate of Kluyveromyces marxianus. Biotechnol Prog. 30:1326–1334.
- Spiegelhalder C, Gerstenecker B, Kersten A, Schiltz E, Kist M. 1993. Purification of Helicobacter pylori superoxide dismutase and cloning and sequencing of the gene. Infect Immun. 61:5315–5325.
- Su CK, Chiang BH. 2006. Partitioning and purification of lysozyme from chicken egg white using aqueous two-phase system. Process Biochem. 41:257–263.
- Tian Y, Mao L, Okajima T, Ohsaka T. 2002. Superoxide dismutase-based third-generation biosensor for superoxide anion. Anal Chem. 74:2428–2434.
- Tien M, Kirk T. 1984. Lignin-degrading enzyme from Phanerochaete chrysosporium: purification, characterization, and catalytic properties of a unique H2O2-requiring oxygenase. Proc Natl Acad Sci. 81:2280–2284.
- Tongul B, Tarhan L. 2014. The effect of menadione-induced oxidative stress on the in vivo reactive oxygen species and antioxidant response system of Phanerochaete chrysosporium. Process Biochem. 49:195–202.
- Walter H. Partitioning in aqueous two–phase system: theory, methods, uses, and applications to biotechnology. New York: Academic Press; 2012.
- Warner HR. 1994. Superoxide dismutase, aging, and degenerative disease. Free Radic Biol Med. 17:249–258.
- Wu YT, Pereira M, Venâncio A, Teixeira J. 2001. Separation of endo-polygalacturonase using aqueous two-phase partitioning. J Chromatogr A. 929:23–29.
- Xu Y, Vitolo M, Albuquerque CN, Pessoa A. 2002. Affinity partitioning of glucose-6-phosphate dehydrogenase and hexokinase in aqueous two-phase systems with free triazine dye ligands. J Chromatogr B Analyt Technol Biomed Life Sci. 780:53–60.
- Yost FJ, Fridovich I. 1973. An iron-containing superoxide dismutase from Escherichia coli. J Biol Chem. 248:4905–4908.
- Youn H, Kim E, Roe J, Hah Y, Kang S. 1996. A novel nickel-containing superoxide dismutase from Streptomyces spp. Biochem J. 318:889–896.
- Yücekan İ, Önal S. 2011. Partitioning of invertase from tomato in poly (ethylene glycol)/sodium sulfate aqueous two-phase systems. Process Biochem. 46:226–232.
- Zaslavsky BY. Aqueous two-phase partitioning: physical chemistry and bioanalytical applications. Boca Raton (FL): CRC Press; 1994.