Abstract
Purpose: The most important challenges in photodynamic therapy (PDT) are related to the limited penetration of light and the low uptake of photosensitizers. In radiotherapy, they are correlated to radiation damage of normal tissues. Therefore, a targeted radio and photosensitizer can reduce the limitations of the mentioned methods. In this study, photosensitizing and radio-sensitizing effects of 5-aminolevulinic acid (5ALA)-conjugated GNPs were investigated. Materials and methods: First, cell toxicities of 5ALA, GNPs and a conjugate were assessed on Mel-Rm cell line. Then, the radio sensitizing effect of every agent was studied. Different experiments were designed in four separate groups, each group containing six subgroups receiving different radiation doses by using a superficial X-ray tube. Furthermore, the photosensitizing efficacy of the agents was evaluated after cells were irradiated by a He–Ne laser at four light doses in separate groups. Results: With regards to radio sensitivity assessments, there was no significant difference between different irradiation doses. The investigation on photosensitivity of 5ALA and a conjugate showed significant differences between the control (without illumination) and groups that received PDT in the presence of 5ALA and conjugate, wherein ED50 were estimated at 136.2 J/cm2 and 56.2 J/cm2, respectively. With regards to PDT experiments, the conjugate induces cell death more than twice in comparison with 5ALA. Conclusion: The conjugate does not cause any enhancement of radiation efficiency on MeL-Rm cell line. With regards to PDT, we found that the conjugate induced cell death at twice the rate when compared with 5ALA alone. Therefore, the conjugate can be an appropriate delivery agent for 5ALA and may also enhance the destruction of tumor cells. Finally, comparing the two types of treatment shows that PDT is a more efficient treatment for this cell line.
Introduction
Radiotherapy and photodynamic therapy (PDT), as two non-invasive techniques in cancer treatment, have been preferred to surgical resection, because, they help to preserve the structure and function of the involved organs (Takahashi et al. Citation2013). Photodynamic therapy (PDT) utilizes an activated photosensitizer by irradiating an appropriate wavelength (generally in the transparent optical window region between 600–800 nm). Preferential localization of the photosensitizer into the malignant tissue, minimal side effects, and inducing apoptosis are the most important advantages of PDT (Cabuy Citation2012). However, PDT suffers from important challenges that can be referred to two problems; the first is the lack of a complete selectivity and localization of the photosensitizer in tumors vs. normal tissue, and the second one can be referred to the fact that most wavelengths of light cannot penetrate more than several mm through the tissue, thus the application of PDT will be limited to the treatment of superficial tumors and accessible malignancies. The first problem can be modified by using nanoparticles for providing good selectivity and localization of the photosensitizer in tumors. Nowadays, gold nanoparticles have been used as a good delivery agent in photodynamic therapy (Luksiene et al. Citation2006). Nanoparticles (NPs) act as a delivery agent of PSs “that could be protected from being up taken by the reticuloendothelial system and extend the circulation time of NPs in the blood, and finally, become preferentially accumulated in tumor sites through the so-called “enhanced permeability and retention (EPR)” effect” (Lkhagvadulam et al. Citation2013). Protoporphyrin IX (PpIX) is produced in cells via the heme synthesis pathway, from the substrate aminolevulinic acid (5ALA), and is known as a high bio-compatible photosensitizer (Kim et al. Citation2011, Sultan et al. Citation2006). Previous studies have shown that gold nanoparticles can act as the carrier for 5ALA and in the presence of the 5ALA-conjugated gold nanoparticles will result in increased efficiency of PDT (Hainfeld et al. Citation2004, Xu et al. Citation2012). Therefore, the conjugate can be considered as an effective photosensitizer that approximately covers the first problem of PDT. However, the second challenge that remains has been caused by the poor application of PDT in cancer treatment. On the other hand, during radiotherapy, not only tumors, but also normal tissues are damaged by high-energy radiation along its path. Although recent advancements in beam control technologies have enabled X-ray focusing on tumours (Rahman et al. Citation2009), the exposure tolerance of normal tissues along the radiation path remains a major concern. Gold nanoparticles with a high atomic number (z = 79) are known as a radiosensitizers and can enhance the deposited energy into the cells through the X-rays in the energy range of kilo voltage. Therefore, using gold nanoparticles causes the preservation of normal tissue and radiation dose enhancement in the target tumour (Yamamoto et al. Citation2012).
Previous studies have demonstrated that PpIX can cause enhanced generation of reactive oxygen species under X-ray irradiation (Takahashi et al. Citation2013). As a result of the biocompatibility of 5ALA and the activation capability of PpIX by X-ray, it is a suggested candidate to be activated as a sensitizer with X-ray irradiation (Thomas and Hersey Citation1998). A basic mechanism of X-ray absorption and ROS generation by PpIX refers to energy transfer into fluorescence and phosphorescence emission (Takahashi et al. Citation2013). Taking into account the subjects expressed, in this study, we evaluated the efficiency of photodynamic treatment and radiotherapy on melanoma cell lines by using 5ALA-conjugated gold nanoparticles as a photo and radiosensitizer and compared them with each other.
Material and methods
Chemicals
HAuCl4, Trypan blue and 5-ALA (∼95% purity) were purchased from the Sigma Company (St. Louis, MO). Fetal calf serum [FCS] and RPMI-1640 cell-culture medium was provided by the Gibco Company (USA, Lot No: 371748). For separating the cells from the bottom of the flasks used, Trypsin-EDTA was purchased from the Biogen Company (Iranian) and MTT Powder was purchased from the Merck Company (German).
Cell line and cell culture conditions
This study was performed on the Mel-Rm cell line. This cell line was separated from patients who had suffered from melanoma in the melanoma division of Newcastle and Sydney in Australia (Wieder et al. Citation2006). The cells were grown in 75-cm2 plastic tissue culture flasks as a monolayer on RPMI-1640 medium supplemented with 10% FBS and 1% antibiotics (penicillin 50 units/mL-streptomycin 50 mg/mL). The cells were incubated at 37 °C in a humidified atmosphere in a CO2 incubator containing 5% CO2. When the cells were proliferated and the flask was filled, they were detached from the flask bed using trypsin-EDTA. The Trypan blue method was used for cell counting and assurance of cell survival of more than 98% before performing designed treatments.
Conjugating 5ALA to GNPs and characterization of GNPs
GNPs were synthesized as described below (Mohammadi et al. Citation2013). Firstly, HAuCl4 was dissolved in water with a concentration of 0. 01 molar; ionic power and pH were set as 0.005M and 8.7, respectively, by a phosphate buffer system. A non-aqueous phase (Toluene C6H5CH3) containing NaBH4 (Sigma-Aldrich, Lot no. 247677, UK) in 0.02 M concentration was also prepared separately. Following this, both phases were added together and shaken strongly. Then, by separating the organic material phase, the solvent was removed by a rotary device at 500°C and under low-pressure conditions. In the last step, the GNPs that were gathered from the flask floor were dispersed in a phosphate buffer and eventually a homogeneous solution was obtained. This colloidal solution was characterized by a red color and transparent medium (Hainfeld et al. Citation2004).
The GNPs size distribution was determined using a particle size analyzer (Malvern Instruments, Southborough, MA). The GNPs UV-visible absorption spectrum was also recorded by a UV-visible spectrophotometer (Shimadzu Model UV1700; Japan).
For conjugating 5ALA to GNPs (conjugate), 5 mL of HAuCl4 (Sigma-Fluka, USA) aqueous solution (10 mM) and 5 ml of the polyethylene solution (12 mg/ml) were shed in the quartz container and placed in the ice bath. The gold was exposed to a UV light for five hours (245 nm and 300 w). Subsequently, the gold was obtained with a positive charge and a size of roughly 30 nm. Then, 5ALA was prepared in sterile water with a concentration of 20 mM. 10 ml of the prepared 5ALA was added to a solution that contained gold nanoparticles. 5ALA was attached to the surface GNPs by an electrostatic force. The PH of the resulting solution reached to 7.4 by NaOH and HCl. The solution was then sterilized by a 0.22 micron filter (Oo et al. Citation2008).
Cytotoxicity of 5ALA, GNPs and the conjugate
In this section, optimum 5-ALA concentration for the Mel-Rm cell line with no toxic effects was determined. The cells were not treated during this study. In this step, 104 of the Mel-Rm cell line were seeded in every well of the 96-well plate. Cells were then re-incubated for 24 h in a RPMI-1640 cell culture medium with 10% FBS and 1% penicillin/streptomycin in a humidified incubator at 37 °C with 5% CO2. Afterwards, the cells were washed with phosphate buffer saline solution (PBS) and different concentrations of 5-aminolevulinic acid (5ALA) solution were added to the cells and incubated for four hours (Hainfeld et al. Citation2004). The cell viability was evaluated by the MTT assay, which was recorded by an ELISA Reader (AWERNESS Company, FAX 2100, USA) at 545 nm in the 96-well plates.
Cytotoxicity of 5ALA was assessed in 0.25, 0.5, 1, 2 and 4 mM concentrations. The darkness toxicity of GNPs was evaluated in 0.05, 0.1, 0.2 and 0.4 mM of concentration. In the last step, the conjugate cytotoxicity was determined in 0.25, 0.5, 1 and 2 mM of concentration. All examination groups were repeated three times.
Cell uptake of 5ALA
As the accumulation of PpIX into the cells can present the rate of 5ALA uptake, we assessed the PpIX concentration after 4 h cell incubation with 5ALA and conjugate, separately (Mohammadi et al. Citation2013). The cell density was 3 × 104 cell/ml and 5ALA concentration was 1 mM. During cell incubation with the drugs, the cell-culture medium was supplemented with 2% FCS. Then PpIX was extracted and PpIX fluorescence signal intensity of the conjugate and 5-ALA were measured by the fluorometric method via a spectrophotometer (JASCO, FP-6200, Japan) with high sensitivity; the excitation and emission wavelengths were determined at 407 nm and 602 nm, respectively.
Experimental groups
After determination of the optimum concentration of the drugs, treatment groups were executed via two protocols: X-ray and photodynamic treatment.
The first protocol, to assess the X-ray treatment efficacy, the Mel-Rm cells were examined in different groups () as follows:
Table 1. Treatment conditions of the studied groups.
Control (with no additive agent and radiation).
To assess the effect of radiation alone on cell growth in different radiation doses.
To evaluate the effect of radiation in the presence of 5ALA and different radiation doses.
To assess the effect of radiation in the presence of GNPs on cell growth in different radiation doses.
To evaluate the effect of radiation in the presence of the conjugate and different radiation doses.
The conjugate concentration was expressed based on the existing 5ALA concentration in the conjugate suspension.
In the second protocol, to evaluate the photodynamic treatment efficacy, the Mel-Rm cells were examined in different groups () as shown below:
Control (in the absence of drug and light).
To assess the effect of light alone on cell growth in different light doses.
To evaluate the effect of light in the presence of 5ALA and different optical doses.
To assess the effect of light in the presence of GNPs on cell growth in different optical doses.
To evaluate the effect of light in the presence of the conjugate and different optical doses.
All the performed experiments were repeated three times.
In the beginning, trypsinization and cell counting were performed. After assurance of a cell survival rate of at least 98%, the cell suspension was prepared in a culture medium at a concentration of 5 × 104 cells/ml and was seeded in a 96-well plate for 24 h as described earlier. Then the drugs were incubated at the optimal concentration, which was obtained in the earlier stages. After removing additional drugs and washing the cells by PBS, the samples were refreshed with 100 μl cell-culture medium supplemented with 5% FBS to grow the cells. Then, the cells in a 96-well plate were treated with doses of 2, 4, 6, 8, 10, 12, 16 Gy X-ray at 1229.5 Gy/minute dose rate in a (7 × 7) cm2 field size by a superficial X-ray tube(Philips company) with 100 kVp. After irradiating in order to prepare a suitable condition for cell proliferation, 100 μl of the cell-culture medium was supplemented with 10% FBS, which was added to every well and incubated for 24 h. During the irradiation time, all of the samples were placed on ice.
Photodynamic therapy
After cell culture in a 96-well plate as described in the previous section, the drugs were incubated at an optimal concentration for four hours (Hainfeld et al. Citation2004). After removing the residual agents from the cell culture medium through cell-washing by PBS, the drugs were renewed with 100 μl cell-culture medium supplemented with 5% FBS to grow the cells. To determine the phototoxic effect, the cells were irradiated into 20, 40, 60 and 80 J/cm2 optical doses by a He–Ne laser (Product of Iran's Atomic Energy Research Center, 628 nm, 220V, 50 Hz). After treatment, 100 μl of the cell-culture medium supplemented with 10% FBS was added to every well and incubated for 24 h. In the final step, the cell viability was assessed by the MTT method. Note that during the irradiation time, all of the samples were also placed on ice.
Cell viability assessment
The viability of treated cells was evaluated by the MTT (3-[4, 5-dimethylthiazol-2-yl]-2, 5 diphenyltetrazolium bromide) assay. After unloading the wells from the cell-culture medium, 100 μl of cell-culture medium-free Phenol Red and 10 μl of MTT (0.5 mg/Ml) were added to each well. To avoid a reduction of MTT by background light, the plates were then wrapped in an aluminum foil. The plates were kept in an incubator for four hours (which is the time required for maximum MTT reduction). Then, the wells were refreshed by 200 μl dimethyl sulfoxide (DMSO). Absorbance of the extract was measured at 545 nm using a 96-well ELISA Reader (Awareness, Model STATFAX 2100; USA). All the performed experiments were repeated three times.
Judgment parameters
Sensitivity factor
For a closer look at the impact of each agent on X-ray treatment, a factor called sensitivity factor was defined, which shows the ratio of the average cell death in the presence of the drugs at different doses of X-rays to average cell death in the control group at the same doses. After the statistical tests on the relevant groups, the response of this cell line to the X-ray treatment in the presence of each drug can be examined.
Effective dose 50 (ED50)
In addition to the cell survival percentage after applying different designed treatments, ED50 (the required dosage for inducing 50% cell death) was utilized to estimate and compare the efficacy of the photodynamic treatment in the presence of 5ALA, GNPs, and their conjugate. This was calculated by the function that has been built into the Masterplex software (Four Parameter Logistics, published by the MiraiBio Group of Hitachi Solutions America, Ltd.) on the data.
Data analysis
The Kruskal–Wallis one-sample method was applied in order to guarantee the normal distribution of data and then statistical analysis was performed by one-way ANOVA and Tukey tests with the Statistical Package for Social Sciences (SPSS, Chicago, IL) software, version 16.
Results
Size distribution of GNPs
GNPs have positive zeta potentials. shows the size distribution curve; according to the curve, the maximum number of GNPs was seen at a diameter of about 34 nm.
Cytotoxicity of 5ALA, GNPs and the conjugate
According to , in the presence of 5ALA, as well as with an increasing concentration of 5ALA, the percentage of the Mel-Rm cell line survival was enhanced. This was observed as a significant difference between the control group and the group receiving 4 mM of 5ALA. In toxicity studies on the GNPs, it was observed that the cells viability was reduced by up to 40% for an AuNPs concentration of 0.4 mM (). In studying the toxicity of the conjugate, the cell survival percentage in the control group showed a significant difference compared to the other concentrations. According to , the cell survival percentage at the concentration of 2 mM showed a significant difference compared to that of concentrations of 0.25 and 0.5 mM.
Figure 2. Percentage of the cell survival in the presence of various concentrations of 5-ALA. Cell incubation period was selected for 4 h. The data represent mean ± standard error on the mean obtained from three performed experiments.
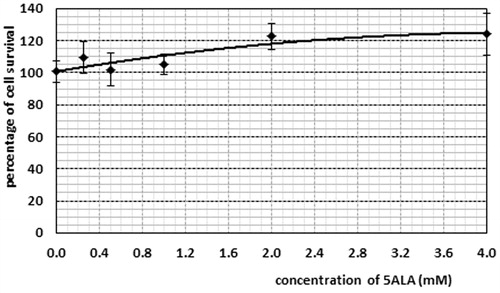
Fluorescence signal of PpIX
Recorded fluorescence signal of protoporphyrin IX after a 4 h incubation period of Mel-Rm cells with 5-ALA and conjugate were mean ± standard error, respectively. The fluorescence signal of the control group was subtracted from the other groups. There was a significant difference between the obtained signals of the groups receiving 5-ALA and the conjugate (P = 0.023).
X-ray treatment
X-ray treatment was performed on certain concentrations of each drug that were achieved in the cytotoxicity section. After incubation of the drugs on the Mel-Rm cell line, X-ray treatments were performed at 2, 4, 6, 8, 10, 12 and 16 Gy radiation doses on the four groups independently.
In order to eliminate the agents’ cytotoxicity from the recorded data, cell survival calculations of X-ray treatments were performed in the presence of each agent in relation to the dark as the control group. According to , in the absence of drugs, the control group showed a significant difference with groups that received radiation doses of 8, 10, 12 and 16 Gy.
Figure 5. Fluorescence signal of protoporphyrin IX after four hours incubation time of Mel-Rm cells with 5-ALA and conjugate. The cell density was 3 × 104 cell/ml and 5ALA concentration was 1 Mm. The control fluorescence signal was subtracted. The data represent mean ± standard error on the mean obtained from three performed experiments.
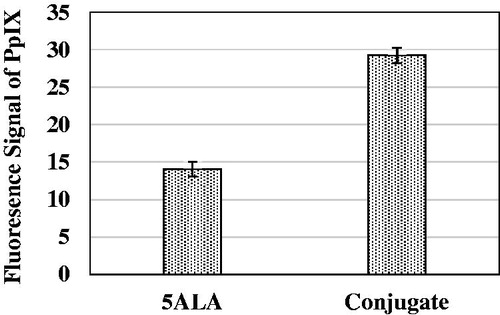
In the second group (in the presence of 5ALA alone), the results showed a significant difference between the control group and 4, 6, 8, 10, 12 and 16 Gy (). According to , significant differences were observed between the zero doses of GNPs and the groups receiving radiation doses of 2 Gy in the presence of GNPs. In the last group (4) of X-ray treatment, 5ALA conjugated gold nanoparticles were evaluated and a significant difference between the control group and 4 and 6 Gy dose groups was observed (). The sensitivity factor was calculated 1.33, 0.69 and 0.76 for 5ALA, GNPs and conjugate, respectively. No significant differences were observed between control group and the factors for each drug in the statistical tests between the groups.
Figure 6. Percentage of cell survival in the presence of 5ALA, GNPs and conjugate with different x-ray doses, 24 h after treatment. The incubation time of GNPs was 4 h. The data are expressed as mean 3 experiments ± standard error of mean.
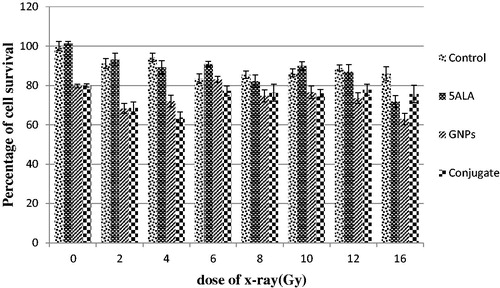
Figure 7. Percentage of cell survival of Mel-Rm cell line. 24 h after treatment. The incubation time of GNPs was 4 h. The data are expressed as mean 3 experiments ± standard error of mean.
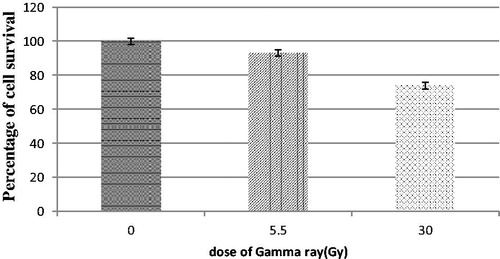
In this study, radiation sensitivity of Mel-Rm cell line was evaluated by using beams of gamma rays at doses of 5.5 and 30 Gy. The results showed a significant difference between the control group and 30 Gy group ().
Photodynamic therapy
Photodynamic therapy was executed with 20, 40, 60 and 80 J/cm2 optical doses on the four groups independently. As shown in , the cell survival percentage in group one decreased and then increased when the irradiation dose was increased to around 120%. In the presence of 5ALA, the maximum cell death was obtained with 60 J/cm2 of irradiation (P < 0.001) and cell survival increased with no significant difference between the optical doses of 60 and 80 J/cm2. In the presence of GNPs, significant differences were observed between the zero dose of GNPs and the groups receiving optical doses of 20 and 40 J/cm2 (P < 0.01). PDT efficacy was assessed in the presence of the conjugate in group four. As shown in , a noticeable dose-dependent reduction was observed 60 J/cm2 in cell survival that shows significant differences between the control group and the groups receiving 20, 40 and 60 J/cm2 of optical doses. The most effective dose for The PDT was 60 J/cm2 (). ED50 was calculated as 136.2 J/cm2 for 5ALA and 56.2 J/cm2 in the presence of the conjugate.
Figure 8. Cell survival percentage after different light doses irradiation, in the absence of drugs, in the presence of 5ALA, in the presence of GNPs and in the presence of conjugate 24 h after treatment. The data are expressed as mean of three repeated experiments ± standard error of mean.
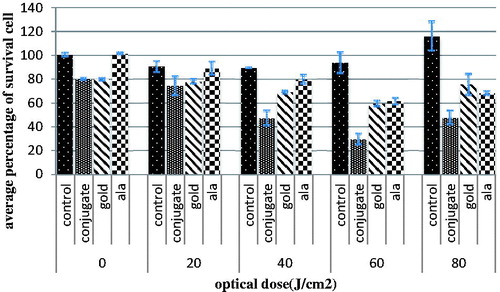
Figure.9. Cell survival percentage after different light doses irradiation, in the absence of drugs, in the presence of 5ALA, in the presence of GNPs and in the presence of conjugate 24 h after treatment at dose of 60 J/cm2. The data are expressed as mean of three repeated experiments ± standard error of mean.
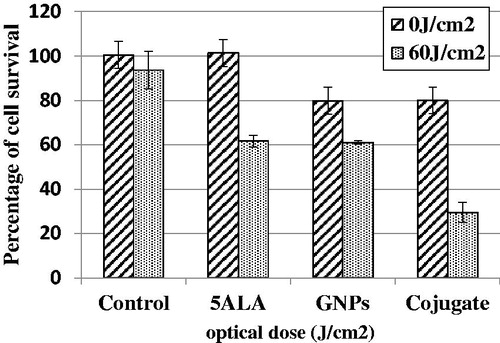
Discussion
Taking into account the drawbacks of PDT that are associated with inefficiency in the management of large or metastatic tumors due to low penetration through tissues and pain during treatment (Wachowska et al. Citation2011), this study used 5ALA-conjugated gold nanoparticles as a radiosensitizer in X-ray treatment on the Mel-Rm cell line and compared this method with photodynamic therapy in which gold nanoparticles were used as a delivery agent for 5ALA on the Mel-Rm cell line also. The size of the gold nanoparticles utilized in this project was 34 nm (Popov et al. Citation2007). Positive GNPs were bonded to negative 5ALA by an electrostatic bond and were applied as a conjugate. Then cell toxicity of the conjugates was evaluated. Afterwards, an assessment was conducted of the conjugate efficacy during X-ray treatment and PDT was performed at different doses on the Mel-Rm cell line. Following this, the sensitivity factor and ED50 were calculated to evaluate X-ray and photodynamic treatment efficiency. According to our findings, GNPs were found to be toxic on the Mel-Rm cell line in the applied concentrations. GNPs at the concentration of 0.2 mM, which was used in this study, exhibited 20% toxicity. All the studies that examined the cytotoxicity of GNPs can be classified into two groups; some of them in which the GNPs can enter cells efficiently are indicated to be nearly harmless to cultured cells (0) (Becker et al. Citation2004, Butterworth et al. Citation2010, Connor et al. Citation2005, Hauck et al. Citation2008, Paciotti et al. Citation2004, Yamamoto et al. Citation2012). However, some of them carried out in this field implied a lack of toxicity of GNPs (Alkilany and Murphy Citation2010, Oo et al. Citation2008). Different levels of gold nanoparticle toxicity can cause their size, cell type, anionic or cationic nature and their localization in the cells (cytoplasm or mitochondria), whereas cationic GNPs are more toxic than the anionic ones (0) (Goodman et al. Citation2004, Yah Citation2013).
The conjugate showed toxicity levels similar to GNPs because of the nontoxic nature of 5ALA. The effectiveness of nanoparticles refers to the level of their uptake by cells that was dependent on incubation time, particle size and concentration of nanoparticles (Mironava et al. Citation2014). GNPs have positive zeta potentials and show a great affinity for binding to negative cell membranes. The presence of the AuNP scan causes increased population doubling times, decreased cell motility and cell-mediated collagen contraction (Mironava et al. Citation2010).
In X-ray treatments, No significant differences were observed between control and the sensitivity factor for each particle. So, any enhancement effective treatment was observed in the presence of a conjugate. Failure to respond to this part of the treatment can be caused by various factors, such as incubation time after cells irradiation by X-ray, the size of the GNPs, X-ray dosage or resistance of the cell type used in this study. One parameter that was examined referred to the radiosensitivity of the Mel-Rm cell line. The radiation sensitivity of the cell types of the Mel-Rm derived from melanoma were evaluated. The results show that significant differences were observed in the 30 Gy of treatment group compared with the control group (). Therefore, the lack of response to treatment with X-ray scan can be caused by two factors: the radiation resistance of the Mel-Rm cell line, insufficient X-ray doses for this cell line or insufficient incubation time after cells irradiation for the proliferation effect of deleted cells from the cell cycle to appear.
Our findings in PDT on the Mel-Rm cell line showed, ED50 was reduced from 136.2 J/cm2 for 5ALA to 56.2 J/cm2 in the presence of the conjugate. Xu et al. produced another study that has also shown the increasing efficiency of PDT (Xu et al. Citation2012). This indicates that the presence of the conjugate leads to a more than two-fold increase in the efficiency of PDT on Mel-Rm cells in comparison to 5ALA alone. Based on previous experiments, efficiency can be enhanced by using a conjugate for PDT by two phenomena. Firstly, the conjugate can play the role as delivery agent for 5ALA and induce more 5ALA transport into the cells. Consequently, more PpIX is formed, thereby enhancing the effect of ALA-PDT because the hydrophilic nature of ALA limits its ability to penetrate cell membranes. Secondly, the presence of GNPs in the conjugate structure by itself does not increase PpIX formation; however, it helps the formation of single oxygen (1O2) indirectly. The reason for this is that the surface plasmon resonance (SPR) property of GNPs can increase the optical flow between the GNPs and PpIX after exposure (14).
Conclusion
In summary, we can compare the two photo and radio sensitivities of the conjugate on cell the Mel-Rm cell line. It seems this conjugate increases the photosensitivity of 5ALA more than its radiosensitivity and can act as an effective photosensitizer during PDT on this cell line. Further studies are necessary to identify the causes behind this finding.
Acknowledgements
The results presented in this work have been taken from MSC thesis relating the Medical Physics Department of Mashhad University of Medical Science. Financially, this work was supported by the Research Deputy of Mashhad University of Medical Science.
Disclosure statement
The authors report no conflicts of interest. The authors alone are responsible for the content and writing of this article.
References
- Alkilany AM, Murphy CJ. 2010. Toxicity and cellular uptake of gold nanoparticles: what we have learned so far? J Nanopart Res. 12:2313–2333.
- Becker ML, Bailey LO, Wooley KL. 2004. Peptide‐derivatized shell‐cross‐linked nanoparticles. 2. Biocompatibility evaluation. Bioconjug Chem. 1:710–717.
- Butterworth KT, Coulter JA, Jain S, Forker J, McMahon SJ, Schettino G, et al. 2010. Evaluation of cytotoxicity and radiation enhancement using 1.9 nm gold particles: potential application for cancer therapy. Nanotechnology. 21:1–18.
- Cabuy E. 2012. Reliable cancer therapies. Energy-based Therap. 3:1–54.
- Connor EE, Mwamuka J, Gole A, Murphy CJ, Wyatt MD. 2005. Gold nanoparticles are taken up by human cells but do not cause acute cytotoxicity. Small. 1:325–327.
- Goodman CM, McCusker CD, Yilmaz T, Rotello VM. 2004. Toxicity of gold nanoparticles functionalized with cationic and anionic side chains. Bioconjug Chem. 15:897–900.
- Hainfeld JF, Slatkin DN, Smilowitz HM. 2004. The use of gold nanoparticles to enhance radiotherapy in mice. Phys Med Biol. 49:N309–N315.
- Hauck TS, Ghazani AA, Chan WC. 2008. Assessing the effect of surface chemistry on gold nanorod uptake, toxicity, and gene expression in mammalian cells. Small. 4:153–159.
- Kim CH, Chung CW, Choi KH, Yoo JJ, Kim do H, Jeong YI, Kang DH. 2011. Effect of 5-aminolevulinic acid-based photodynamic therapy via reactive oxygen species in human cholangiocarcinoma cells. Int J Nanomedicine. 6:1357–1363.
- Lkhagvadulam B, Kim JH, Yoon I, Shim YK. 2013. Size-dependent photodynamic activity of gold nanoparticles conjugate of water soluble purpurin-18-N-methyl-d-glucamine. Biomed Res Int. 2013:720579.
- Luksiene Z, Juzenas P, Moan J. 2006. Radiosensitization of tumours by porphyrins. Cancer Lett. 235:40–47.
- Mironava T, Hadjiargyrou M, Simon M, Rafailovich MH. 2014. Gold nanoparticles cellular toxicity and recovery: adipose derived stromal cells. Nanotoxicology. 8:189–201.
- Mironava T, Hadjiargyrou M, Simon M, Jurukovski V, Rafailovich MH. 2010. Gold nanoparticles cellular toxicity and recovery: effect of size, concentration and exposure time. Nanotoxicology. 4:120–137.
- Mohammadi Z, Sazgarnia A, Rajabi O, Soudmand S, Esmaily H, Sadeghi HR. 2013. An in vitro study on the photosensitivity of 5-aminolevulinic acid conjugated gold nanoparticles. Photodiagnosis Photodyn Ther. 10:382–388.
- Oo MK, Yang X, Du H, Wang H. 2008. 5-Aminolevulinic acid-conjugated gold nanoparticles for photodynamic therapy of cancer. Nanomedicine. 3:777–786.
- Paciotti GF, Myer L, Weinreich D, Goia D, Pavel N, McLaughlin RE, Tamarkin L. 2004. Colloidal gold: a novel nanoparticle vector for tumor directed drug delivery. Drug Deliv. 11:169–183.
- Popov AP, Priezzhe AV, Myllylä1 R. 2007. Optimal sizes of gold nanoparticles for laser treatment of tumors. Fifth International Conference on Photonics and Imaging in Biology and Medicine. Proceedings of SPIE; Wuhan, China.
- Rahman WN, Bishara N, Ackerly T, He CF, Jackson P, Wong C, Davidson R, Geso M. 2009. Enhancement of radiation effects by gold nanoparticles for superficial radiation therapy. Nanomedicine. 5:136–142.
- Sultan SM, El-Doray AA, Hofstetter A, Abdel-Gawad O, El-MahdyAel D, Khoder W. 2006. Photodynamic selectivity of 5-aminolevulinic acid to prostate cancer cells. J Egypt Natl Canc Inst. 18:382–386.
- Takahashi J, Misawa M, Murakami M, Mori T, Nomura K, Iwahashi H. 2013. 5-Aminolevulinic acid enhances cancer radiotherapy in a mouse tumor model. Springerplus. 2:602.
- Thomas WD, Hersey P. 1998. TNF-related apoptosis-inducing ligand(TRAIL) induces apoptosis in Fas ligand-resistant melanomacells and mediates CD4T cell killing of target cells. J Immunol. 161:2195–2200.
- Wachowska M, Muchowicz A, Firczuk M, Gabrysiak M, Winiarska M, Wańczyk M, Bojarczuk K, Golab J. 2011. Aminolevulinic Acid (ALA) as a prodrug in photodynamic therapy of cancer. Molecules. 16:4140–4164.
- Wieder ME, Hone DC, Cook MJ, Handsley MM, Gavrilovic J, Russell DA. 2006. Intracellular photodynamic therapy with photo sensitizer—nanoparticles conjugates: cancer therapy using a Trojan horse. Photochem Photobiol Sci. 5:727–734.
- Xu H, Liu C, Mei J, Yao C, Wang S, Wang J, Li Z, Zhang Z. 2012. Effects of light irradiation upon photodynamic therapy based on 5-aminolevulinic acid-gold nanoparticle conjugates in K562 cells via singlet oxygen generation. Int J Nanomedicine. 7:5029–5038.
- Yah CS. 2013. The toxicity of gold nanoparticles in relation to their physiochemical properties. Biomed Res. 24:400–413.
- Yamamoto J, Ogura S, Tanaka T, Kitagawa T, Nakano Y, Saito T, et al. 2012. Radiosensitizing effect of 5-aminolevulinic acid-induced protoporphyrin IX in glioma cells in vitro. Oncol Rep. 27:1748–1752.