ABSTRACT
Volatile organic compounds (VOCs) emitted from surface coatings have caused growing public concern for air quality. Even the low-emitted VOC impact from water-based paints on indoor air quality in urban areas has caused concern. This paper presents experimental data using a mathematical model to simulate dynamic VOC emissions from water-based paints that is based on mass transfer and molecular diffusion theories. A series of field-analogous experiments were carried out to continuously measure the VOCs emitted from two typical water-based paints using a gas chromatography–flame-ionization detector monitor in an artificial wind tunnel system. In the study cases, the mass flux of VOCs emitted from the water-based paints was up to 50 μg/m2sec. It was found that the time needed to completely emit VOCs from water-based paints is just hundreds of seconds. However, the order of magnitude of the VOC emission rate from water-based paints is not lower than that from some dry building materials and solvent-based paints. The experimental data were used to produce a useful semiempirical correlation to estimate the VOC emission rates for water-based paints. This correlation is valid under appropriate conditions as suggested by this work with a statistical deviation of ±7.6%. With this correlation, it seems feasible to predict the dynamic emission rates for VOCs during a painting process. This correlation is applicable for assessing the hazardous air pollutant impact on indoor air quality or for environmental risk assessment. Associated with the dynamic VOC emission characterization, the air-exchange rate effect on the VOC emission rates is also discussed.
This paper presents experimental data using a mathematical model to simulate dynamic VOC emissions from water-based paints that is based on mass transfer and molecular diffusion theories. A semiempirical correlation is suggested to estimate the VOC emission rates for water-based paints. With this correlation, it is feasible to interpret and predict the dynamic emission rates for VOCs during a painting process. The results obtained in this work will be useful in evaluating the indoor air quality impact by primary VOCs or hazardous air pollutants emitted from water-based paints.
INTRODUCTION
Volatile organic compounds (VOCs) are known to degrade human health and contribute secondary pollutants such as photochemical oxidants and suspended particulate matter. Although the potential harmful effects of individual VOCs have not been evaluated completely, some VOCs such as benzene and butadiene have various reported toxic activities.Citation1 The hazardous effects of identified and quantified VOCs and their dynamic emission rates are required to elucidate their toxic potencies. VOCs emitted from surface coatings have caused growing public air quality concern. Even low-emitted VOCs from water-based (or water-soluble) paints affect the indoor air quality in urban areas.Citation2–5 Although the direct impact of VOCs emitted from paints is short lived, lasting no more than a few hours, some VOCs will affect the ambient air quality in the early painting stage, especially the indoor air quality during the painting process. Paints have been shown to emit significant amounts of VOCs into the atmosphere, particularly during the painting process. Some components in these emissions are highly reactive and may contribute to photochemical smog. Paint manufacturing and paint utilization have received considerable attention from regulatory bodies. The primary VOCs in water-based paints are alcohols and esters.Citation6 The VOCs used in the water-based paints include film-forming agents (e.g., texanol and cellosolve) and antifreezing agents (e.g., glycols).Citation7 As a resulting of the U.S. Clean Air Act Amendments of 1990, environmental regulatory agencies have begun to more closely scrutinize industrial sources of hazardous air pollutants. There has been growing public concern about air quality in urban areas.Citation8 The Taiwan Environmental Protection Agency announced the Indoor Air Quality Act (IAQA) in 2008 and implemented some initiatives to control the domestic VOC emission inventory.
Many predictive models have been developed based on simplified and reliable methods for estimating VOC emissions from VOC sources.Citation9–21 Most of these studies have been conducted on VOC emissions from dry building materials. For example, Low et al.Citation9 studied the effects of airflow on VOC emissions from carpet-adhesive assemblies. Lee et al.Citation10 studied the VOC source and sink behavior in porous building materials. Hung and HaghighatCitation11 developed VOCs emission modeling for dry building materials. Afshar et al.Citation14 used three small chamber test methods to measure the VOC emission rate from paint. The film thickness has a pronounced effect on the initial chemicals emitted from a paint layer. The three chambers suggest that it is possible to estimate how long VOCs emit after the start of the emission test. Deng and KimCitation17 adopted a new analytical model for VOC emissions that considered the mass transfer through the air boundary layer and the diffusion in the building material. They found that the gas-phase mass transfer coefficient has a great effect on the concentration in the air and the emission flux in the early painting stage. Hu et al.Citation19 developed an analytical mass transfer model to provide a powerful tool for predicting VOC emissions from composite materials such as furniture and layered wall structures. Qian et al.Citation21 proposed a relationship among the air-exchange rate, loading ratio, air velocity, and the VOC emission rate. The relationship conveniently reveals the impact of these factors on different emission stages.
Some researches studied VOC emissions from wet material.Citation22–26 Yang et al.Citation22 studied a mass transfer model for simulating VOC emissions from wet coating materials applied to absorptive substrates. Haghighat and ZhangCitation24 proposed a model to estimate the gas-phase mass transfer coefficient for VOC emissions from varnish painting. Lee et al.Citation25 studied the wet film thickness effect on VOC emissions from a finishing varnish. Zhang and Niu indicated that solvent-based interior coating materials were recognized as a major source of VOCs in the indoor environment.Citation26 In the emission process, the substrate acts as a secondary source. The sink effects have been studied with a detailed mass transfer model considering convective mass transfer in airstreams, the VOC diffusions in painting film, and the sorption and diffusions of VOCs in substrates. Li et al.Citation27–29 used the field and laboratory emission cell to investigate the VOC emissions from commercially available water-based emulsion paint. Nakashima et al.Citation7 developed an analytical method for VOCs used as film-forming agents or antifreeze agents in water-based paints using flame-ionization detection (FID)–gas chromatography (GC) and GC–mass spectrometry. Brun et al.Citation30 studied the film-formation process of several coating systems (water-based and solvent-based) using a new optical technology based on diffusion-wave spectroscopy. They found that the film formation kinetics, displayed in real time, provided a new vision of the successive steps in the mechanisms taking place (evaporation, packing, etc.) as well as accurate information on drying times (e.g., open time) for an in-depth characterization of the film-formation process. Recently, AltinkayaCitation23 predicted the emission characteristics of VOCs from wet surface coating.
However, most of those researches or prediction models for wet materials focused on solvent-based paint emission factors. Few researchers studied water-based paints, especially the dynamic emission rate during the painting process. They also recommended that the VOC emissions from water-based paints should be included in the indoor and urban emission inventories so that more accurate air quality predictions can be determined. Few studies exist on dynamic VOC emissions during painting for water-based paints. This paper presents experimental data using a mathematical model to simulate dynamic VOC emissions from water-based paints that is based on mass transfer and molecular diffusion theories. The results are expected to be useful in evaluating the indoor air quality impact using primary VOCs emitted from water-based paints.
THEORETICAL BACKGROUND
VOC emissions are highly dependent on their fugitive sources and ambient conditions. Their emission rates may be derived or estimated using theoretical models. Some of the possible pathways for VOC emissions have been explored.Citation31–34 It is generally believed that the emission process has three phases.Citation35 The first phase represents the period shortly after the paint material is applied and is still relatively wet. High emission rates but fast decay characterizes the VOCs emitted in this phase. The emissions in this phase are related to evaporation at the surface of the paint material. In the second phase, the paint material dries as the emission mode changes from an evaporation-dominant phase to an internal-diffusion controlled phase. In the final (third) phase, the paint material becomes relatively dry, the VOC off-gas rate decreases, and this process lasts for a long time. Therefore, the emission fundamentals include molecular diffusion, mass transport by convection, and organic characterization present in the paint material.Citation21,Citation36–38 Although all of these mechanisms have been known for several years, the detailed dynamic emission process has yet to be clarified for VOCs emitted during the coating process for water-based paints. The theoretical model used in this study assumes that VOCs diffuse through wet-phase paint and then emit into the ambient air. As shown in , the transient VOC diffusion can be described using a one-dimensional diffusion equation for thin-layer paint with homogeneous diffusivity.
The boundary condition at the paint-air interface is imposed by accounting for the surface emission as follows:
The VOC concentration (C a) in the air can be solved by mathematical analysis with the equations described above. The mass flow rate of VOCs emitted from paints is thereby also calculated by setting m = C a NV , and the dimensionless emission rate (M) is calculated using M = m/D pδC 0. Therefore, M is dependent on the paint material properties (e.g., D p), convective mass-transfer coefficient (h), air-exchange rate (N), paint layer thickness (δ) elapsed time (t), etc. All of the variables in this model can be determined by experiments or are offered in the literature. Some researchers have presented a fully analytical solution to these equations and gave an exact solution.Citation19 The normalization analysis was conducted referring to these analytical methods and shows that the dimensionless emission rate (M) can be correlated into three dimensionless groups: the Biot number for mass transfer, Bio (= hδ/D p); the Fourier number for mass transfer, Fom (=D p t/δ2); and the dimensionless air-exchange rate, Aer (= Nδ2/D p). In other words, the model presented in this work concerns four dimensionless groups: M, Bio , Fom, and Aer.
EXPERIMENTAL METHODS
A field-analogous tunnel system was used to obtain VOC concentration, as shown in , which shows a schematic layout of the tunnel system containing four parts: a wind tunnel, a painted slab, a VOC monitor, and an air supplier with velocity controller. The wind tunnel was made of acrylic resin in a semielliptical shape. At the wind tunnel entrance, a rectification buffer was installed to prevent the entrance effect. The buffer was long enough to develop an effective airflow stream. The tunnel fundamental hydrodynamics were studied to verify that it characterized the wind in a controllable manner as in the other previous works.Citation40 A series of experiments were conducted in the wind tunnel. Two typical domestic water-based paints were used in this work. The major VOCs in these two paints are n-butyl alcohol, ethylene glycol, and C7 ester as listed in , where the VOC concentrations in the paints are those after blending with water. A small quantity of water is typically used as a solvent while painting. The table also lists the operating conditions, including air-exchange rates and paint layer thicknesses used in this study. A melamine resin slab 60 cm in length, 15 cm in width, and 5 mm in thickness, was used as the medium coated by paint. The work has made a reasonable assumption that there was no VOC sink effect in the melamine resin slab by theoretical estimation of diffusivity, which is also lower than that in paint layer at least by 2 orders of magnitude.
Table 1a. Properties of VOCs used in this work
Table 1b. Parameters used in this work
Online FID-VOC monitors (Perkin-Elmer, model CZHE-323) were used to continuously monitor the volatile organic concentrations in this work. The instrument was operated at 600 mL/min with an automatic flow rate controller. The sampling and analysis for the VOCs were followed using Taiwan standard method NIEA-A723.72B. A data acquisition system (DAS) was installed and connected with a personal computer to collect the dynamic experimental data. A single point measurement for VOC concentration was adopted in this work. Because the experimental apparatus was designed to allow the VOC concentration measured at the tunnel outlet to be well mixed, as shown in , it is reasonably assumed that there is a uniform VOC concentration at the measured point in the tunnel outlet where the airflow converges from a duct diameter of 0.21 m (equivalent) to 0.03 m. The eddy flow with disturbance propeller at the convergent point will mix (almost complete mixing) the VOC concentrations.
A painted slab was placed into the wind tunnel. The airflow was started up and controlled at a desired rate when the painted slab was placed in the wind tunnel. VOC monitoring and DAS were simultaneously carried out. The airflow rate was controlled at a desired constant value using an inverter to adjust the upwind fan frequency. A turbo flow meter (Davis Instruments) was used to monitor the airflow rate. The air velocity was then calculated by dividing the cross-sectional area of the tunnel outlet to the air volume flow rate measured at the tunnel outlet. The wind variation was tolerated within a narrow limit of ±2% mean air velocity. The relative humidity was 60.8–75.3% and the ambient temperature was 18–28 °C in this work.
RESULTS AND DISCUSSION
Experimental Data
The experimental data were systematically collected using the methods and procedures described above. The data precision was limited to a minimum of 95% for these experiments. The measured VOC concentrations (i.e., C a) varied approximately from zero up to 35 mg/m3 for butyl alcohol, up to 1.2 mg/m3 for ethylene glycol, and up to 4 mg/m3 for C7 ester. Typical dynamic VOC concentrations for N = 5 (1/hr) are shown in The VOC concentrations decreased with time through exponential decay because VOC depletion by evaporation will decrease the VOC concentrations in ambient air. The VOCs took a longer time to emit off when the paint thickness was increased. However, the time needed to completely emit VOCs off is just a few minutes.
Figure 3. Dynamic concentrations for different paint thicknesses in the case of N = 5 (1/hr): (a) PA-butanol, (b) PA-ester, (c) PB-butanol, and (d) PB-ethylene alcohol.
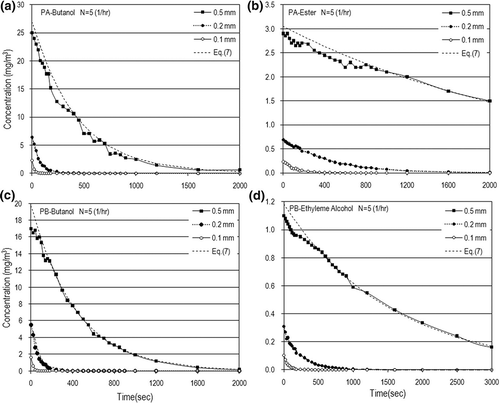
As shown in , the VOC concentrations decreased with an increase in air-exchange rate at the same elapsed time because a higher air-exchange rate or air velocity will dilute the VOC concentration but provide more driving potential to increase the mass-transfer rate. Basically, an increase in air velocity has a significant influence on the convective mass transfer and VOC concentration dilution. From the experimental results, the air velocity or air-exchange rate effect on the mass-transfer rate to enhance VOC concentration is weaker than that to dilute the VOC concentration. Another interesting finding is that the VOC concentrations increased with an increase in air velocity in the early painting stage. However, the VOC concentrations decay very fast and tend to be independent of the air velocity as time goes on. It reveals that the air velocity has a great effect on the VOC concentration only in the early stage. Over a longer time the air velocity has little influence on the VOC concentration. This means that the air velocity effect on the VOC concentration in the early painting stage is stronger than that in the end painting stage.
Mass Fiow Rate and Fiux
Mass flow rates (m) were calculated based on the measured data of VOC concentrations. shows the dynamic mass flow rates and mass flux in the N = 5 (1/hr) case. The VOC mass rates were in the range of few micrograms to hundreds of micrograms per second, with the mass flux up to 50 μg/m2sec. This figure also shows that the VOC mass flow rate increased with an increase in the paint thickness used. The air-exchange rate effect on the mass flow rate is obvious only in the early painting stage. After a longer time, the air-exchange rate has little influence on the mass flow rate and mass flux as shown in Eventually, the VOC mass flow rates decay very fast and tend to be independent of the air-exchange rate as time goes on. Although the time needed to completely emit VOCs from water-based paints is just hundreds of seconds, which is faster than those from solvent-based paints; the order of magnitude of the VOC emission rate from water-based paints is not lower that that from dry building materials (e.g., carpet)Citation11 and some solvent-based paints.Citation22,Citation25 This is why VOC pollution emitted from water-based paints is a concern especially in the early painting stage for indoor air quality.
Figure 5. Dynamic mass flow rates for different paint thicknesses in the case of N = 5 (1/hr): (a) PA-butanol, (b) PA-ester, (c) PB-butanol, and (d) PB-ethylene alcohol.
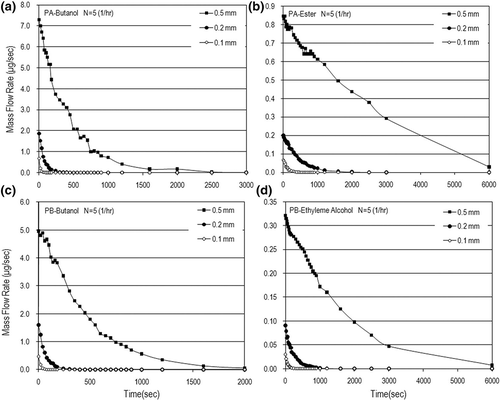
Figure 6. Dynamic mass flux for different paint thicknesses in the case of N = 5 (1/hr): (a) PA-butanol, (b) PA-ester, (c) PB-butanol, and (d) PB-ethylene alcohol.
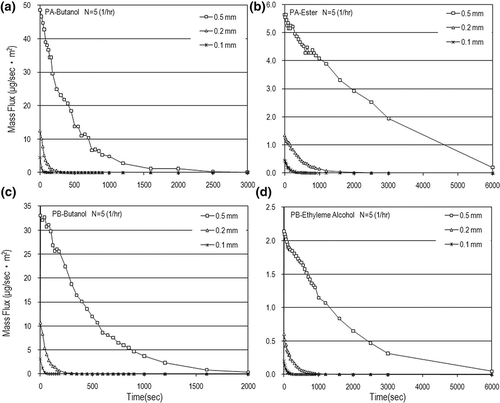
The dimensionless dynamic mass flow rates, which can be calculated from the data of m, are shown in and In the cases of this work, the dimensionless mass flow rates (M) are up to approximately 600,000 for the water-based paints used in this work.
Dimensionless Group Correlation
As proposed in the Theoretical Background section, dimensionless analysis based on the Buckingham л theory was adopted to acquire an appropriate relationship among the dimensionless groups M, Aer, Bio, and Fom.Citation41 The correlation is expressed as follows:
EquationEquation 7 illustrates the functional relationship between the dependent and independent variables. Statistically, there is a square correlation coefficient of 0.9106 and a standard deviation of 7.6% for this correlation if submitted under a 95% probability. The correlation shows that the data collected from these experiments disperses in a reasonable range of deviation from the regression equation, shown in , which shows the comparisonbetween the experimental data and the predicted results obtained in this work. The correlation indicates that the Bio effect is stronger than that of Aer on the VOC mass flow rate.
Figure 9. Dynamic dimensionless mass flow rates for different paint thicknesses in the case of N = 5 (1/hr): (a) PA-butanol, (b) PA-ester, (c) PB-butanol, and (d) PB-ethylene alcohol.
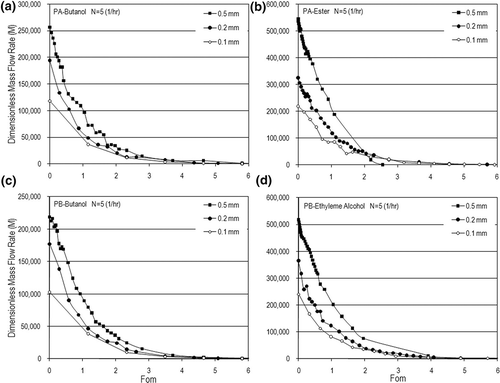
Figure 10. Dynamic dimensionless mass flow rates for different air-exchange rates in the case of δ= 0.2 (mm): (a) PA-butanol, (b) PA-ester, (c) PB-butanol, and (d) PB-ethylene alcohol.
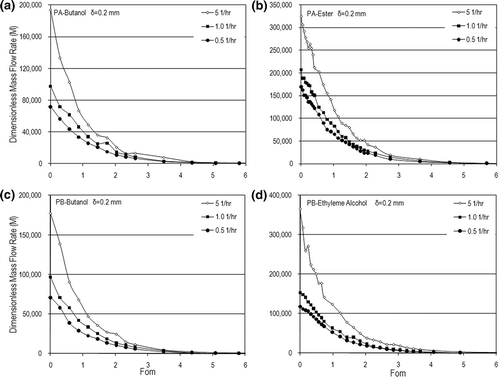
Figure 11. Comparison of dimensionless mass flow rates between experimental and predicted data: (a) PA-butanol, (b) PA-ester, (c) PB-butanol, and (d) PB-ethylene alcohol.
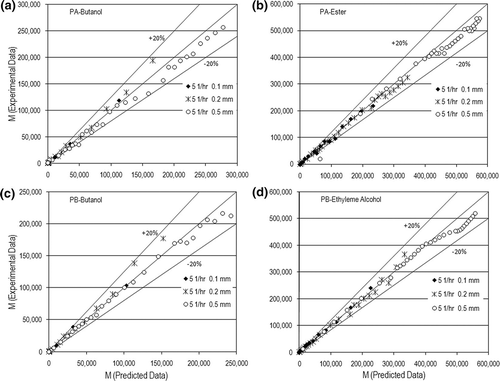
The load factor (ratio of paint volume to wind tunnel volume) is significant on the VOC emission although it does not appear in Equationeq 7. The initial C 0 concentration or paint layer thickness (δ) will increase with an increase in the load factor. If C 0 is constant, δ will increase with an increase in the load factor, as shown by Equationeq 7. The VOC emission or mass flow rate will increase with an increase in the load factor by the positive function of Equationeq 7. Consequently, the dimensionless emission rate (M) will increase with an increase in the load factor. In other words, the VOC emission rate increases with an increase in the load factor if C 0 is constant.
It was also found that there are some minor discrepancies in the VOC emission estimation in the early painting stage, corresponding to larger M values. These discrepancies result from mass transfer deviation because the paint material is still relatively wet in the early painting stage. At this stage, the mass transfer is dominated by evaporation at the paint material surface more than molecular diffusion in the interior paint material. The actual VOC emission mechanism from a painting process could be more complex than those adopted in this study, in which the mathematical model used is based on convective mass transfer and diffusion control. It is possible that the simplified model cannot completely govern the entire VOC emission mechanism, especially in the early painting stage. Similar discrepancies between the experimental and model estimated data were observed by others such as Qian et al.,Citation21 who also found overestimation in the initial VOC emission period. Fortunately, this error is gradually eliminated or even neglected as the elapsed time goes on.
Application Constraints
The results from the model presented here agree with the experimental data. However, there is an estimation deviation (∼7.6% in this work) by applying the correlation to estimate the VOC concentration emitted from the water-based paints. Also note that the constant 6.581 × 104 in Equationeq 7 (for the case presented in this work) might be significantly affected by the VOC characterizations and others not investigated in this study. EquationEquation 7 would overestimate the VOC concentrations in the air, especially in the early painting stage. In fact, many factors (e.g., the paint material and local meteorological conditions [likely humidity]) affect fugitive VOC emissions and thus the indoor air quality during the painting process. It may not be easy to fully analyze the complex emission mechanism in fugitive VOCs if one estimates only the VOC emission rates or concentrations. The potential problem with this work's approach is that Equationeq 7 does not generally correspond to all cases (i.e., the semiempirical approach could be flexible). There are some constraints to applying the results obtained from this work, as well as Equationeq 7, to a practical situation.
An examination of Equationeq 7 suggests some constraints must be considered. First, Equationeq 7 is proposed to be valid for the conditions N = 1/5 to 5 1/hr (∼0.02–10.21 cm/sec or ∼1.26 –12.6 cm/min) and δ= 0.1– 0.5 mm. More experimental data are necessary to confirm the validity of Equationeq 7 for other conditions. Second, the application would be limited for water-based paints with properties similar to those used in this work because Equationeq 7 was correlated using multivariable regression to estimate the VOC emission rate only for limited kinds of paints. More intensive work on other water-based paints with different characteristics would be better to verify these results. Third, meteorological sunlight and wind patterns usually have very strong seasonal dependencies if the correlation is applied to estimate VOC emission in an outside environment. The uncertainty error from Equationeq 7 due to these paint characteristics or meteorological features requires more exploration. In addition, the results obtained in this work would still be a subject of controversy if they are applied to an absorptive substrate because an absorptive substrate (e.g., particle board [PB] or cement) would act like a sponge, which lowers the peak VOC concentration but prolongs the presence of VOCs from the applied paints.Citation28
CONCLUSIONS
To estimate the dynamic VOC emission rates from water-based paints, this paper presented experimental data using a mathematical model to simulate dynamic VOC emissions from water-based paints that is based on mass transfer and molecular diffusion theories. A series of field-analogous experiments were carried out to continuously measure VOCs emitted from two typical water-based paints using a GC-FID monitor in an artificial wind tunnel system. In the cases presented here, the mass flux of VOCs emitted from the water-based paints was up to 50 μg/ m2sec. The time needed to completely emit the VOCs from water-based paints was just hundreds of seconds, but the order of magnitude of the VOC emission rate from water-based paints was not lower than those from some dry building materials and solvent-based paints. The VOC concentrations decay very fast and tend to be independent of the air velocity as time goes on. It was found that the dimensionless emission rate (M = m/D pδC0) can be correlated with three dimensionless groups: Biot number (Bio = hδ/D p), Fourier number (Fom = D p t/δ2), and dimensionless air-exchange rate (Aer = Nδ2/D p), which is valid in appropriate conditions suggested using work with a statistical deviation of ±7.6%. The correlation shows that the Bio effect is stronger than that for Aer on the VOC mass flow rate. Thus, with the dimensionless correlation suggested by this paper, it seems feasible to interpret and predict the dynamic emission rates for VOCs during a painting process. It was also found that there are some minor discrepancies for VOC emission estimation in the early painting stage because of mass transfer deviation and the application would be limited for water-based paints, which have properties similar to those used in this work. More experimental data are necessary to confirm the validity of the results obtained in this work for other conditions.
REFERENCES
- 2005 . WHO Air Quality Guidelines Global Update 2005: Report on a Working Group Meeting , Geneva , , Switzerland : World Health Organization .
- Haghighat , F. and Huang , H. 2003 . Integrated IAQ Model for Prediction of VOC Emissions from Building Material . Build. & Environ. , 38 : 1007 – 1017 .
- Chao , C.Y. and Chan , G.Y. 2001 . Quantification of Indoor VOCs in Twenty Mechanically Ventilated Buildings in Hong Kong . Atmos. Environ. , 35 : 5895 – 5913 .
- Chin , P. and Ollis , D.F. 2008 . Design Approaches for a Cycling Adsorbent/ Photocatalyst System for Indoor Air Purification: Formaldehyde Example . Journal of the Air & Waste Management Association , 58 : 494 – 501 . doi: 10.3155/ 1047-3289.58.4.494
- Tsai , C.W. , Chang , C.T. , Chiou , C.S. , Shie , J.L. and Chang , Y.M. 2008 . Study on the Indoor Volatile Organic Compound Treatment and Performance Assessment with TiO2/MCM-41 and TiO2/Quartz Photoreactor under Ultraviolet Irradiation . Journal of the Air & Waste Management Association , 58 : 1266 – 1273 . doi: 10.3155/1047–3289.58.10.1266
- Architectural Surface Coating . Emission Inventory Improvement Program Technical Report Series, Volume 3, Area Sources . Prepared for the Area Sources Committee; Emission Inventory Improvement Program by Radian Corporation . NC . Research Triangle Park .
- Nakashima , H. , Nakajima , D. , Takagi , Y. and Goto , S. 2007 . Volatile Organic Compound (VOC) Analysis and Anti-VOC Measures in Water-Based Paints . J. Health Sci. , 53 : 311 – 319 .
- Yu , K.P. , Lee , G.W.M. , Huang , W.M. , Wu , C.C. , Lou , C.L. and Yang , S. 2006 . Effectiveness of Photocatalytic Filter for Removing Volatile Organic Compounds in the Heating, Ventilation, and Air Conditioning System . Journal of the Air & Waste Management Association , 56 : 666 – 674 .
- Low , J.M. , Zhang , J.S. , Plett , E.G. and Shaw , C.Y. 1998 . Effects of Airflow on Emissions of Volatile Organic Compounds from Carpet Adhesive Assemblies . ASHRAE Trans. , 104 : 1281 – 1283 .
- Lee , C.S. , Haghighat , F. and Ghaly , W.S. 2005 . A Study on VOC Source and Sink Behavior in Porous Building Materials—Analytical Model Development and Assessment . Indoor Air , 15 : 183 – 196 .
- Huang , H. and Haghighat , F. 2002 . Modeling of Volatile Organic Compounds Emission from Dry Building Materials . Build. & Environ. , 37 : 1349 – 1360 .
- Xu , Y. and Zhang , Y. 2004 . A General Model for Analyzing Single-Surface VOC Emission Characteristics from Building Materials and Its Application . Atmos. Environ. , 38 : 113 – 119 .
- Yan , W. , Zhang , Y. and Wang , X. 2009 . Simulation of VOC Emissions from Building Materials by Using the State-Space Method . Build. & Environ. , 44 : 471 – 478 .
- Afshari , A. , Lundgren , B. and Ekberg , L.E. 2003 . Comparison of Three Small Chamber Test Methods for the Measurement of VOC Emission Rates from Paint . Indoor Air , 13 : 156 – 165 .
- Guo , H. , Murray , F. , Lee , S.C. and Wilkinson , S. 2004 . Evaluation of Emissions of Total Volatile Organic Compounds from Carpets in an Environmental Chamber . Build. & Environ. , 39 : 179 – 187 .
- Sanongraj , W. , Chen , Y. , Crittenden , J.C. , Destaillats , H. , Hand , D.W. , Perram , D.L. and Taylor , R. 2007 . Mathematical Model for Photocatalytic Destruction of Organic Contaminants in Air . Journal of the Air & Waste Management Association , 57 : 1112 – 1122 . doi: 10.3155/1047-3289.57.9.1112
- Deng , B. and Kim , C.N. 2004 . An Analytical Model for VOCs Emission from Dry Building Materials . Atmos. Environ. , 38 : 1173 – 1180 .
- Li , F. and Niu , J. 2007 . Control of Volatile Organic Compounds Indoors—Development of an Integrated Mass-Transfer-Based Model and Its Application . Atmos. Environ. , 41 : 2344 – 2354 .
- Hu , H.P. , Zhang , Y.P. , Wang , X.K. and Little , J.C. 2007 . An Analytical Mass Transfer Model for Predicting VOC Emissions from Multi-Layered Building Materials with Convective Surfaces on Both Sides . Int. J. Heat & Mass Trans. , 50 : 2069 – 2077 .
- Xiong , J. , Chen , W. , Smith , J.F. , Zhang , Y. and Zhang , J. 2009 . An Improved Extraction Method to Determine the Initial Emittable Concentration and the Partition Coefficient of VOCs in Dry Building Materials . Atmos. Environ. , 43 : 4102 – 4107 .
- Qian , K. , Zhang , Y. , Little , J.C. and Wang , X. 2007 . Dimensionless Correlations to Predict VOC Emissions from Dry Building Materials . Atmos. Environ. , 41 : 352 – 359 .
- Yang , X. , Chen , Q. , Zeng , J. , Zhang , J.S. and Shaw , C.Y. 2001 . A Mass Transfer Model for Simulating Volatile Organic Compound Emissions from Wet Coating Materials Applied to Absorptive Substrates . Int. J. Heat & Mass Trans. , 44 : 1803 – 1815 .
- Altinkaya , S.A. 2009 . Predicting Emission Characteristics of Volatile Organic Compounds from Wet Surface Coatings . Chem. Eng. J. , 155 : 586 – 593 .
- Haghighat , F. and Zhang , Y. 1999 . Modeling of Emission of Volatile Organic Compounds from Building Materials—Estimation of Gas-Phase Mass Transfer Coefficient . Build. & Environ. , 23 : 377 – 389 .
- Lee , S.C. , Kwok , N.H. , Guo , H. and Hung , W.T. 2003 . The Effect of Wet Film Thickness on VOC Emissions from a Finishing Varnish . Sci. Total Environ. , 302 : 75 – 84 .
- Zhang , L.Z. and Niu , J.L. 2002 . Mass Transfer of Volatile Organic Compounds from Painting Material in a Standard Field and Laboratory Emission Cell . Int. J. Heat & Mass Transfer. , 46 : 2415 – 2423 .
- Xiong , J. , Zhang , Y. , Wang , X. and Chang , D. 2008 . Macro-Meso Two-Scale Model for Predicting the VOC Diffusion Coefficients and Emission Characteristics of Porous Building Materials . Atmos. Environ. , 42 : 5278 – 5290 .
- Li , F. , Niu , J. and Zhang , L. 2006 . A Physically-Based Model for Prediction of VOCs Emissions from Paint Applied to an Absorptive Substrate . Build. & Environ. , 41 : 1317 – 1325 .
- Yang , X. , Chen , Q. , Zhang , J.S. , Magee , R. , Zeng , J. and Shaw , C.Y. 2001 . Numerical Simulation of VOC Emissions from Dry Materials . Build. & Environ. , 36 : 1099 – 1107 .
- Brun , A. , Dihang , H. and Brunel , L. 2008 . Film Formation of Coatings Studied by Diffusing-Wave Spectroscopy . Prog. Organic Coat. , 61 : 181 – 191 .
- Bodalal , A. , Zhang , J.S. and Plett , E.G. 2000 . A Method for Measuring Internal Diffusion and Equilibrium Partition Coefficients of Volatile Organic Compounds for Building Materials . Build. & Environ. , 35 : 101 – 110 .
- Xu , Y. and Zhang , Y. 2003 . An Improved Mass Transfer-Based Model for Analyzing VOC Emissions from Building Materials . Atmos. Environ. , 37 : 2497 – 2505 .
- Wang , X. , Zhang , Y. and Xiong , J. 2008 . Correlation between the Solid/Air Partition Coefficient and Liquid Molar Volume for VOCs in Building Materials . Atmos. Environ. , 42 : 7768 – 7774 .
- Yuan , H. , Little , J.C. , Marand , E. and Liu , Z. 2007 . Using Fugacity to Predict Volatile Emissions from Layered Materials with a Clay/Polymer Diffusion Barrier . Atmos. Environ. , 41 : 9300 – 9308 .
- Zhang , L.Z. and Niu , J.L. 2003 . Effects of Substrate Parameters on the Emissions of Volatile Organic Compounds from Wet Coating Materials . Build. & Environ. , 38 : 939 – 946 .
- Sherwood , T.K. , Pigford , R.L. and Wilke , C.R. 1995 . Mass Transfer , New York : McGraw-Hill .
- Deng , Q. , Yang , X. and Zhang , J. 2009 . Study on a New Correlation between Diffusion Coefficient and Temperature in Porous Building Materials . Atmos. Environ. , 43 : 2080 – 2083 .
- Zhang , L.Z. and Niu , J.L. 2004 . Modeling VOCs Emissions in a Room with a Single-Zone Multi-Component Multi-Layer Technique . Build. & Environ. , 39 : 523 – 531 .
- White , F.M. 1988 . Heat and Mass Transfer , Reading , MA : Addison-Wesley .
- Liu , L. , Gao , S. , Shi , P. , Li , X. and Dong , Z. 2003 . Wind Tunnel Measurements of Adobe Abrasion by Blown Sand: Profile Characteristics in Relation to Wind Velocity and Sand Fiux . J. Arid Environ. , 53 : 351 – 363 .
- Bird , R.B. , Stewart , W.E. and Lightfoot , E.N. 1980 . Transport Phenomena , New York : John Wiley and Sons .