Abstract
Objective: The goal of this study was to characterize the light curing characteristics of a new oligomer PEM-665 designed to be used as an alternative monomer to BisGMA.
Materials and methods: PEM-665 (P) and BisGMA (B) solutions were prepared with triethylene glycol dimethacrylate (T) diluent in different weight proportions (70/30 and 50/50). Solutions containing 70% P and 30% T were designated as 70PT, 70%B and 30%T as 70BT, 50%P and 50%T as 50PT and 50%B and 50%T as 50BT. The initiators were CQ (EDMAB was used as amine accelerator for CQ) and DPO in 1% concentration. Eight solutions were prepared in a factorial design: 70PT/DPO; 70PT/CQ; 50PT/DPO; 50PT/CQ; 70BT/DPO; 70BT/CQ; 50BT/DPO; 50BT/CQ. BISCO VIP visible light was used to cure the monomer solutions using 30 s exposure time and 400 W power setting. TA Instruments Differential Scanning Calorimeter (DSC 2910) was used to determine the heat of cure (J/g) during polymerization at 37 °C, from which molar heat of cure (kJ/mole) and %Conversion values were estimated.
Results: Range of mean values as a function monomer selections were: heat of cure (J/g): 161.7 for 70PT/DPO system to 198.6 for 50BT/CQ system; molar heat of cure (kJ/mole): 67.3 for 70BT/DPO to 78.86 for 50PT/CQ; % conversion: 59.9 for 70BT/DPO to 70.3 for 50PT/CQ. Analysis of variance and Tukey HSD pairwise contrast showed statistically significant differences between % conversion means of PEM and BisGMA mixtures, with PEM mixtures showing significantly higher mean values.
Conclusions: The results suggest that PEM-665 is a promising candidate material for dental polymer applications.
Introduction
Dental composites are the mainstay of esthetic restorative materials used in dentistry. Despite its widespread use in restorative dentistry, the limitations of composite resin technology are also widely recognized. Many brands of composites use dimethacrylate monomers for the composites. One of the primary reasons for such use is the ease with which they can be cured by visible light activation, a convenient and popular chair-side dental restorative procedure. The composite resins comprise a resin monomer and filler particles, and when the monomer is cured through light activated curing agents, it becomes the matrix in which the filler particles are dispersed and embedded. Most of the important properties of the composites are determined by the choice of the monomer, filler particulate composition, size and size distribution of filler particles as well as the efficacy of the filler-resin interfacial bonds. Although there have been significant improvement and diversification of monomer and filler choices in recent years,[Citation1–4] BisGMA, developed by the pioneering work of Bowen,[Citation5] is still one of the more popular dimethacrylate resins extensively used in dentistry. Bis-GMA is however derived from Bisphenol-A (BPA). BPA is considered as a xenoestrogen, and it belongs to a group of chemicals termed as “endocrine disruptors” that are able to disrupt the chemical messenger system in the body.[Citation6–11] There is growing international concern about man-made endocrine disrupting chemicals. Therefore, there is considerable interest in finding new monomers not derived from BPA, but at the same time retains the facile light curing characteristics of dimethacrylate moiety.
Recently, a cycloaliphatic polyester dimethacrylate oligomer (PEM-665, hereafter referred to as only PEM) has been introduced commercially by Designer Molecules Inc. (San Diego, CA) as a new monomer for dental applications. A comparison of the monomer structural features appears to indicate distinct differences between the structures of PEM and BisGMA monomers. The monomer structures are shown in . The main differences are (a) the presence of hydroxyl group in the BisGMA monomer, but not in PEM (b) the presence of the bulky aromatic groups in BisGMA, but not in PEM and (c) the presence of cycloaliphatic groups in PEM, but not in BisGMA. PEM, as the structure indicates, is also not a derivative product of BPA. In addition to these chemical structural differences, there are other differences. The molecular weight of PEM monomer (M.W.:665) is higher than that of BisGMA monomer (M.W.:512). The viscosity of PEM (η = 3.0 Pas) is less than half of that of bisGMA (η = 7.2 Pas) at 50 °C.[Citation12]
These structural and property characteristics indicate that PEM is potentially a viable alternative to the BisGMA monomer for dental composite resins. Unlike BisGMA, and other aliphatic monomers such as urethane dimethacrylates, there is only limited work in cycloaliphatic systems. Podgórski[Citation13] recently reported synthesis and characterization of novel dimethacrylate monomers with bulky bicycloaliphatic rings and revealed promising results for their potential use as dental monomers with improved conversion and reduced shrinkage. A number of other articles have also recently appeared in which dimethacrylate formulations not derived from bis-phenol A have been characterized as alternatives to BisGMA.[Citation14–22] The main focus in these investigations was to improve conversion, reduce shrinkage and/or eliminate bis-phenol A as one of the source materials. Promising results have been reported in the target objectives in most systems. PEM is a new cycloaliphatic polyester dimethacrylate recommended for dental applications, but there is little published documentation of its feasibility for use as a dental monomer. For convenient use in dentistry, any candidate monomer for direct restorative use must be readily polymerizable by visible light activation. In this study, our goal was to characterize and evaluate PEM curing response to typical light activation conditions used in chair-side curing of commercial dental composite resins. The light activated curing characteristics of PEM were investigated by measuring the heat of cure during polymerization. BisGMA was used as a control. We used the same diluent monomer triethylene glycol dimethacrylate (TEGDMA) for comparison. The main variables considered were two monomers, two monomer/diluent ratios and two initiators. Molar heat of cure and % conversion were estimated under standardized conditions of light cure. The following hypotheses were tested:
There are significant differences in molar heat of cure and % conversion between the two monomers (with typical monomer/diluent ratios) under identical light activation conditions.
There are significant differences in the molar heat of cure and % conversion of the monomers as a function of initiator differences under identical light activation conditions.
Materials and methods
Materials
lists the materials used. PEM and BisGMA were evaluated as the primary monomers. TEGDMA, a monomer used in many commercial composite resins, was used as a diluent monomer for both. shows the monomer molecular structures. Two monomer/diluent proportions (70/30 and 50/50 by weight) were used for the comparison. These monomer mixtures are designated 70PT and 50 PT for solutions containing PEM-TEGDMA, and 70BT and 50BT for those containing BisGMA and TEGDMA, respectively. Camphoroquinone (CQ) with ethyl-4-dimethylaminobenzoate (EDMAB) as co-initiator, and diphenyl(2,4,6-trimethoxybenzoyl) phosphinoxide (DPO) were evaluated as visible light photo initiators for both monomer groups. 1(w)% Initiator was added in both cases.
Table 1. Materials.
Experimental method for heat of cure
A TA Instruments (New Castle, DE) DSC model 2190 was used to study the heat of cure during light-activated polymerization. The curing light was mounted on a supporting stand with a clamp and the light tip was placed on top of the open DSC cell as shown in . Such an arrangement standardized the uniformity of the light exposure conditions throughout the experiments. About 25–30 mg of the monomer solution containing initiator was placed in the aluminum sample pan of the DSC cell. A similar blank aluminum pan was used as the reference pan. The temperature during polymerization was maintained at 37 °C by programmed temperature control of the DSC by the Thermal Advantage Program. A VIP light cure unit (BISCO, Schaumberg, IL), at 400 W power setting, was used for light curing. Experiments were done with four successive 30-s light exposures to follow heat-flow (W/g) during and after initial polymerization, as shown in . The exotherm during the first light exposure included heat of polymerization and any heat generated by the light itself. To correct for the heat from the light, three additional light exposures were used to get an average exotherm from light alone. The heat of cure was determined by the difference between the first exotherm and the average of the exotherms from the next three light exposures. A total sample size of N = 24 was used.
Figure 2. DSC set up for heat of cure measurements. The VIP light is mounted on a stand with the light tip at the top of the DSC cell. The light tip was maintained at the same distance from the sample pan to ensure uniform light exposure conditions during all experiments.
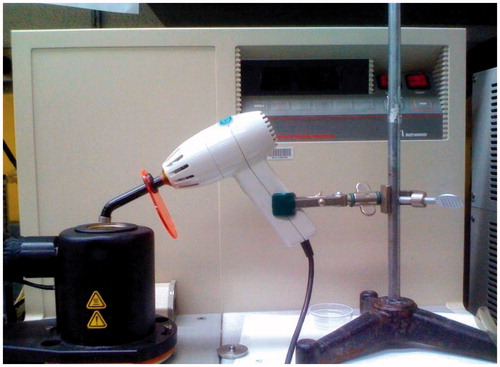
Figure 3. Heat flow(W/g) change as a function of light exposure time. The first exotherm represents the heat generated (J/g) by monomer conversion as well as the heat contributed directly by the light. The subsequent exotherms show approximately the same amount of heat and is associated only with light.
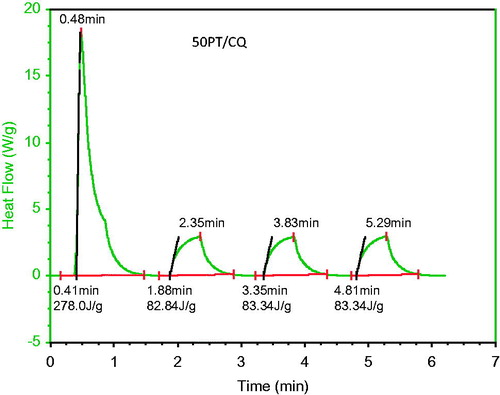
Calculation of molar heat of cure and % conversion
The equivalent molecular weight of a monomer mixture was estimated using the mole-fractions of the monomers in the mixture. The molecular weights of the monomers are PEM = 665, BisGMA = 512 and TEGDMA = 286. Mole fractions of the monomers in the monomer groups studied were estimated from weight fractions using the formula below:
Mf of monomer B in the same mixture = 1 − Mf of A.
The estimated mole fractions for the different monomer groups were:
70 BT: BisGMA 0.566, TEGDMA 0.434; 50BT: BisGMA 0.358, TEGDMA: 0.642;
70 PT: PEM 0.501, TEGDMA 0.499; 50PT: PEM 0.30, TEGDMA 0.70.
Based on these mole fractions, equivalent molecular weights of the monomer mixtures were calculated by assuming the rule of additive mixtures (assuming no interactive effects in the heat of polymerization of monomer mixtures):
70 BT: (0.566 × 512 + 0.434 × 286) = 413.9
50 BT: (0.358 × 512 + 0.642 × 286) = 366.9
70 PT: (0.501 × 665 + 0.499 × 286) = 475.9
50 PT: (0.30 × 665 + 0.70 × 286) = 399.7
The molar heat of cure for each monomer mixture was estimated by multiplying the heat of cure (J/g) with the equivalent molecular weight of the monomer mixture and expressing it in kJ/mole.
The molar heat of cure for 100% conversion of each methacrylate group, evaluated for a series of monomers, is 56 kJ/mole.[Citation23] Since we used dimethacrylate monomers, the heat of cure for 100% conversion was assumed as 112 kJ/mole. The fraction of the experimentally determined molar heat of cure for each dimethacrylate monomer mixture to that of the heat of cure for 100% conversion of a dimethacrylate monomer (112 kJ/mole), when expressed as a percentage gives us the % conversion for the respective monomer mixture, and this was also estimated.
Statistical analysis
The estimated % conversion data were analyzed by two-way analysis of variance (ANOVA) with monomer group and initiator system as independent variables (). Significant differences between means of % conversion levels were evaluated at a significance level of α = 0.05, both as function of monomer groups and initiator systems. When ANOVA results confirmed significant differences between means, pairwise Tukey–Kramer contrast was also used to test significant differences between individual groups. Statistical analysis was done with JMP statistical Program version 10 (SAS Statistical Institute, Corey, NC).
Table 2. Summary of ANOVA results.
Results
is a bar graph of the means and (SD) of the heat of cure (J/g) data. The heat of cure data shows higher values for monomer mixtures with higher diluent proportions in both BisGMA and PEM groups. Since the molecular weight of the diluent monomer is significantly lower than that of the primary monomer in both PEM and BisGMA systems, the heat of cure values expressed on per gram basis is expected to be higher for diluent-rich mixtures.
Figure 4. Bar graph of heat of cure (J/g) means and (SD) for different monomer mixtures. Note the higher heat of cure means associated with diluent-rich monomers, as expected.
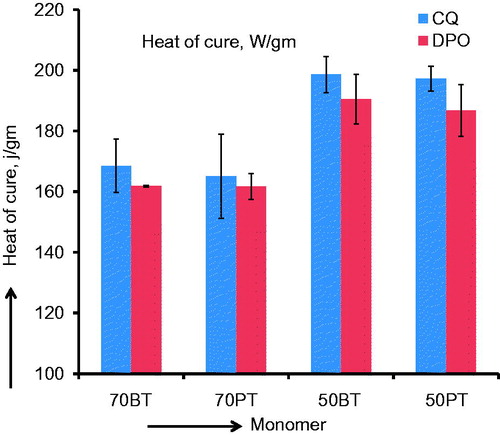
is a bar graph of the means and (SD) of the molar heat of cure (kJ/mole). The data now show that PEM-based monomer groups have higher molar heat of cure mean values in both CQ initiator-based subgroup and DPO initiator-based subgroup. The molar heat of cure values were converted to % conversion values by dividing the molar heat of cure data with the heat of polymerization for 100% conversion and expressing the fractions as percentage. is a bar graph of the % conversion means and (SDs). The bar graphs indicate that each PEM-TEGDMA mixture has higher % conversion mean than that of either BisGMA-TEGDMA means.
Discussion of results
The summary of a statistical two-way ANOVA is shown in . The results show significant differences only as a function of monomer systems (p = 0.0004). A Tukey–Kramer pairwise comparison of means due to main effects of monomers and initiators is shown in . It presents a comparison of means by mean diamonds and Tukey–Kramer comparison circles. The horizontal line between the mean diamonds is the line representing the grand mean of all data. The diagonal line of each mean diamond displays the mean % conversion of the group representing the variable (monomer in 7(a), initiator in 7(b)). The 95% confidence range is indicated by the lines parallel to the diagonal in each mean diamond. The Tukey–Kramer comparison circles are shown on the right in both . Significant differences are highlighted by the color difference of the circles for the groups. In , the comparison circles illustrate that while the monomer solutions within the BisGMA and PEM show no significant differences (p >> 0.05) in their mean % conversions, the inter-monomer group comparisons between BisGMA and PEM show significant differences (p < 0.05), as seen in . The estimated p values for significance comparison are also listed in the attached table in . The results support the acceptance of the first hypothesis that there is a significant difference between mean % conversions of PEM and BisGMA monomers. On the other hand, indicates that there is no statistically significant difference between the % conversion means of the monomers cured by CQ and those cured by DPO, and the results support the rejection of the second hypothesis. There was also no significant initiator*monomer interaction contribution (p ≈ 0.89), as seen in .
Figure 7. (A) Tukey–Kramer contrast of mean % conversion means of different monomers (ignoring initiator differences). Note that PEM resins have statistically higher mean % conversion than BisGMA (B) Tukey–Kramer contrast of mean % conversion means based only on initiators (without differentiating monomers and monomer/diluent proportions). Note that overall % conversion is similar for CQ and DPO.
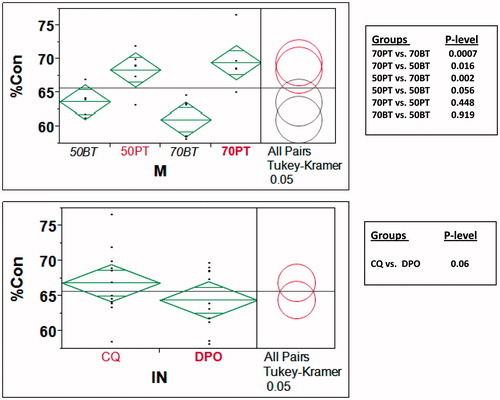
Figure 8. Profile plot of interactive effects of monomer/diluent proportions and initiators. There is no interactive effect at different levels of monomer/diluents ratios.
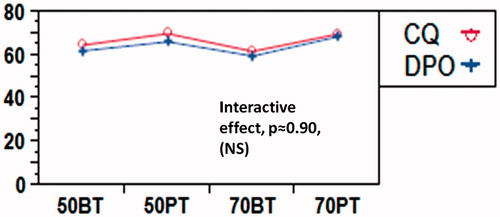
The results of this study agree with previous reports of relatively lower conversion levels in BisGMA-based resins, but there are no previous published data on conversion in PEM that can be used for comparison. It is well established in the literature that the structure of BisGMA is a limiting factor that adversely affects its degree of polymerization. In fact, Sideridou et al. [Citation24] have shown that the % conversion in neat BisGMA is capped at 39% as the upper limit. The hydroxyl groups in the BisGMA structure are positioned diametrically across the rigid bisphenol core structure containing the bulky aromatic groups, and this rules out any intra-molecular hydrogen bonds.[Citation25] For this reason, inter-molecular bonds form in the uncured state, leading to association between molecules forming a very viscous hydrogen bonded “quasi-network” structure with highly impaired mobility for the molecules.[Citation25] The addition of TEGDMA significantly improved the conversion levels from 39% for neat BisGMA to the 60% range for BisGMA-TEGDMA mixtures because of the plasticizing effect of TEGDMA on BisGMA, as seen in the lowering of Tg from −7.3 °C for neat BisGMA to the range of (−47 °C to −61 °C) in BisGMA-TEGDMA mixtures.[Citation24] Ferracane et al. had also previously shown enhanced conversion in BisGMA-based resins with the addition of TEGDMA.[Citation26] The % conversion levels for BisGMA-TEGDMA mixtures in this study (60–65%) are close to the values reported in these and other past reports.
Interestingly, the mobility problem in BisGMA oligomer also limits termination events during polymerization because it is difficult to bring together radicals attached to rigid molecular chains in a network, thus limiting rapid termination by combination or disproportionation.[Citation24] The presence of unreacted radicals in the network and residual unreacted monomer molecules in the system facilitates a slow dark cure process, and it is well known that BisGMA undergoes such a dark cure for a few days. It is also noteworthy that ambient temperature during polymerization significantly influences % conversion in the BisGMA resins because of the temperature effect on mobility.[Citation27]
Thus, PEM is clearly an important alternative candidate dimethcrylate monomer from visible light activation point of view. Designer molecules claim that it has a significantly lower shrinkage also (4.8% for neat PEM vs. >6% for neat BisGMA, both after 100% conversion). The refractive index of PEM (n = 1.51) is well within the appropriate or modifiable range for use with selected commercially available filler materials (e.g. strontium borosilcate, n = 1.50). The absence of hydroxyl group in its structure makes it more hydrophobic vis-à-vis BisGMA that contains hydroxyl groups. The higher hydrophobicity may be an important advantage to reduce water sorption and to protect the ester groups in the PEM structure from hydrolysis because it helps to repel water responsible for hydrolysis. The higher molecular weight of PEM is conducive to reducing polymerization shrinkage. A recent report in the American Dental Research Association conference has reported reduced water sorption/solubility and lower shrinkage for PEM vis-à-vis BisGMA monomer systems, in keeping with these structural differences (Esquibel et al., Abstract #1026, New monomers as alternatives to BisGMA, AADR conference, 2011, Tampa, FL). The reduction in viscosity is especially important because the lower viscosity of PEM (relative to BisGMA) may help facilitate use of lower diluent levels, and help reduce shrinkage further. For example, a PEM/TEGDMA weight % ratio of 75:25 corresponds to 0.565 mole fraction of PEM, roughly equal to the mole fraction of 0.566 estimated for BisGMA in the 70:30 weight % ratio of BisGMA/TEGDMA formulation. Thus, when equivalent monomer/diluent mole fractions are considered, the use of PEM/TEGDMA can be done at a higher weight fraction ratio with a lower viscosity and higher equivalent molecular weight, thus promoting better working consistency in the monomer and reduced shrinkage in the polymer.
Polymers derived from oligomers with bulky aromatic groups have been reported in the past to form more rigid and brittle structures, while oligomers with aliphatic/cycloaliphatic sequences create a more pliant and tougher structure.[Citation28] The strength and brittleness in BisGMA resin is also presumably significantly aided by the hydrogen bonding (due to hydroxyl groups) in the polymer network.[Citation29] Brittleness promotes fatigue failure under repetitive loading typical of chewing and grinding. The network structure in PEM is expected to be less brittle and tougher than BisGMA under masticatory functional cycles.
There is, however, concern in the use of TEGDMA as a diluent monomer. TEGDMA is a leachable and degradable component in many current generation composites, and may present toxicological concerns of its own.[Citation30–34] The TEGDMA structure is shown in . TEGDMA is recognized to be somewhat hydrophilic and this may partially account for its degradable properties. When considering PEM as a candidate monomer for dental applications, an alternative diluent monomer is desirable. An alternate diluent monomer is 1,6 hexanedioldimethacrylate (HDDMA), and this has been shown to be compatible for use with PEM in our preliminary studies. The structure of HDDMA is shown below:
With fewer hetero atoms in its structure than TEGDMA (which is considered as somewhat hydrophilic), HDDMA is more hydrophobic and may help resist hydrolytic degradation. The methylene (−CH2−) group sequence can help add conformational freedom to chain segments and improve flexibility to the matrix network, increasing its fracture toughness.
PEM in conjunction with a proper diluent monomer is thus a very promising candidate for dental application based on visible light cure characteristics, and further research is important to characterize its mechanical, biocompatibility and clinical performance characteristics. It is also to be noted that significant improvements in overall dental composite performance requires not only improved monomers, but also optimum filler particle size and size range as well as its uniform dispersion. Nanofiller technology is now becoming increasingly used in dental composites, but the developing polymer brush technology using advanced polymerization techniques such as Atom Transfer Radical polymerization and Reversible Addition Fragmentation Transfer polymerization are additional methodologies available for enhancing filler-matrix bonding and filler dispersion in nano-composites.[Citation35,Citation36] Finally, better adhesion at the restoration–tissue interface is also critical.
Conclusions
Cycloaliphatic polyester dimethacrylate monomer PEM is a very promising candidate material for dental composite applications.
The % conversion of PEM-TEGDMA monomer system during light cure is better than that of similar BisGMA-TEGDMA monomer system.
There is no apparent statistical or clinically significant difference between the initiators CQ and DPO on monomer conversion in PEM- and BisGMA-based monomers.
Declaration of interest
A declaration of interest statement reporting no conflict has been inserted. Please confirm the statement is accurate.
References
- Cramer NB, Stansbury JW, Bowman CN. Recent advances and developments in composite dental restorative materials. J Dent Res. 2011;90:402–416
- Moszner N, Salz U. New developments in polymeric dental composites. Prog Polym Sci. 2001;26:525–576
- Chen MH. Update on nanocomposites. J Den Res. 2010;89:549–560
- Ferracane JL. Current trends in dental composites. Crit Rev Oral Bio. 1995;6:302–318
- Bowen RL. Dental filling material comprising vinyl silane treated fused silica and a binder consisting of the reaction product of bisphenol and glycidyl acrylate. United States patent US 3,066,112. 1962 Nov 27
- Olea N, Pulgar R, Perez P, Olea-Serrano F, Rivas A, Novillo-Fertrell AN, et al. Estrogenicity of resin-based composites and sealants used in dentistry. Environ Health Perspect. 1996;104:298–305
- Pulgar R, Olea-Serrano F, Novillo-Fertrell AN, Rivas A, Pazos P, Pedraza V, et al. Determination of bisphenol A and related aromatic compounds released from bis-GMA-based composites and sealants by high performance liquid chromatography. Environ Health Perspect. 2000;108:21–27
- Hugo ER, Brandebourg TD, Woo JG, Loftus J, Alexander JW, Ben-Jonathan N. Bisphenol A at environmentally relevant doses inhibits adiponectin release from human adipose tissue explants and adipocytes. Environ Health Perspect. 2008;116:1642–1647
- Alonso-Magdalena P, Morimoto S, Ripoll C, Fuentes E, Nadal A. The estrogenic effect of bisphenol A disrupts pancreatic β-cell function in vivo and induces insulin resistance. Environ Health Perspect. 2006;114:106–112
- Lang IA, Galloway TS, Scarlett A, Henley WE, Depledge M, Wallace RB, et al. Association of urinary bisphenol A concentration with medical disorders and laboratory abnormalities in adults. J Am Med Assoc. 2008;300:1303–1310
- Soderholm KJ, Mariotti A. BIS-GMA-based resins in dentistry: are they safe? J Am Dent Assoc. 1999;130:201–209
- Andrzejewska E, Marcinkowska A. New aspects of viscosity effects on the photo-polymerization kinetics of the 2,2-bis[4-(2-hydroxymethacryloxypropoxy)-phenyl]propane/triethylene glycol dimethacrylate monomer system. J Appl Polym Sci. 2008;110:2780–2786
- Podgórski M. Synthesis and characterization of novel dimethacrylates of different chain lengths as possible dental resins. Dent Mater. 2010;26:e188–e194
- Liang X, Liu F, He J. Synthesis of none bisphenol A structure dimethacrylate monomer and characterization for dental composite applications. Dent Mater. 2014;30:917–925
- Podgórski M. Structure-property relationship in new photo-cured dimethacrylate-based dental resins. Dent Mater. 2012;28:398–409
- Cramer NB, Couch CL, Schrek KM, Carioscia JA, Boulden JE, Stansbury JW, et al. Investigation of thiol-ene and thiol-ene-methacrylate based resins as dental restorative materials. Dent Mater. 2010;26:21–28
- Carioscia JA, Lu H, Stansbury JW, Bowman CN. Thiol-ene oligomers as dental restorative materials. Dent Mater. 2005;21:1137–1143
- Boulden JE, Cramer NB, Schreck KM, et al. Thiol-ene-methacrylate composites as dental restorative materials. Dent Mater. 2011;27:267–272
- Andreani L, Silva LL, Will MA, Meter M, Joussef AC, Soldi V. Development of dental resinous systems composed of bisphenol A ethoxylated dimethacrylate and three novel methacrylate monomers: synthesis and characterization. J Appl Polym Sci. 2013;128:725–734
- He J, Liu F, Valittu PK, Lassila LV. Synthesis and characterization of new di-methacrylate monomer and its application in dental resin. J Biomater Sci Polym Ed. 2013;24:417–430
- Kim JW, Kim LU, Kim CK, Cho BH, Kim OY. Characteristics of novel dental composites containing 2,2-bis[4-(2-methoxy-3-methacryloyloxy propoxy) phenyl] propane as a base resin. Biomacromolecules. 2006;7:154–160
- Reinelt S, Tabatabai M, Moszner N, Fischer UK, Utterodt A, Ritter H. Synthesis and photopolymerization of thiol-modified triazine-based monomers and oligomers for the use in thiol-ene-based dental composites. Macromol Chem Phys. 2014;215:1415–1425
- Davis TP. Polyacrylates. In: Olabisi O, Adewale K, editors. Handbook of thermoplastics. New York: Marcel Dekker Inc.; 1997. p. 206
- Sideridou I, Tserki V, Papanastasiou G. Effect of chemical structure on degree of conversion in light-cured dimethacrylate-based dental resins. Biomaterials. 2002;23:1819–1829
- Davy KWM, Kalachandra S, Pandain MS, Braden M. Relationship between composite matrix molecular structure and properties. Biomaterials. 1998;19:2007–2014
- Ferracane JL, Greener EH. The effect of resin formulation on the degree of conversion and mechanical properties of dental restorative resins. J Biomed Mat Res. 1986;20:121–131
- Vaidyanathan J, Vaidyanathan TK. Interactive effects of resin composition and ambient temperature of light curing on the percentage conversion, molar heat of cure and hardness of dental composite resins. J Mater Sci Mater Med. 1992;3:19–27
- Kunal K, Robertson CG, Pawlus S, Hahn SF, Sokolov AP. Role of chemical structure in fragility of polymers: a qualitative picture. Macromolecules. 2008;41:7232–7238
- Lemon MT, Jones MS, Stansbury JW. Hydrogen bonding interactions in methacrylate monomers and polymers. J Biomed Mater Res A. 2007;83:734–746
- Seiss M, Track N, Hickel R, Reichl FX. In vitro stability of methacrylic acid, TEGDMA and HEMA exposed to esterases. Dent Mater. 2009;25:1044–1049
- Geurtsen W, Leyhausen G. Chemical–biological interactions of the resin monomer triethyleneglycoldimethacrylate (TEGDMA). J Dent Res. 2001;80:2046–2050
- Emmler J, Seiss M, Kreppel H, Reichl FX, Hickel R, Kehe K. Cytotoxicity of the dental composite component TEGDMA and selected metabolic by-products in human pulmonary cells. Dent Mater. 2008;24:1670–1675
- Gregson KS, Terrence O'Neill J, Platt JA, Jack Windsor L. In vitro induction of hydrolytic activity in human gingival and pulp fibroblasts by triethyleneglycol dimethacrylate and monocyte chematactic protein-1. Dent Mater. 2008;24:1461–1467
- Imazato S, Horikawa D, Nishida M, Ebisu S. Effects of monomers eluted from dental resin restoratives on osteoblast-like cells. J Biomed Mater Res Part B: Appl Biomater. 2009;88:378–386
- Werne TV, Patten TE. Atom transfer radical polymerization from nanoparticles: a tool for the preparation of well-defined hybrid nanostructures and for understanding the chemistry of controlled/“living” radical polymerizations from surfaces. J Am Chem Soc. 2001;123:7497–7505
- Zhao Y, Perrier S. Synthesis of well-defined homopolymer and diblock copolymer grafted onto silica particles by Z-supported RAFT polymerization. Macromolecules. 2006;39:8603–8608