Abstract
Hydrophobic drugs can absorb as guest molecules inside the cavity of cyclodextrins as host sites. So, forming the drug-cyclodextrin complex can exert a profound effect on the physicochemical and biological properties of the drugs. According to these advantages, in this study, we synthesized the tamoxifen (TMX) loaded cyclodextrin (CD)-conjugated MNPs to evaluate simultaneously the cytotoxicity and sustained release as well as hepatoprotective effect of this nanomedicine. The average size of Fe3O4-DPA-PEG-CD-TMX NPs was approximately 31 nm. By energy-dispersive X-ray spectroscopy (EDS), it was revealed that Fe3O4 constitutes 14.34% of the composition of modified MNPs. In the other words, nearly 85% of Fe3O4-DPA-PEG-CD NPs are made of dopamine (DPA), polyethylene glycol (PEG) and β-cyclodextrin (β-CD). The TMX loaded MNPs (with entrapment efficiency of 33 mg TMX per unit CD (mg) and loading efficiency of 87.5%) showed sustained liberation of TMX molecules (with 91% release in 120 h). Cytotoxicity assay and apoptosis assay by TUNEL analysis revealed that the engineered Fe3O4-DPA-PEG-CD-TMX NPs were able to significantly inhibit the MCF-7 breast cancer cells. According to effect of CD on TMX sustained release, it was found that CD can decrease the hepatotoxicity induced by TMX nearly 30%. Based upon these findings, we suggest the Fe3O4-DPA-PEG-CD-TMX NPs as an effective multifunctional nanomedicine with simultaneous therapeutic and hepatoprotective effects.
Introduction
Cyclodextrins (CDs) that are a group of cyclic starches with six (α), seven (β), or eight (γ) 1,4-α-linked glucose units are widely utilized as drug hosts with good biocompatibility (Tonelli Citation2014). The β-cyclodextrins (β-CDs) like other CDs have a truncated cone-like three-dimensional structure with a hydrophilic outer surface and a hydrophobic inner surface (Cai et al. Citation2011). So, hydrophobic drugs can absorb as guest molecules inside the cavity of CDs as host sites (Wu et al. Citation2009). One of the insoluble drugs with widespread use in all stages of breast cancer is tamoxifen (TMX). Increase blood clotting, retinopathy, liver cancer, hyperplasia, and corneal opacities are some side effects associated with TMX dose that can be related to the drug insolubility. Therefore, use of hosting nanocarriers (NCs) can increase TMX circulation that its result can be in minimal side effects (Heidari Majd et al. Citation2013c, Memisoglu-Bilensoy et al. Citation2005).
Previously, TMX has been delivered by the various nanoparticles (NPs) such as polyester nanosponge particles (Lockhart et al. Citation2015) or nanocapsules of β-CDC6 (Memisoglu-Bilensoy et al. Citation2005). Among various smart drug delivery nanosystems (e.g., nanoliposomes, polymeric NPs, dendrimers, and other organic/inorganic NPs) used for targeting cancer cells, magnetic and superparamagnetic NPs appear to be used as NCs for specific delivery of chemotherapy drugs such as TMX (Barar et al. Citation2015, Heidari Majd et al. Citation2013a, Hu et al. Citation2006). It is clear that unmodified magnetic nanoparticles (MNPs) have short plasma half-life and must be modified with biocompatible polymers such as polyethylene glycol (PEG) (Prabhakaran et al. Citation2006, Prijic and Sersa Citation2011). Surface modifying of MNPs with PEG could provide higher accumulation in the target sites, better blood circulation time, and lower elimination through reticuloendothelial system (RES) (Aronov et al. Citation2003, Ebrahimi et al. Citation2016, Heidari Majd et al. Citation2013a, Xie et al. Citation2007). Also, surface modification enables the MNPs to be more decorate with other functionalized group such as targeting agents and or hosting factors (Heidari Majd et al. Citation2013b, Hilgenbrink and Low Citation2005, Kang et al. Citation2009, Kohler et al. Citation2004).
In previous work, we engineered PEGylated MNPs armed with folic acid and loaded with TMX (Heidari Majd et al. Citation2013c), but in this study, we aimed to demonstrate the efficacy of CD-conjugated PEGylated MNPs for TMX delivery to the MCF-7 cancer cells and also to evaluate the slower release of TMX in simulated condition by use of host–guest system. Therefore, we expect the CD-conjugated MNPs can increase the circulation time of TMX which is useful for enhanced permeability and retention (EPR) effect and also can decrease the side effect of TMX such as hepatotoxicity.
Materials and methods
Sodium citrate, 1,1'-carbonyldiimidazole (CDI), triethylamine, and 3–(4, 5-dimethylthiazol-2-yl)-2, 5-diphenyltetrazolium bromide (MTT) were purchased from Merck (Germany). TMX, Triton X-100, and paraformaldehyde were obtained from Sigma–Aldrich (Germany). β-CD was purchased from Acros (Belgium). Sodium lauryl sulfate (SLS) was obtained from Parstous (Iran). MCF-7 cells were obtained from Pasture Institute (Iran). All media and cell culture components were obtained from Invitrogen (Germany). Terminal deoxynucleotidyl transferase-mediated dUTP nick-end labeling (TUNEL kit) was obtained from Roche (Switzerland).
Engineering and morphological characterization of PEGylated MNPs
MNPs were synthesized and modified with dopamine-polyethylene glycol-amine (DPA-PEG-NH2) to gain Fe3O4-DPA-PEG-NH2, as described previously (Heidari Majd et al. Citation2013a, Sargazi et al. Citation2015). The morphology and size of the engineered MNPs were characterized using transmission and scanning electron microscopies as reported previously (Barar et al. Citation2015, Saei et al. Citation2014).
Synthesis of Fe3O4-DPA-PEG-CD
β-CDs (0.16 g, 0.14 mmol) and 1,1'-CDI (0.034 g, 0.24 mmol) were dissolved in 3 mL N, N-dimethylformamide (DMF), and was mixed on a magnetic stirrer at room temperature (RT) for 1 h. Then 10 mL cold diethylether was added to reaction mixture and precipitated with centrifuge at 4000×g. The product was dissolved in 2 mL dimethylsulfoxide (DMSO) and stored at 4 °C. Separately, the Fe3O4-DPA-PEG-NH2 (0.40 g, containing 0.13 mmol of NH2 groups) was dissolved in 3 mL DMSO and also activated β-CD (β-CD-CI) in DMSO and triethylamine (0.12 mL) were added dropwise over 2 h and then stirring was continued for 5 h. The mixture was precipitated with diethylether and collected using the magnetic bead separation system “Dynamag TM-50.”
Characterization of Fe3O4-DPA-PEG-CD NPs
The size and morphological studies of β-CD-conjugated MNPs were performed with field emission scanning electron microscopy (FESEM) (Mira 3-XMU) and transmission electron microscopy (TEM) (LEO 906, Carl Zeiss, Germany). The surface modifications of MNPs were validated by FT-IR spectroscopy using Shimadzu IR PRESTIGE 21 spectrophotometer (Shimadzu Scientific Instruments, Tokyo, Japan). An energy-dispersive X-ray spectroscope (EDS) attached to the FESEM was used to analyze the sample composition of modified MNPs.
TMX loading
The Fe3O4-DPA-PEG-CD NPs (50 mg) were dissolved in 5.0 mL phosphate buffered saline (PBS, pH 7.4) and sonicated for complete dispersion. TMX (120.0 mg) was dissolved in 2.0 mL DMSO and sonicated for 10 min. These two solutions were mixed and sonicated for 15 min, and kept under shaking (150 rpm) at 25 °C overnight. Then, Fe3O4-DPA-PEG-CD-TMX NPs were collected using Dynamag TM-50 system. The supernatant was examined by UV (CECIL, CE1021) to determine the entrapment efficiency and the associated drug percentage (Memisoglu-Bilensoy et al. Citation2005).
Drug release evaluation
Release profiles of TMX from Fe3O4-DPA-PEG-CD-TMX NPs were determined in PBS pH 7.4 containing SLS (0.10% w/v) as surfactant to solvate the released TMX in PBS. Accordingly, 1.0 mg/mL of Fe3O4-DPA-PEG-CD-TMX NPs were sealed at 37 °C into a dialysis bag (MWCO = 14,000) (Sigma-Aldrich, St. Louis, MO) and placed in 40 mL of buffer solution. Incubation was continued for 120 h. Released TMX from PEGylated MNPs was quantified by UV spectroscopy at 278 nm. Also, for the control, release of free TMX from dialysis bag was done in 40 mL buffer and same conditions.
Cell culture and in vitro cytotoxicity analysis: MTT assay
MTT assay was used to determine the cytotoxicity of prepared MNPs in breast cancer MCF-7 cells (Anari et al. Citation2015, Sorinezami et al. Citation2014). Cells were routinely cultured at a seeding density of 4.0 × 104 cells/cm² in RPMI 1640 media supplemented with 10% fetal bovine serum, 100 units/mL penicillin G, and 100 mg/mL streptomycin at 37 °C in a humidified CO2 incubator during cultivation and during experiments. MCF-7 cells at a density of 1 × 104 cells/well (200 μL) were seeded in 96-well plates. After culturing for 24 h (40–50% confluency), the medium was exchanged with 200 μL medium containing different concentrations (i.e., ranging from 15 to 240 μM) of Fe3O4-DPA-PEG-CD-TMX NPs, Fe3O4-DPA-PEG-CD NPs, and free TMX. The concentration of Fe3O4-DPA-PEG-CD-TMX NPs was set to be equivalent to the TMX alone. After specified periods of time (i.e., 24, 48, and 72 h), the culture medium was removed from each well and then subjected to 3–(4, 5-dimethylthiazol-2-yl)-2, 5-diphenyltetrazolium bromide (MTT). About 150 mL of fresh media plus 50 mL MTT solutions (prepared as 2.0 mg/mL in FBS) were added to each well and incubated for 4 h at 37 °C in a CO2 incubator. The media were removed and the cells were washed (3×), and then the formazin crystals were solubilized with 200 μL DMSO and 25 μL Sorenson’s buffer for each well. The absorbance was read at 570 nm using a spectrophotometer (BioTek Instruments, Inc., Bad Friedrichshall, Germany).
Apoptotic study by TUNEL assay
MCF-7 cells were treated with 14 μM of prepared MNPs for 72 h. Cells were washed with PBS and then control and treated cells were fixed in 4.0% paraformaldehyde in PBS for 1 h and rinsed (3×) with PBS. A solution containing 0.1 g Triton X-100 and 0.10 g sodium citrate in 100 mL of molecular grade H2O was added for 15 min at RT to permeabilize the cells. After washing with PBS (3×), cells were incubated with TUNEL reaction mixture for 1 h at 37 °C in a humidified atmosphere in the dark. Cells were rinsed with PBS (3×) and photographed using fluorescent microscope.
In vitro analysis of hepatotoxicity
Rat hepatocytes were collected by the collagenase perfusion method (Shahraki et al. Citation2014) and cells (2.0 × 104 cells/well) were cultivated onto 24-well plates in a CO2 incubator. At 12 h post-seeding, the cultured cells were treated with 240 μM of Fe3O4-DPA-PEG-CD-TMX NPs, Fe3O4-DPA-PEG-CD NPs, and free TMX. The cells were incubated for 3 d and cell viability was assessed by MTT as described above. The absorbance was read at 570 nm using BioTek plate reader.
Results
Synthesis and characterization
schematically represents the all modification steps of MNPs. Fe3O4 MNPs (∼7–10 nm) were synthesized by the thermal decomposition reaction at 270 °C according to previously reported method (Sargazi et al. Citation2015). The surface of MNPs was modified by bifunctional PEG moiety containing an anchoring agent (DPA) at one end and amino group at the other end to form Fe3O4-DPA-PEG-NH2 NPs. To obtain β-CD functionalized MNPs, hydroxyl groups of β-CD were activated by 1.7 equivalent of 1,1'-CDI and then covalently reacted with amino group of Fe3O4-DPA-PEG-NH2 NPs (1.1:1) to form Fe3O4-DPA-PEG-CD as a host carrier.
The TEM and FESEM determined the size and morphology of MNPs. (panel A and B) represents the FESEM micrograph of Fe3O4-DPA-PEG-NH2 and Fe3O4-DPA-PEG-CD NPs which show average sizes of 12 and 31 nm, respectively.
Figure 2. Morphology, size and composition of the engineered MNPs. (A) SEM micrograph of PEGylated MNPs (MNP-DPA-PEG-NH2). (B) SEM micrograph of CD-conjugated PEGylated MNPs (MNP-DPA-PEG-CD). (C) TEM micrograph of CD-conjugated PEGylated MNPs. (D) EDS of CD-conjugated PEGylated MNPs.
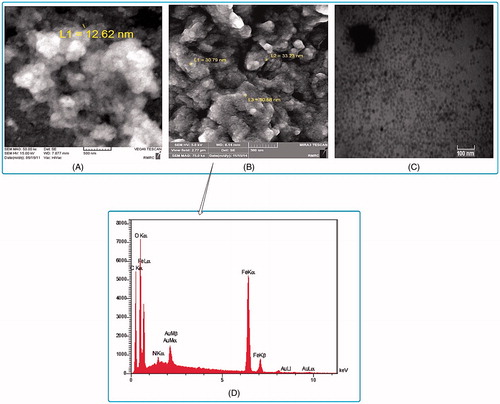
The FTIR spectroscopy confirmed the surface modification of MNPs with DPA-PEG-NH2 and then with CD. In the FTIR spectrum of PEGylated MNPs (, panel A), absorption peak at νmax 1487 cm–1 well identify existence of NH2-terminus. In addition, in both spectra, the absorption peaks related to Fe3O4 and DPA-PEG are obvious. The main absorption peaks for Fe3O4-DPA-PEG-CD NPs are: the α-pyranyl of β-CD (910 cm–1), C–O–C of glycosidic bridge (1205 cm–1), the coupled C–O/C–C (1025 and 1110 cm–1). Also, the multiple peaks in the range of 1600–1700 are related to carbonyl, amide, and ester groups of the modified MNPs (, panel B).
Figure 3. FT-IR Spectra of (A) Fe3O4–DPA-PEG-NH2 and (B) Fe3O4-DPA-PEG-CD; the multiple peaks at 1650–1750 cm–1 well proves the presence of amide bonds between PEG and CD.
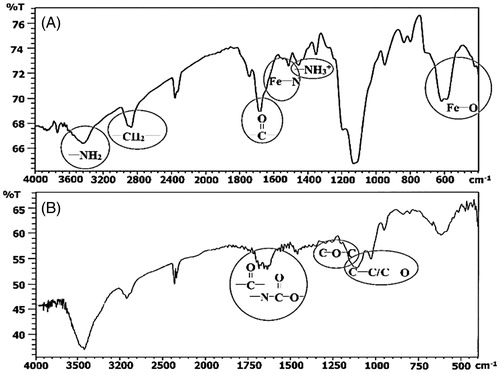
By the EDS attached to FESEM, the element composition of the samples was evaluated. The results in (panel D) indicate the existence of C, O, N, and Fe in the structure of MNPs. Atom Au in EDS pattern is for supporting FESEM specimens. Quantitative results of the composition of modified MNPs were shown that 10.38% of sample is Fe. So, after calculation using molecular weight was revealed that Fe3O4 constitute nearly 14.34% of the sample (Table S1 in electronic supplementary information (ESI)).
Loading and release evaluation
TMX molecules were loaded in the cavities of CDs as host sites of modified MNPs. Then, Fe3O4-DPA-PEG-CD-TMX NPs were collected using the magnetic bead separation system and the remaining amount of TMX was evaluated by UV/vis (λ = 278 nm) spectroscopy method. The amount of entrapped TMX in the cavities of CDs is 104.94 mg when 120 mg TMX was added to the MNPs solution. Hence, the entrapment efficiency calculates 33 mg TMX per unit CD (mg) and the associated drug percentage (loading efficiency) calculates 87.5%. In vitro drug release experiment was carried out for 120 h (). Nearly 41% of release occurred within the first 24 h. Also, the release profile revealed that 91% of TMX is released after 120 h. To demonstrate the superiority of this system, free TMX was sealed in dialysis bag and release time was evaluated in the same conditions. As expected, nearly 99% of TMX crossed from dialysis membrane after 7 h.
Cytotoxicity effects of TMX-loaded MNPs
The tumor inhibition activities of different CD-conjugated MNPs were evaluated through detection of cell viability assay (MTT) and also cell apoptosis assay (TUNEL). As represents (panel A–C), both of the Fe3O4-DPA-PEG-CD-TMX and free TMX significantly induce the cytotoxicity in the MCF-7 cells (i.e., ∼90–95% toxicity after 72 h), but after 72 h treatment for the CD-conjugated MNPs did not deduce any considerable cytotoxic effects. The IC50 values of Fe3O4-DPA-PEG-CD-TMX and free TMX were evaluated 14 and 10 μM, respectively. The inhibition influence of free TMX on MCF-7 cell lines seems to be concentration dependent, while influence of Fe3O4-DPA-PEG-CD-TMX NPs on breast cancer cells is time and concentration dependent inhibitory effects. To reveal the apoptosis findings, the fluorescein-dUTP was used for TUNEL assay. In the magnified section of (panel D), programmed cell death, or apoptosis is obvious by the DNA fragmentation in the treated cell lines versus the untreated cells. We found this significant induction of apoptosis (∼47%) in the MCF-7 cells when they treated with only 14 μM of Fe3O4-DPA-PEG-CD-TMX.
Figure 5. Cytotoxic (MTT assay), apoptosis (TUNEL assay) and hepatotoxicity study of Fe3O4-DPA-PEG-CD, Fe3O4-DPA-PEG-CD-TMX NPs and Pure TMX in the MCF-7 breast cancer cells. (A) Treated MCF-7 cells after 24 h. (B) Treated MCF-7 cells after 48 h. (C) Treated MCF-7 cells after 72 h. Data represents mean (n = 5) +SD. (D) DNA fragmentation of MCF-7 cells treatment with 14 μM Fe3O4-DPA-PEG-CD-TMX NPs for 72 h (TUNEL assays). (E) Effect of cyclodextrin on induced hepatotoxicity by TMX. Cyclodextrin can decrease the cell death induced by TMX nearly 30%.
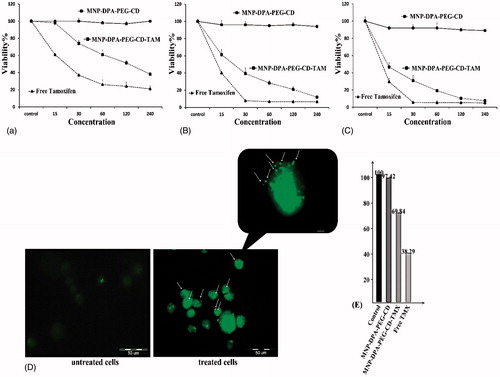
Evaluation of hepatotoxicity
With a simple method, the protective effects of the CD-conjugated MNPs against TMX-induced hepatotoxicity in rats were evaluated by MTT assay. Rat hepatocytes were collected and then were treated with maximum amount of Fe3O4-DPA-PEG-CD-TMX NPs, Fe3O4-DPA-PEG-CD NPs, and free TMX. (Panel E) represents the impact of different MNPs on hepatocytes. The CD-conjugated MNPs have reduced the cell death induced by TMX; so that, only 30% of exposed cells with Fe3O4-DPA-PEG-CD-TMX NPs were dead, but free TMX was the cause of 62% of the cell death in hepatocytes.
Discussion
The PEGylated MNPs are considered for biocompatibility and hydrophilic properties when they used as a drug carriers (Jahangiri and Akbarzadeh Citation2015). They display better pharmacokinetic properties with improved blood circulation time. Also, PEGylated MNPs can be decorated with functional groups such as CD, resulting in enhanced drug delivery. Hosting of CDs is an effective strategy for stabilizing the guest molecules against aggregation, thermal denaturation, or degradation (Sun et al. Citation2008, Teixeira et al. Citation2014). In recent decades, NP delivery systems based on CDs are considered as interesting agents to enhance drug solubility and loading. The CDs are capable to inclusion high amount of TMX in their hydrophobic cavities; therefore, relatively slower in vitro release can happen to enhance the efficacy of TMX in cancer therapy (Memisoglu-Bilensoy et al. Citation2005). Our previous report was about the synthesis of folic acid armed PEGylated MNPs in which TMX was loaded physically (Heidari Majd et al. Citation2013a). Because of physical loading of TMX onto this magnetic carrier, the loading efficiency was not only 49%, but also about 80% of release occurred within the first 24 h. For this reason in this study, β-CD was selected as a drug homing agent for engineering of multifunctional NC. For synthesis of such multimodal NC, CD molecules were covalently conjugated to the Fe3O4-DPA-PEG-NH2 NPs to perform Fe3O4-DPA-PEG-CD NPs that were then loaded with TMX molecules. FT-IR analyses confirmed all the modifications of MNPs (, panel A and B), so that the multiple peaks at 1650–1750 cm–1 well prove the presence of amide bonds between PEG and CD. Also, the EDS analysis revealed that nearly 85% of Fe3O4-DPA-PEG-CD NPs are made of C, O, and N; indicative the surface modification of MNPs with PEG and β-CD. Successful engineering of CD-grafted MNPs was confirmed by TEM and FESEM analysis when the average size became ∼31 nm. Similar to our work, CDs have been immobilized onto MNPs via 3-aminopropyltriethoxysilane with an average size of ∼196 nm (Cai et al. Citation2011). Also, in another same work, the β-CD functionalized MNPs were prepared with average sizes about 11 nm for detection of the DPA (Wu et al. Citation2009). It is noteworthy to mention that small NPs (<10 nm) can be quickly removed from the blood circulation through the clearance functions of kidney and/or liver, while the larger particles show tendency to be cleared by mononuclear phagocyte system, the so-called RES (Barar et al. Citation2015, Beach et al. Citation1992, Petros and DeSimone Citation2010). Hence, we speculate that the CD-conjugated PEGylated MNPs with a size range at ∼31 nm have freely distribution with no aggregations, lower kidney/hepatic filtration, and extremely penetration into the solid tumors. Because of TMX dose-dependent side effects, use of biocompatible targeted NCs for efficient delivery of TMX can provide maximal pharmacological effects with minimal inadvertent side effects (Yu and Bender Citation2002). On the other hand, TMX does not has sufficient water solubility to be readily delivered to the tumor cells (Boyd et al. Citation2015). Therefore, with the unique properties of CD we were able to enhance the sustained release of TMX for 120 h. Although, the circulation time is better to be evaluated by the in vivo tests, but long release time of Fe3O4-DPA-PEG-CD-TMX versus free TMX could confirm the superiority of this system in raising the blood circulation time. Also, the Fe3O4-DPA-PEG-CD-TMX NPs display a trend of time and concentration dependent inhibitory effects in MTT assay; unlike with our previously NCs which only showed concentration dependent inhibitory effects (Heidari Majd et al. Citation2013a). Accordingly, TUNEL apoptosis assay showed occurrence of apoptosis within the MCF-7 cells treated with 14 μM (equivalent with IC50) of Fe3O4-DPA-PEG-CD-TMX NPs.
TMX, as a nonsteroidal agent with potent antiestrogenic effects, can use in the treatment and prevention of hormone-dependent breast cancer. TMX can also induce the promoter activity of maspin which is silenced during breast cancer progression (Heidari Majd et al. Citation2013b, Khalkhali-Ellis et al. Citation2004). But, TMX therapy may be accompanied with undesired effects in normal cells such as hepatic injury (Albukhari et al. Citation2009). Forming the drug-CD complex by inclusion in cavities of CDs can exert a profound effect on the physicochemical and biological properties of the drugs such as: solubility, stability, taste, etc. (Del Valle Citation2004). It was confirmed that hepatoprotective effects of some compounds have been increased when they formulated with CD, because CD could improve the performance of compounds in terms of efficacy, solubility, and bioavailability (Hermenean et al. Citation2012). According to effect of CD on TMX sustained release, for the first time we decided to simply evaluate about the protective effect of CD on hepatotoxicity of TMX. For this purpose, effects of prepared MNPs on rat hepatocyte were evaluated by MTT assay. It was found that CD can decrease the cell death induced by TMX nearly 30%. Although we know that this assessment needs to be further researched.
Conclusion
Nowadays PEGylated MNPs have become to the potential drug carriers. However, most of these magnetic drug carriers have been loaded by exploiting of physical techniques; therefore their clinical applications due to rapid release of the drugs are limited. In this work, β-CD was selected as a drug homing agent for engineering of multifunctional magnetic NC. According to slow sustained release, the TMX loaded CD-conjugated MNPs not only can induce the apoptosis into the MCF-7 cells, but also can act as a protective for induced hepatotoxicity by TMX. Based upon our findings about protective effect of CD, we can propose these TMX-loaded PEGylated MNPs as multifunctional nanomedicine with simultaneous therapeutic and hepatoprotective effects.
Acknowledgements
The authors thank the Zabol University of Medical Sciences, for all support provided.
Disclosure statement
The authors report no declarations of interest. The authors alone are responsible for the content and writing of the paper.
References
- Albukhari AA, Gashlan HM, El-Beshbishy HA, Nagy AA, Abdel-Naim AB. 2009. Caffeic acid phenethyl ester protects against tamoxifen-induced hepatotoxicity in rats. Food Chem Toxicol. 47:1689–1695.
- Anari E, Akbarzadeh A, Zarghami N. 2015. Chrysin-loaded PLGA-PEG nanoparticles designed for enhanced effect on the breast cancer cell line. Artif Cells Nanomed Biotechnol. [Epub ahead of print]. doi: 10.3109/21691401.2015.1029633.
- Aronov O, Horowitz AT, Gabizon A, Gibson D. 2003. Folate-targeted PEG as a potential carrier for carboplatin analogs. Synthesis and in vitro studies. Bioconjug Chem. 14:563–574.
- Barar J, Kafil V, Majd MH, Barzegari A, Khani S, Johari-Ahar M, et al. 2015. Multifunctional mitoxantrone-conjugated magnetic nanosystem for targeted therapy of folate receptor-overexpressing malignant cells. J Nanobiotechnology. 13:1–16.
- Beach MC, Morley J, Spiryda L, Weinstock SB. 1992. Effects of liposome encapsulated hemoglobin on the reticuloendothelial system. Biomater Artif Cells Immobilization Biotechnol. 20:771–776.
- Boyd BJ, Galle A, Daglas M, Rosenfeld JV, Medcalf R. 2015. Traumatic brain injury opens blood–brain barrier to stealth liposomes via an enhanced permeability and retention (EPR)-like effect. J Drug Target. 23:847–853.
- Cai K, Li J, Luo Z, Hu Y, Hou Y, Ding X. 2011. β-Cyclodextrin conjugated magnetic nanoparticles for diazepam removal from blood. Chem Commun (Camb). 47:7719–7721.
- Del Valle EM. 2004. Cyclodextrins and their uses: a review. Process Biochem. 39:1033–1046.
- Ebrahimi E, Khandaghi AA, Valipour F, Babaie S, Asghari F, Motaali S, et al. 2016. In vitro study and characterization of doxorubicin-loaded magnetic nanoparticles modified with biodegradable copolymers. Artif Cells Nanomed Biotechnol. 44:550–558.
- Heidari Majd M, Asgari D, Barar J, Valizadeh H, Kafil V, Abadpour A, et al. 2013a. Tamoxifen loaded folic acid armed PEGylated magnetic nanoparticles for targeted imaging and therapy of cancer. Colloids Surf B Biointerfaces. 106:117–125.
- Heidari Majd M, Asgari D, Barar J, Valizadeh H, Kafil V, Coukos G, Omidi Y. 2013b. Specific targeting of cancer cells by multifunctional mitoxantrone-conjugated magnetic nanoparticles. J Drug Target. 21:328–340.
- Heidari Majd M, Barar J, Asgari D, Valizadeh H, Rashidi MR, Kafil V, Shahbazi J, Omidi Y. 2013c. Targeted fluoromagnetic nanoparticles for imaging of breast cancer MCF-7 cells. Adv Pharm Bull. 3:189–195
- Hermenean A, Popescu C, Ardelean A, Stan M, Hadaruga N, Mihali CV, Costache M, Dinischiotu A. 2012. Hepatoprotective effects of Berberis vulgaris L. extract/β cyclodextrin on carbon tetrachloride-induced acute toxicity in mice. Int J Mol Sci. 13:9014–9034.
- Hilgenbrink AR, Low PS. 2005. Folate receptor-mediated drug targeting: from therapeutics to diagnostics. J Pharm Sci. 94:2135–2146.
- Hu F, Neoh K, Kang E. 2006. Synthesis and in vitro anti-cancer evaluation of tamoxifen-loaded magnetite/PLLA composite nanoparticles. Biomaterials. 27:5725–5733.
- Jahangiri S, Akbarzadeh A. 2015. Preparation and in vitro evaluation of methotrexate-loaded magnetic nanoparticles modified with biocompatible copolymers. Artif Cells Nanomed Biotechnol. [Epub ahead of print]. doi: 10.3109/21691401.2015.1090443.
- Kang S, Choi I, Lee K-B, Kim Y. 2009. Bioconjugation of poly(poly(ethylene glycol) methacrylate)-coated iron oxide magnetic nanoparticles for magnetic capture of target proteins. Macromol Res. 17:259–264.
- Khalkhali-Ellis Z, Christian AL, Kirschmann DA, Edwards EM, Rezaie-Thompson M, Vasef MA, et al. 2004. Regulating the tumor suppressor gene maspin in breast cancer cells a potential mechanism for the anticancer properties of tamoxifen. Clin Cancer Res. 10:449–454.
- Kohler N, Fryxell GE, Zhang M. 2004. A bifunctional poly(ethylene glycol) silane immobilized on metallic oxide-based nanoparticles for conjugation with cell targeting agents. J Am Chem Soc. 126:7206–7211.
- Lockhart JN, Stevens DM, Beezer DB, Kravitz A, Harth E. 2015. Dual drug delivery of tamoxifen and quercetin: regulated metabolism for anticancer treatment with nanosponges. J Control Release. 220:751–757.
- Memisoglu-Bilensoy E, Vural I, Bochot A, Renoir JM, Duchene D, Hıncal AA. 2005. Tamoxifen citrate loaded amphiphilic beta-cyclodextrin nanoparticles: in vitro characterization and cytotoxicity. J Control Release. 104:489–496.
- Petros RA, DeSimone JM. 2010. Strategies in the design of nanoparticles for therapeutic applications. Nat Rev Drug Discov. 9:615–627.
- Prabhakaran M, Manjula BN, Acharya SA. 2006. Molecular modeling studies of surface decoration of hemoglobin by maleimide PEG. Artif Cells Blood Substit Immobil Biotechnol. 34:381–393.
- Prijic S, Sersa G. 2011. Magnetic nanoparticles as targeted delivery systems in oncology. Radiol Oncol. 45:1–16.
- Saei AA, Barzegari A, Majd MH, Asgari D, Omidi Y. 2014. Fe3O4 nanoparticles engineered for plasmid DNA delivery to Escherichia coli. J Nanopart Res. 16:1–11.
- Sargazi A, Kuhestani K, Nosrat Nahoki T, Heidari Majd M. 2015. Specific targeting of folate receptor by methotrexate conjugated modified magnetic nanoparticles: enzymatic release and cytotoxic study. IJPSR. 6:5047–5055.
- Shahraki J, Motallebi A, Barekati I, Seydi E, Pourahmad J. 2014. Comparison of cellular and molecular cytotoxic mechanisms of Cochlodinium polykrikoides in isolated trout and rat hepatocytes. Toxicol Environ Chem. 96:917–930.
- Sorinezami Z, Mansouri-Torshizi H, Shahraki S, Ghahghaei A, Doostkami M, Divsalar A, Sabouri AA, Majd MH. 2014. Synthesis, cytotoxicity and DNA binding of novel binuclear antitumor complexes formed by linking two “2,2'- bipyridine palladium (II)” moieties via alkylene bisdithiocarbamates. AJPTI. 2:105–122.
- Sun Y, Xu L, Xu P, Ma B. 2008. Preparation of levodopa-2, 6-dimethyl-beta-cyclodextrin inclusion by saturation solution process. Artif Cells Blood Substit Immobil Biotechnol. 36:352–359.
- Teixeira K, Denadai A, Sinisterra R, Cortés M. 2014. Cyclodextrin modulates the cytotoxic effects of chlorhexidine on microrganisms and cells in vitro. Drug Deliv. 22:444–453.
- Tonelli AE. 2014. Non-stoichiometric polymer-cyclodextrin inclusion compounds: constraints placed on un-included chain portions tethered at both ends and their relation to polymer brushes. Polymers. 6:2166–2185.
- Wu Y, Zuo F, Zheng Z, Ding X, Peng Y. 2009. A novel approach to molecular recognition surface of magnetic nanoparticles based on host-guest effect. Nanoscale Res Lett. 4:738–747.
- Xie J, Xu C, Kohler N, Hou Y, Sun S. 2007. Controlled PEGylation of monodisperse Fe3O4 nanoparticles for reduced non-specific uptake by macrophage cells. Adv. Mater. 19:3163–3166.
- Yu F-L, Bender W. 2002. A proposed mechanism of tamoxifen in breast cancer prevention. Cancer Detect Prev. 26:370–375.