Abstract
In this study, we reported folate-conjugated polypropylene imine dendrimers (FA–PPI) as efficient carrier for model anticancer drug, methotrexate (MTX), for pH-sensitive drug release, selective targeting to cancer cells, and anticancer activity. In the in vitro drug release studies this nanoconjugate of MTX showed initial rapid release followed by gradual slow release, and the drug release was found to be pH sensitive with greater release at acidic pH. The ex vivo investigations with human breast cancer cell lines, MCF-7, showed enhanced cytotoxicity of MTX–FA–PPI with significantly enhanced intracellular uptake. The biofate of nanoconjugate was determined in Wistar rat where MTX–FA–PPI showed 37.79-fold increase in the concentration of MTX in liver after 24 h in comparison with free MTX formulation.
Introduction
Dendrimers present a well defined three-dimensional polymeric scaffold, which is synthesized in a step-wise controlled manner by attaching branching units to a radiating focal point known as core (Jain and Jain Citation2014, Jain et al. Citation2014). Dendrimers have hyper-branched structure with multivalent surface functional groups which play important role in controlling the properties of dendrimers as well as in delivery of therapeutic moieties that has been encapsulated within or complexed with dendrimers. Large number of surface functional groups on dendrimer’s outer shell can be tailored or conjugated with a variety of guest molecules (Jain et al. Citation2013a, Citation2013b, Sharma et al. Citation2011). Poly(propylene)imine dendrimers (PPI) is an important member of dendrimers’ family reported first by de Brabander‐van den Berg and Meijer (Citation1993). At present, PPI dendrimers are commercially available as AstramolTM by DSM (Jain et al. Citation2010, Jain et al. Citation2015a, Kesharwani et al. Citation2014). The surface functionality can be “tailored” to attach a variety of drugs, genes, targeting agents, and imaging agents either by complexation or conjugation (Mout et al. Citation2012, Perumal et al. Citation2008). Surface-engineered/conjugated dendrimers, with drug molecules or targeting ligands or both, are receiving much attention as a promising carrier system and an alternative to the toxicity problems. Targeted anticancer drug delivery includes drug delivery by passive targeting and active targeting utilizing the enhanced permeability and retention (EPR) effect and tumor-specific ligand receptor interactions, respectively, avoiding reticulo-endothelial system (RES) (Maruyama Citation2011, Thakur et al. Citation2015). The major drawback with the anticancer drug delivery is that these indiscriminately attack healthy cells as well as cancerous cells. However, the harmful side effects due to such therapy can be reduced by developing a drug delivery system that is specific to tumor cells. To overcome this, a strategy called active targeting can be followed, wherein functionalities that respond to over-expressed receptors (e.g. folate conjugation on dendrimer surfaces) on tumor cells are attached to the drug carrier (Feng et al. Citation2013, Jain et al. Citation2015a, Jain et al. Citation2015b, Ryu and Raucher Citation2015).
Folic acid (FA) is a vital nutrient for the growth of cancer cells and can be used as a ligand due to high binding affinity and non-immunogenicity. Cell surface receptors of FA are over-expressed on many types of cancer cells. Folate binding protein, a glycosylphosphatidyl inositol (GPI) anchored cell surface receptor for folate, has been known to be over-expressed in several human tumors (Hu et al. Citation2014, Jain et al. Citation2014, Liu et al. Citation2012, Thomas et al. Citation2012).
In this study, we explored methotrexate (MTX)-loaded and folic acid-conjugated 5.0G PPI (MTX-FA-PPI) dendrimers for anticancer activity and controlled delivery of anticancer drug in ex vivo studies with human breast cancer cell lines MCF-7 and in vivo studies in Wistar rats.
Material and methods
Materials
The drug methotrexate (MTX) was obtained as a gift sample from Dabur, Rudrapur (India). Acrylonitrile, ethylene diamine (EDA), dimethyl sulphoxide (DMSO), folic acid, N,N-dimethylformamide (DMF) was purchased from Loba Chemie Pvt. Ltd., Mumbai (India). 4-Dimethylaminopyridine (DMAP), ethanolamine, and glacial acetic acid were purchased from SD Fine-Chem Limited, Mumbai (India). Dichloromethane (DCM) was purchased from Thames Chemicals, Ludhiana (Punjab, India). N-Hydroxysuccimide (NHS) and sodium tripolyphosphate (STPP) were purchased from Sigma Aldrich, Mumbai (India). Dialysis membrane (0.22 μm, 0.45 μm) was obtained from Sigma Chemical Co., St. Louis, MO.
Synthesis and characterization of 5.0G PPI dendrimers
Briefly, EDA cored PPI dendrimers up to 5.0G were synthesized using repetition of double Michael addition of acrylonitrile to primary amines, followed by heterogeneously catalyzed hydrogenation of the nitriles, resulting in doubling the number of primary amines (Jain et al. Citation2013b).
5.0G PPI dendrimer was characterized by IR and 1H NMR spectroscopy (Bagre et al. Citation2013, Gupta et al. Citation2010).
Optimization of 5.0G PPI dendrimers
Process variable, i.e. weight ratio of MTX and 5.0G PPI dendrimers were optimized on the basis of their effect on the particle size and polydispersity index ().
Table 1. Optimization parameters for 5.0G dendrimers.
Synthesis of MTX–PPI conjugates
Methotrexate was conjugated to PPI dendrimers following the previously reported method with slight modifications (Li et al. Citation2012, Thomas et al. Citation2012, Wu et al. Citation2006). Briefly, 5.0G PPI dendrimers dissolved in anhydrous DMF and mixed with solution of DMAP and NHS in anhydrous DMF followed by stirring for 36 h at room temperature. Then drug, MTX dissolved in DMF with TEA was added to the above reaction mixture with stirring for 24 h at room temperature followed by an addition of ethnolamine and mixing was continued for an additional period of 6 h. At the end of the reaction, this mixture was concentrated to ∼3 mL in volume in rotary vacuum evaporator and diluted with 50 mL of PBS pH 7.4. The solution was loaded into a membrane dialysis tubing (MWCO 10 kDa), and dialyzed against PBS pH 7.4. Then small amount of solution was taken in 1 cm standard cuvette after 15 min and absorbance of this solution was recorded at λmax 303 nm against PBS pH 7.4 as blank using UV-visible spectrophotometer (Shimadzu, UV-1700 series, Japan).
MTX–PPI conjugate was characterized by IR spectroscopy. In IR analysis, MTX–PPI conjugate was analyzed by the KBr pellet method in a PerkinElmer IR spectrophotometer (Waltham, MA).
Folic acid conjugation
Folic acid was conjugated with surface amino groups of 5.0G PPI dendrimers using EDC activated folic acid (Jain et al. Citation2014, Singh et al. Citation2008).
PPI–FA conjugate was characterized by IR and 1H NMR spectroscopy. In IR analysis, PPI–FA conjugate was analyzed by the KBr pellet method in a PerkinElmer IR spectrophotometer.
Synthesis of MTX–FA–PPI conjugate
MTX-FA-PPI conjugate was synthesized by conjugating MTX to free amino groups of FA-PPI conjugate using the procedure as described for the conjugation MTX to PPI dendrimers. The amount of drug conjugated to the amino groups of dendrimers was determined indirectly by measuring the amount of un-entrapped drug as described previously for MTX-PPI conjugate.
MTX–FA–PPI conjugate was characterized for various parameters like morphology by Scanning electron microscopy (SEM), size and polydispersity index using SEM and zeta sizer, FT-IR, 1H NMR spectroscopy, differential scanning calorimetry (DSC), entrapment efficiency and in vitro drug release (Chahal et al. Citation2013, Jain et al. Citation2014, Sunoqrot et al. Citation2012, Wang et al. Citation2015).
In vitro drug release studies
The in vitro drug release profile of MTX from the formulations (i.e. MTX–PPI, MTX–FA–PPI) were determined at two different pH conditions, in PBS pH 7.4 and acetate buffer pH 5.0 to simulate the pH of blood plasma and pH of interstitial spaces and endosomes in tumor, respectively, under perfect sink conditions. Drug content was estimated by UV-visible spectrophotometer (Shimadzu, UV-1700 series, Japan) using PBS pH 7.4 and acetate buffer pH 5.0, respectively, as control (Jain et al. Citation2014).
In vitro cytotoxicity assay and cancer cell growth inhibition
Cytotoxicity of developed formulations was determined againstMCF-7 human breast cancer cell lines purchased from National Center for Cell Sciences (NCCS), Pune, India. MCF-7 cells were seeded in 96-well microculture plates at 1 × 104 cells/well in Dulbecco’s Modified Eagle’s Medium (DMEM; HiMedia, Mumbai, India) supplemented with 10% heat inactivated fetal bovine serum (FBS; HiMedia, Mumbai, India) and incubated for 24 h at 37 ± 0.5°Cin 5% CO2 incubator. Formulations (MTX–PPI, MTX–FA–PPI, and MTX) were diluted to various concentrations in culture medium and incubated with MCF-7 cells for 24 h, respectively. Then percent cell growth inhibition was measured using MTT [3-(4,5-dimethylthiazol-2-yl)-2,5-diphenyltetrazoliumbromide, a yellow tetrazole] assay standard protocol.
Cellular uptake studies
Cellular uptake of developed dendrimeric carrier was determined following the reported procedure, with slight modifications. Briefly, MCF-7 cells were incubated with dendrimeric formulations (MTX–PPI, MTX–FA–PPI, and MTX) at 37 °C with 5% CO2 for 4 h. Then the medium was removed and cells were washed three times with PBS pH 7.4. The fluorescence due to uptake of formulation was visualized qualitatively (Olympus, CK X 41, Melville, NY).
In vivo studies
The anticancer activity and targeting efficiency of developed dendrimeric formulation was determined in Wister rats. In vivo studies comprised of two parts, i.e. pharmacokinetic studies to elucidate the release behavior and biodistribution studies to determine disposition of dendrimeric formulations of MTX in biological environment.
Animal
Wistar albino female rats (200–280 g) were used to study pharmacokinetics of various formulation of MTX, i.e. plain drug, MTX–PPI conjugate, and MTX–FA–PPI conjugate after i.v. administration. Animals were procured from Animal House of I.S.F. College of Pharmacy, Moga, India and were housed under standard laboratory conditions with free access to food and water. For pharmacokinetics study, animals were fasted overnight and 8 h after the dosing. Protocol for studies was approved by the Institutional ethical committee at I.S.F. College of Pharmacy, Moga, India. The experiments were conducted as per the CPCSEA (Committee for Prevention, Control and Supervision of Experimental Animals ISF/CPCSEA/ICEA/Meeting No. 14/2015/Protocol No. 260) guidelines.
Route of administration and study plan
Various organs concentration-time profile and pharmacokinetic parameters were obtained after i.v. administration of plain drug (i.e. MTX), MTX–PPI conjugate and MTX–FA–PPI conjugate. For pharmacokinetic studies, rats were divided into four groups, each group having three rats. Total 18 animals will be divided into three groups (n = 6 × 3 = 18) Wistar rats for pharmacokinetic study and drug-organ distribution study as described in .
Table 2. Experimental layout for pharmacokinetic and organ distribution studies.
In the pharmacokinetic study, first group was given intravenously the free drug (MTX) solution (in 0.9% sodium chloride) at a dose of 5.0 mg/kg body weight. Second and third groups were treated intravenously with MTX–PPI and MTX–FA–PPI formulations at a dose equivalent to free drug (5.0 mg/kg body weight), respectively. The Eppendorf was flushed with 0.3 mL of heparinized normal saline solution (100 IU) to prevent blood clotting. Samples were collected at different time intervals (i.e (0).1, 4, 8, 12, 24 h) by drawing blood from retro-orbital plexus of eye. The samples were centrifuged at 1000 rpm for 10 min to obtain a plasma supernatant for further analyses and amount of MTX was estimated by HPLC after loading this clear supernatant in HPLC system (Shimadzu, C18, Japan). Total amount of MTX was determined according to the previously reported method, with slight modifications (Jain et al. Citation2014).
For organ distribution, rats were sacrificed by cervical dislocation and organs were isolated. Organ samples were homogenized and immediately centrifuged (5000–10,000 rpm) for 10 min at an ambient temperature. After centrifugation, supernatant plasma was transferred into clean, fresh Eppendorf, and stored in a freezer (at –70 °C) until analysis. Samples collected from rats were analyzed using validated bio-analytical method and amount of drug in different organs were determined using calibration curve.
Statistical analysis
The statistical analysis of data was performed using analysis of variance (ANOVA) followed by Tukey’s multiple comparison test. The results were expressed as mean ± standard deviation and showing the number of repeats. A difference of P < 0.05 was considered as statistically significant. The survival analysis was done by Kaplan–Meier method using GraphPad Prism V5.01 (La Jolla, CA).
Results
Synthesis and characterization of 5.0G PPI dendrimers
Dendrimer synthesis was performed as reported earlier by DeBrabander-van den Berg and Meijer (1993) with some modifications. EDA was used as dendrimer core. Synthesis was monitored at each step (or generation) up to fifth generation (Figure S1 and S2). IR spectroscopy confirmed the synthesis of 5.0G PPI dendrimers. In IR analysis, 5.0G PPI dendrimers revealed peaks () at C–N stretching of CH2–NH2 (1111.68 cm−1), C–H stretching (2935.88 cm − 1), N–H bending vibrations of amine (1561.16 cm−1), and most importantly, N–H stretching of primary amine (3851.85 cm−1). Here, the full generation dendrimers displayed strong peak of –NH2 (3500–3100 cm−1).
Synthesis, characterization, and optimization of MTX–PPI conjugate
IR analysis of MTX–PPI conjugate revealed peaks () at C–N stretching (2247.73 cm−1), N–H bending (1554.15 cm−1), O–H stretching (2934.33 cm−1), and C–C stretching (1680.32 cm−1).
The size of 5.0G dendrimers was found to decrease from 24.73 ± 0.20 to 22.56 ± 0.20 nm on increasing the ratio of MTX from 2 to 10 with respect of 5.0G PPI dendrimers. 5.0G PPI dendrimers: MTX ratio 1:10 was considered optimum because it has appropriate size range (22.56 ± 0.20 nm) with minimum polydispersity index (0.17 ± 0.02) and also better drug loading efficiency (99.70 ± 0.01%).
Folic acid conjugation
Folic acid conjugated dendrimer (FA–PPI) was prepared and characterized through various spectroscopic studies (Singh et al. Citation2008). IR confirmed the synthesis of FA–PPI conjugate synthesis. IR spectrum of the PPI–FA () revealed important peaks at 711.24 cm−1 (aromatic C-H bending); 1018.25 cm−1 (ester unconjugated C = O stretching); 1606.56 (aromatic C = C bending and stretching due to attachment of folic acid). 1H NMR spectrum further confirmed the conjugation of folic acid to dendrimers as shown in .
Drug loading, entrapment efficiency, and formulation development
The drug carrying capacity of the formulation was determined through calculation of the quantity of drug loaded therein and hence determining the moles of the drug entrapped per mole of the dendrimer. Drug encapsulation was accomplished through equilibrium dialysis method. UV–visible spectrophotometry (Shimadzu, UV-1700 series, Japan) was used to confirm indirectly the drug loading in 5.0G PPI dendrimers as well as FA-PPI conjugates, which was evident from a slight shift in the λmax of MTX (303 nm). Percent drug encapsulation was measured indirectly by estimating the un-entrapped drug spectrophotometrically during equilibrium dialysis method. Percent MTX encapsulation in both, i.e. MTX–PPI and MTX–FA–PPI was found to be 99.72 and 98.60%, respectively. The mechanism of interaction between MTX and dendrimers was found to be mediated by hydrogen bond formation between carboxyl groups of MTX and abundant amine groups in the dendrimers. The higher drug loading in case of MTX–FA–PPI conjugate was possibly due to increased dendritic architecture of folic acid conjugated dendrimers (Jain et al. Citation2013b, Wu et al. Citation2006).
Characterization of folic acid conjugates
Morphology
MTX–FA–PPI conjugate was characterized for their shape and surface morphology using SEM. The SEM photographs revealed that the surface of MTX–FA–PPI conjugate were smooth, spherical in shape and anchoring of ligand on 5.0G dendrimers could also be appreciated from its surface. SEM images of MTX–FA–PPI conjugate are shown in .
Particle size and polydispersity index
The size and polydispersity index of MTX-FA-PPI conjugate were determined using zetasizer (Beckman Coulter, Delsa NanoC, Brea CA). These are graphically presented in .
FT-IR spectroscopy
The MTX–FA–PPI conjugate was characterized by IR spectroscopy. IR spectrum of MTX–FA–PPI conjugate was found to be concordant with the reference spectrum, as the observed peaks and reported peaks showed similarity as described in and .
Table 3. Reported and observed peaks of MTX–FA–PPI conjugate.
Differential scanning calorimetry
Differential scanning calorimetry (DSC) was performed to examine the thermal stability of MTX–FA–PPI conjugate. DSC curve of MTX-FA-PPI conjugate gave an endothermic peak at 56.67 °C and an exothermic peak at 79.84 °C (). These studies further strengthen the evidence that there is compatibility between the drug and the polymer and also the chance of entrapment of drug inside the dendrimeric formulation. The DSC studies support our rationale, as stability is the primary concern, which can affect the formulation in many ways. To achieve stability and compatibility between the drug and the polymer must be ensured which can be confirmed by the DSC (Bagre et al. Citation2013, Barbosa et al. Citation2014).
In vitro drug release study
In vitro drug release studies were carried out in two different media (i.e. PBS pH 7.4 and acetate buffer pH 5.0) for the formulations (i.e. MTX–PPI, MTX–FA–PPI). It was observed that the release of MTX from PPI and FA–PPI was comparatively slow at pH 7.4 in comparison with pH 5.0. A possible mechanism of delayed release of the drug molecule may be the encapsulation of the drug in the hydrophobic cavities of the dendrimer that act as a sink to retain the drug molecules for extended duration than the surface of dendrimer molecules. In case of MTX–PPI, approximately 98.13 ± 3.45% drug was released in PBS pH 7.4 at 72 h and 98.00 ± 3.67% drug was released in acetate buffer pH 5.0 at 1 h. While in the case of MTX–FA–PPI, the drug release was 90.94 ± 4.04% and 57.30 ± 3.76% in PBS pH 7.4 and acetate buffer pH 5.0, respectively ().
In vitro cytotoxicity assay and cancer cell growth inhibition
The cytotoxic activity of drug loaded nanoconjugate was determined using MTT assay after 24 h incubation of formulation with MCF-7 cells. Cytotoxicity of MTX, MTX–PPI conjugate, and MTX–FA–PPI conjugate was investigated with different MTX concentrations to elucidate the dose- and time-dependent anticancer activity of developed formulation in comparison with plain MTX formulation. As all the formulations revealed significant increase in cytotoxicity with an increase in concentration and time, hence a positive correlation was observed between the concentration/time and cytotoxicity. At the same concentration of MTX and incubation time, different dendrimeric formulations (MTX–PPI and MTX–FA–PPI) showed significantly higher cytotoxicity. For instance, at a highest tested dose of 10 μg/mL, MTX showed 15.52% cell viability after 24 h, respectively. The formulations MTX–PPI and MTX–FA–PPI showed higher cytotoxicity at corresponding concentration of MTX and incubation time in comparison with plain MTX formulation, i.e. 12.39% cell viability was observed with MTX–PPI and 8.42% cell viability was observed for MTX–FA–PPI formulation after incubation for 24 h, respectively, at a concentration of 10 μg/mL (). The 5 μg/mL MTX induced 48.61% MCF–7 cell death. Comparatively, MTX–FA–PPI exerts a higher cytotoxic effect than MTX on MCF–7 cells at concentrations above 5 μg/mL. Apparently, the IC50 value of 1.0 μg/mL for MTX–FA–PPI conjugate is obviously lower than that for the MTX indicating higher cytotoxicity of dendrimeric formulations toward MCF-7 cancer cells.
Cellular uptake
Cellular uptake assay was performed to assess the intracellular fate of PPI dendrimers, which showed that dendrimers are internalized by the cancer cells with enhanced uptake in case of MTX–PPI. Cellular uptake of formulations was determined after 4 h time intervals (). MTX–PPI and MTX–FA–PPI conjugate of MTX showed an increase in the cellular uptake by MCF-7 cells at 37 ± 0.5 °C as compared with plain MTX solution. Additionally, on the basis of results of fluorescence microscopy, cells incubated with MTX–FA–PPI conjugate showed greater fluorescence in comparison with MCF-7 cells incubated with MTX–PPI conjugate. Such explorations along with enhanced cytotoxicity against cancer cells and improved drug release profile could support the therapeutic potential of developed dendrimeric nanoconjugates.
Figure 11. (A) Qualitative uptake of (a) MTX, (b) MTX-PPI, and (c) MTX-FA-PPI conjugates and (B) quantitative uptake in MCF-7 cells; (FA) representing pre-incubation with folic acid.
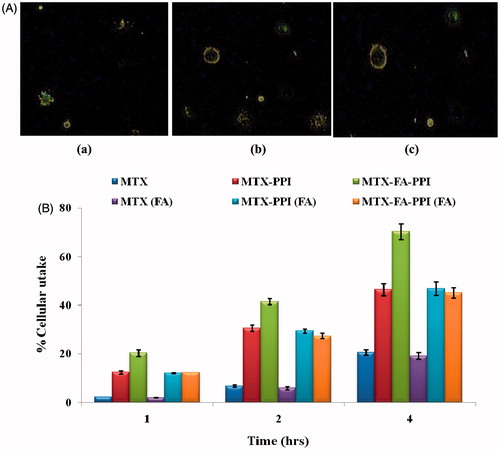
Cellular uptake studies showed that MTX–FA–PPI conjugate has maximum cellular uptake as compared to MTX–PPI conjugate. A high cellular uptake of ligand anchored dendrimers established the proposed hypothesis. The presence of folic acid improves the cellular efficiency of developed formulation for breast cells. Cellular uptake study further confirms that receptor mediated endocytosis is a key mechanism for cellular uptake of developed formulation.
Plasma pharmacokinetic study of MTX
Plasma drug concentration-time profile of MTX, MTX–PPI conjugate, and MTX–FA–PPI conjugate administered i.v. in Wistar rats is presented in and . This depicts graphical representation of plasma concentration of MTX at each time interval point, which indicates that highest plasma concentration was obtained from MTX-FA-PPI conjugate formulation. From the given results, it was observed that the developed optimized formulation showed increased plasma concentration (4.53 μg/mL) and t1/2 (9.97 h) than the MTX–PPI conjugate and plain drug. Further, the plasma concentration takes longer time to reach its maximum value in case of MTX–FA–PPI conjugate followed by MTX–PPI conjugate and plain drug showing sustained release in case of MTX–FA–PPI conjugate.
Table 4. Plasma pharmacokinetic parameters of different formulations.
This could be attributed to preferential localization of polymer MTX–FA–PPI conjugate in liver. Increase in Cmax value could be attributed to increased bioavailability of developed formulation, controlled release mechanism, and retention of developed formulation near the absorption window. Further, a higher tmax in developed formulation is associated with its high affinity for hepatic cell lines. Also, the localization of folate receptor along the sinusoid cell lines improves hepatic permeability and retention of the developed formulation in the liver.
Organ distribution study of MTX after i.v. administration
Organ distribution studies were carried out for MTX–FA–PPI conjugate, MTX–PPI conjugate, and plain drug. represents percent drug in different organs with respect to time. From the given data it is concluded that the amount of drug uptake in liver is more as compared to other organs. The MTX–FA–PPI conjugate increases the uptake of drug in the liver by 5.15-fold more than plain drug. So it can be attributed that FA has ligand properties for the receptors represents in the liver, so can be used for the liver targeting, as well it is efficiency against hepatotoxicity.
Discussion
Nanocarriers are presenting a promising approach for controlled delivery of chemotherapeutic agents. At present, dendrimers, carbon nanotubes, and quantum dots are being investigated for controlled delivery of anticancer drugs (Bajwa et al. Citation2015, Jain et al. Citation2015c, Mehra et al. Citation2015, Sharma et al. Citation2015). In this research work, we have explored FA-conjugated dendrimers for the delivery of anticancer drug, methotrexate (Figure S3). Dendrimers were prepared by a divergent method and characterized by IR spectroscopy. Then these were optimized by using a process variable, i.e. weight ratio of MTX and 5.0G PPI dendrimers on the basis of their effect on the particle size and loading efficiency. The three formulations were prepared in the ratio, i.e. 1:2, 1:4 and 1:10. MTX and 5.0G PPI dendrimers ratio 1:10 was considered optimum because it has appropriate size range (22.56 ± 0.20) for dendrimers with minimum polydispersity index (0.17 ± 0.02) and better drug entrapment efficiency (99.70 ± 0.01). The MTX–PPI conjugate was prepared and characterized by IR spectroscopy, particle size, and in vitro release study. Then MTX–FA–PPI conjugate was prepared and characterized by morphology, size, polydispersity index, FT-IR spectroscopy, differential scanning calorimetry (DSC), and in vitro drug release studies.
The MTX–FA–PPI conjugate was characterized for their shape and surface morphology using SEM. The SEM photographs revealed that the surface of this conjugate is smooth, spherical in shape, and anchoring of ligands on 5.0G dendrimers could also be appreciated from its surface. Measurement of particle size revealed higher size of MTX–FA–PPI conjugate (78.1 nm) as compared to PPI conjugate (22.4 nm) with polydispersity index (PDI) of 0.345 and 0.169, respectively via dynamic light scattering (DLS) measurements (Beckman coulter, Delsa NanoC). The data from DLS measurements revealed the increase in particle size with folate conjugation.
In IR analysis, the full generation dendrimers (5.0G) displayed strong peak of –NH2 (3500–3100 cm−1). MTX–FA–PPI conjugate displayed a peak corresponding to amide bond (–CO–NH–) formed between carboxyl group of folic acid and amine group of 5.0G PPI dendrimers was observed at 1659.46 cm − 1 confirming the interaction between amine group of dendrimers and carboxyl group of folic acid. 1H NMR spectrum showed the peaks for the presence of MTX and FA on the surface of dendrimers.
The DSC was performed to determine the transformation in crystallinity and thermal stability over a range of temperatures. PPI dendrimers, MTX, MTX-PPI, and MTX-FA-PPI conjugate and physical mixture of drug and dendrimers were examined by this study. DSC thermograms of MTX–FA–PPI conjugate displayed endothermic and exothermic peaks. These peaks represented the sharp melting point of MTX–FA–PPI conjugate. The broad endothermic peaks and absence of sharp peaks in both conjugate indicates the change in phase transition of MTX in developed formulation. Further, absence of sharp peaks clearly resolved the loss in crystalline stage of MTX in prepared formulation. The results of DSC studies showing the formation of MTX-PPI conjugate (Bagre et al. Citation2013, Barbosa et al. Citation2014, Gajbhiye et al. Citation2013).
The entrapment efficiency of MTX from MTX–FA–PPI conjugate was estimated and repeated thrice. All the formulations showed good reproducible results. In vitro drug release study was performed in acetate buffer pH 5.0 and PBS pH 7.4 in which the conjugate (MTX-FA-PPI) showed sustained release profile. Further, the release was more sustained at physiological pH 7.4 as compared to acidic pH 5.0. Hence, dendrimeric carrier is capable of sustaining the drug release in systemic circulation with faster release rate at acidic pH revealing that once the system enters the microvascular environment of tumor site, it will quickly release the drug at the target site, i.e. tumor. This faster release of drug in acidic environment could possibly be due to protonation of amine groups of dendrimer leading to opening of compact structure of dendrimer and solubility of drug at acidic pH.
The in vitro cytotoxicity activity of MTX–FA–PPI (containing optimized drug and polymer concentration which were cytotoxic) on MCF-7 cancer cell line was studied according to percentage reduction in cell viability. The IC50 value of MTX–FA–PPI and MTX–PPI conjugate formulation was found to be reduced by 1.8 and 1.2-fold, respectively, in comparison with plain MTX solution; indicating higher cytotoxicity of dendrimeric formulations toward MCF-7 cancer cells. The results of cytotoxicity testing suggest the potential therapeutic benefit of dendrimeric conjugates of MTX in MCF-7 cell lines. Our results are in accordance with the previous reports wherein nanocarriers showed increase in cytotoxicity of anticancer drug toward cancer cells.
MTX–FA–PPI nanoconjugate showed significant increase in the intracellular accumulation of MTX in MCF-7 cells in comparison with MTX–PPI dendrimeric carrier. The enhanced uptake of MTX–FA–PPI conjugate in comparison with MTX–PPI conjugates by MCF-7 cells might be due to additional endocytosis by the folate receptors. Thus, it can be proved that caveolin assisted receptor mediated endocytosis facilitates the internalization of the nanocarriers and thus MTX–FA–PPI conjugate is most suitable to provide more and sustained therapeutic concentrations in the site.
In vivo anticancer studies were performed for various formulations. The plasma concentration time profile of MTX, MTX–PPI conjugates, and MTX–FA–PPI were prepared and various pharmacokinetic parameters calculated from this plasma concentration versus time curve. The Cmax and AUCtotal for MTX were found to be 2.54 and 19.24, respectively, whereas for MTX–FA–PPI conjugate was found to be 4.53 and 52.70, respectively. So the folate conjugated dendrimeric formulation of MTX showed around 2- and 3-fold increase in the maximum concentration of drug (Cmax) and area under the curve (AUC), respectively, as compared to plain MTX solution. The biological half life (t1/2) and mean residence time (MRT) were found to increase from 5.66 and 7.56 to 9.97 and 52.70 h, respectively, with MTX–FA–PPI conjugate in comparison with plain MTX. The significant increase in the AUC, Cmax, t1/2, and MRT clearly depicts the improved bioavailability of MTX with MTX–FA–PPI conjugate, suggesting folate conjugated polypropylene imine dendronized nanoconjugate of MTX as a promising nanocarrier for controlled delivery of anticancer agents. Further, biodistribution studies were also performed to determine the targeting efficiency of MTX–FA–PPI conjugate formulation and it was observed that in case of free drug solution, drug was accumulated into the liver followed by kidney. MTX–PPI conjugate formulation increased the accumulation of drug in liver by approximately 6.3-, 12.7-, 17.4-, 23.4- and 33-fold after 1, 4, 8, 12 and 24 h, respectively, which could be ascribed to dendrimer-mediated enhanced penetration of MTX into liver. In contrast to plain drug solution, significant concentration of drug was observed in liver with MTX–FA–PPI conjugate formulation even after 24 h. MTX–FA–PPI conjugate formulation enhanced the amount of drug in liver tissue by about 10.5-, 17-, 22-, 29.7- and 37.7-fold in comparison with plain MTX formulation after 1, 4, 8, 12 and 24 h, respectively. From biodistribution studies it is clear that the folate-conjugated dendrimers are able to deliver selectively higher concentration of drug to liver in comparison with plain drug formulation owing to enhanced permeation and retention (EPR) effect as well as active targeting via receptor mediated endocytosis.
Nanomaterials like dendrimers are presenting a new horizon for modified drug delivery systems as well as for prophylactic treatment (Jain and Jain Citation2013, Citation2015, Jain et al. Citation2015c). In this study, PPI dendrimers have shown promising potential for controlled and targeted delivery of anticancer drug.
Conclusion
In conclusion, developed formulation, i.e. MTX–FA–PPI found to show controlled delivery of anticancer agent. The conjugates showed pH-responsive drug release systems, which could enable pH-controlled activation of the MTX in buffering environment in lysosomes of tumor cells. The cell line studies revealed promising anticancer and cancer targeting propensity of developed dendronized nanoconjugate. The cell line studies performed on MCF–7 showed that both the conjugates have low cytotoxicity. Further, anticancer efficiency studies demonstrated that the conjugates can increase the anticancer activity of MTX by increasing the drug delivery efficiency into cells. Cell uptake studies elucidated that the formulations were selectively taken up by the tumor cells in comparison with free drug. In the pharmacokinetic and biodistribution studies in Wistar rats, developed nanoconjugate exerted sustained release with improved pharmacokinetic profile and preferential deposition into liver tissue, respectively. Thus, the MTX–FA–PPI formulation can attack on cancerous tissue that could proved to be a promising strategy to treat a deadly ailment, cancer.
Supporting_material_revised.docx
Download MS Word (803.1 KB)Disclosure statement
The authors report no conflicts of interest related to this manuscript. The authors alone are responsible for the content and writing of the paper.
References
- Bagre AP, Jain K, Jain NK. 2013. Alginate coated chitosan core shell nanoparticles for oral delivery of enoxaparin: in vitro and in vivo assessment. Int J Pharm. 456:31–40.
- Bajwa N, Mehra NK, Jain K, Jain NK. 2015. Pharmaceutical and biomedical applications of quantum dots. Artif Cells Nanomed Biotechnol. [Epub ahead of print]. doi: 10.3109/21691401.2015.1052468.
- Barbosa JA, Zoppi A, Quevedo MA, de Melo PN, de Medeiros AS, Streck L, et al. 2014. Triethanolamine stabilization of methotrexate-β-cyclodextrin interactions in ternary complexes. Int J Mol Sci. 15:17077–17099.
- Chahal S, Hussain FSJ, Yusoff MBM. 2013. Characterization of modified cellulose (Mc)/poly (vinyl alcohol) electrospun nanofibers for bone tissue engineering. Proc Eng. 53:683–688.
- De Brabander‐van den Berg E, Meijer E. 1993. Poly(propylene imine) dendrimers: large‐scale synthesis by hetereogeneously catalyzed hydrogenations. Ange Chemie Int Edn Eng. 32:1308–1311.
- Feng D, Song Y, Shi W, Li X, Ma H. 2013. Distinguishing folate-receptor-positive cells from folate-receptor-negative cells using a Fluorescence off-on nanoprobe. Anal Chem. 85:6530–6535.
- Gajbhiye V, Ganesh N, Barve J, Jain NK. 2013. Synthesis, characterization and targeting potential of zidovudine loaded sialic acid conjugated-mannosylated poly(propyleneimine) dendrimers. Eur J Pharm Sci. 48:668–679.
- Gupta U, Dwivedi SKD, Bid HK, Konwar R, Jain N. 2010. Ligand anchored dendrimers based nanoconstructs for effective targeting to cancer cells. Int J Pharm. 393:186–197.
- Hu D, Sheng Z, Fang S, Wang Y, Gao D, Zhang P, et al. 2014. Folate receptor-targeting gold nanoclusters as fluorescence enzyme mimetic nanoprobes for tumor molecular colocalization diagnosis. Theranostics. 4:142–153.
- Jain A, Jain K, Kesharwani P, Jain NK. 2013a. Low density lipoproteins mediated nanoplatforms for cancer targeting. J Nanopart Res. 15:1–38.
- Jain A, Jain K, Mehra NK, Jain NK. 2013b. Lipoproteins tethered dendrimeric nanoconstructs for effective targeting to cancer cells. J Nanopart Res. 15:2003.
- Jain K, Gupta U, Jain NK. 2014. Dendronized nanoconjugates of Lysine and folate for treatment of cancer. Eur J Pharm Biopharm. 87:500–509.
- Jain K, Jain NK. 2013. Novel therapeutic strategies for treatment of visceral leishmaniasis. Drug Discov Today. 18:1272–1281.
- Jain K, Jain NK. 2014. Surface engineered dendrimers as antiangiogenic agent and carrier for anticancer drug: dual attack on cancer. J Nanosci Nanotechnol. 14:5075–5087.
- Jain K, Jain NK. 2015. Vaccines for visceral leishmaniasis: a review. J Immunol Methods. 422:1–12.
- Jain K, Kesharwani P, Gupta U, Jain N. 2010. Dendrimer toxicity: let's meet the challenge. Int J Pharm. 394:122–142.
- Jain K, Mehra NK, Jain NK. 2015c. Nanotechnology in drug delivery: safety and toxicity issues. Curr Pharm Des. 21:4252–4261.
- Jain K, Verma AK, Mishra PR, Jain NK. 2015a. Surface engineered dendrimeric nanoconjugates for macrophage targeted delivery of amphotericin B: formulation development, in vitro and in vivo evaluation. Antimicrob Agents Chemother. 59:2479–2487.
- Jain K, Verma AK, Mishra PR, Jain NK. 2015b. Characterization and evaluation of amphotericin B loaded MDP conjugated poly(propylene imine) dendrimers. Nanomedicine. 11:705–713.
- Kesharwani P, Jain K, Jain NK. 2014. Dendrimer as nanocarrier for drug delivery. Prog Polym Sci. 39:268–307.
- Li MH, Choi SK, Thomas TP, Desai A, Lee KH, Kotlyar A, Holl MMB, Baker JR. 2012. Dendrimer-based multivalent methotrexates as dual acting nanoconjugates for cancer cell targeting. Eur J Med Chem. 47:560–572.
- Liu L, Zheng M, Renette T, Kissel T. 2012. Modular synthesis of folate conjugated ternary copolymers: polyethylenimine-graft-polycaprolactone-block-poly (ethylene glycol)-folate for targeted gene delivery. Bioconjugate Chem. 23:1211–1220.
- Maruyama K. 2011. Intracellular targeting delivery of Liposomal drugs to solid tumors based on EPR effects. Adv Drug Deliv Rev. 63:161–169.
- Mehra NK, Jain K, Jain NK. 2015. Pharmaceutical and biomedical applications of surface engineered carbon nanotubes. Drug Discov Today. 20:750–759.
- Mout R, Moyano DF, Rana S, Rotello VM. 2012. Surface functionalization of nanoparticles for nanomedicine. Chem Soc Rev. 41:2539–2544.
- Perumal OP, Inapagolla R, Kannan S, Kannan RM. 2008. The effect of surface functionality on cellular trafficking of dendrimers. Biomaterials. 29:3469–3476.
- Ryu JS, Raucher D. 2015. Elastin-like polypeptide for improved drug delivery for anticancer therapy: preclinical studies and future applications. Expert Opin Drug Deliv.12:653–667.
- Sharma A, Gautam SP, Gupta AK. 2011. Surface modified dendrimers: synthesis and characterization for cancer targeted drug delivery. Bioorg Med Chem. 19:3341–3346.
- Sharma P, Mehra NK, Jain K, Jain NK. 2015. Biomedical application of carbon nanotubes: a critical review. Curr Drug Deliv. 12: Epub ahead of print.
- Singh P, Gupta U, Asthana A, Jain NK. 2008. Folate and folate − PEG − PAMAM dendrimers: synthesis, characterization, and targeted anticancer drug delivery potential in tumor bearing mice. Bioconjugate Chem. 19:2239–2252.
- Sunoqrot S, Liu Y, Kim DH, Hong S. 2012. In vitro evaluation of dendrimer-polymer hybrid nanoparticles on their controlled cellular targeting kinetics. Mol Pharm. 10:2157–2166.
- Thakur S, Kesharwani P, Tekade RK, Jain NK. 2015. Impact of PEGylation on biopharmaceutical properties of dendrimers. Polymer. 12:612–620.
- Thomas TP, Huang B, Choi SK, Silpe JE, Kotlyar A, Desai AM, et al. 2012. Polyvalent dendrimer-Methotrexate as a folate receptor-targeted cancer therapeutic. Mol Pharm. 9:2669–2676.
- Wang CF, Makila EM, Kaasalainen MH, Hagström MV, Salonen JJ, Hirvonen JT, Santos HA. 2015. Dual-drug delivery by porous silicon nanoparticles for improved cellular uptake, sustained release, and combination therapy. Acta Biomaterial. 16:206–214.
- Wu G, Barth RF, Yang W, Kawabata S, Zhang L, Green-Church K. 2006. Targeted delivery of methotrexate to epidermal growth factor receptor-positive brain tumors by means of cetuximab (IMC-C225) dendrimer bioconjugates. Mol Cancer Ther. 5:52–59.