ABSTRACT
To evaluate the effectiveness of various means to combat the negative health effects of ultrafine particles emitted by internal combustion engines, a reliable, low-cost instrument for dynamic measurements of the exhaust emissions of ultrafine particulate matter (PM) is needed. In this study, an ordinary ionization-type building smoke detector was modified to serve as a measuring ionization chamber and utilized for dynamic measurements of PM emissions from diesel engines. When used with diluted exhaust, the readings show an excellent correlation with total particulate length. The instrument worked well with raw and diluted exhaust and with varying emission levels and is well suitable for on-board use.
The findings suggest the possibility for dynamic PM length measurements in diesel engine exhaust using a simple instrument using an ionization chamber analogous to the ionization chambers used in household smoke detectors, which are mass-produced and readily available on the market at costs in the $10–50 (U.S.) range. Such an instrument could be used on-board or in a test facility for qualitative online measurements and for discrimination between pre-diesel particulate filter (DPF) and post-DPF levels and identification of high-emitting vehicles, becoming a tool analogous to the garage- and inspection-grade opacity meters and exhaust gas analyzers.
INTRODUCTION
Motor vehicle emissions are one of the principal sources of air pollution in most metropolitan areas. Along with regulated pollutants (hydrocarbons [HCs], carbon mon-oxide [CO], nitrogen oxides [NOx], and total mass of particulate matter [PM]), some nonregulated components and nonregulated properties of PM are becoming a concern.Citation1–3 Particles emitted by internal combustion engines are emitted in the immediate vicinity of large concentrations of people, are generally very small,Citation4,Citation5 and readily penetrate into the lungs, where a relatively large fraction of small particles is depositedCitation6 and with ultrafine particles readily penetrating into the bloodstream.Citation7 Proximity to sources of internal combustion engine exhaust has been associated with increased risks of various chronic health problems.Citation8–10
To curb the negative effects of vehicular emissions, stricter emission standards for new engines have been adopted, with yet more standards planned. Historically, this has been the most effective approach, whereas attempts to reduce the vehicle usage have been far less successful.Citation11 Other measures adopted primarily locally include periodic emissions inspections,Citation12 use of alternative fuels, voluntary engine retrofits, low-emissions zones, and various travel demand reduction programs.Citation13 To follow sound economic principles, the effects of each of the adopted or proposed reduction strategies need to be evaluated. However, this is becoming increasingly difficult.
New engines often rely on advanced technologies, sensors and control systems, and aftertreatment devices that are often combined into highly optimized and relatively complex systems. In such systems, a departure from the optimal conditions often results in a substantial reduction of the emission benefits achieved. For example, the efficiency of catalytic devices might be reduced during prolonged low-load operation or during fuel-rich operation associated with some highly transient operation. This has been already observed with gasoline engines with a closed-loop air-to-fuel ratio control and three-way catalysts. In many cases in the past, a substantial portion of total emissions was generated by a disproportionately small fraction of the total vehicle fleet.Citation14–16 Also, a substantial portion of total emissions during real-world operation of vehicle fleets can be produced within a disproportionately short segment of the total travel time,Citation17–20 often during regimes for which the engine control has not been optimized with respect to exhaust emissions.Citation20 Additional challenges and unknowns are being introduced by new technologies and new fuels.
To properly quantify the emissions from a modern vehicle fleet under real-world operation, aggregate data on a relatively large number of vehicles (to capture the variance among individual vehicles) over a relatively long time (to capture high-emissions episodes) are necessary. Such quantification is needed to evaluate the effects of various means of reducing emissions, such as increasingly strict standards for new vehicles, changes in fuels and lubricants, retrofits, low-emission zones, traffic calming and diversions, or “eco-driving” programs. Obtaining these data in traditional laboratory settings might be extremely time- and resource-consuming, notably for heavy vehicles and off-road and nonroad applications. Also, many regions with high growth of vehicular traffic intensity lack properly equipped laboratories.
As a supplement or as an alternative to traditional laboratory testing, portable on-board systems have been developed that can be mounted on a tested vehicle and can measure its emissions during operation on a test track or during regular operation.Citation21–29
Other test methods include determination of the emissions of individual passing vehicles by remote sensing or by mobile chase laboratories or determination of overall emissions of the vehicular fleet by sampling of ambient air using mobile laboratories at intersections, from tunnels, and other suitable locations.Citation30,Citation31
The focus of this work is simple, low-cost, portable, on-board systems that can be used on a wide variety of vehicles for characterization of new technologies and fuels; for engine control system tuning; or for evaluation of improvements in traffic patterns, routes, or driving style. Such systems often use a garage-grade four- or five-gas analyzer or other multigas analyzer suitable for field work to measure concentrations of the pollutants of interest and to determine exhaust mass flow by direct measurementCitation22–24 or by inference from intake air mass flow, which is taken from the engine control unit data, measured,Citation29 or computed using speed-density method.Citation19
For particulate measurement, the traditional low-cost instrument of choice was an opacity meter. However, this meter was found to no longer be sufficient for modern, low-emitting engines and for many biofuels in which the primary measured compound, elementary carbon, no longer accounted for the bulk of the total particulate mass. Some improvements have been realized with a light-scattering instrument,Citation25,Citation32 which had a good dynamic response, but it was generally found to be less sensitive for particles smaller than approximately 200–300 nm, with some improvements gained by sampling raw, undiluted exhaust.
In this work, another low-cost instrument, a measuring ionization chamber obtained from a commercially available building smoke and fire detector, has been evaluated for its potential to serve as a portable instrument for dynamic measurements of ultrafine particles.
The smoke detector ionization chamber utilizes a radioactive source, typically 241Am, to ionize the air within the chamber between two electrodes, across which voltage is applied. Positive and negative ions are then attracted to the respective electrode, creating a small ionization current on the order of 10−10 A. Particles present in the ionization chamber between the electrodes absorb some of the ions, reducing the ionization current. A low-cost method to measure the ionization current is to introduce a third electrode placed in between the two electrodes such that particle-free air is on one side of the electrode, whereas particle-laden air is on the other side. The voltage on the third electrode is then a function of the chamber geometry, applied voltage, flow through the chamber, particle size and concentration, and various other factors.
In addition to their traditional use as smoke detectors, measuring ionization chambers have been used in the past primarily for ambient air quality measurements.Citation33
The response of an ionization chamber is proportional to the number mean particle diameter and number concentrationCitation34–36; therefore, it is proportional to the bx-total particulate length, determined as the sum of the diameters of the individual particles. Ion chambers were reported to have a relatively high response to diesel PMCitation37 but had a very low response to 0.8- to 10μm dust particles.Citation38
The goal of this study was to use a measuring ionization chamber for measurements of PM in diesel exhaust. The immediate application was to characterize the transient emission behavior of an engine powered by vegetable oil; however, the work was carried on with the vision of such instruments being used in portable on-board systems.
EXPERIMENTAL PROCEDURES
Two samples of a household smoke detector with an ionization chamber containing the 241Am isotope (intensity of 35 kBq) were procured from a local manufacturer (ICAS, Praha, Czech Republic). The outer cover was removed, and the electrical leads from the circuit board to the ionization chamber were disconnected. The “outer” two electrodes (“shell” and “base” of the chamber) were connected to a regulated power supply, and the “intermediate” electrode was connected to a voltage-sensing integrated circuit. Apart from this modification, the circuit board with the ionization chamber was left intact and was secured in a 150-mm section of a 75-mm (3-in.) nominal diameter thick-wall polyvinyl chloride sewer pipe that was capped at both ends. The inlet, a section of 6-mm (1¼-in.) diameter copper tubing, was lead through one of the caps directly into an opening in the ionization chamber cover. Outside air was allowed to penetrate through other narrow openings into the enclosure and ionization chamber to serve as a sheath and dilution air. The outside air was not filtered or conditioned in any way. The outlet was coupled to a 15-L/min air pump that was preceded by a filter to remove the particles. The setup of the instrument is shown in
The instrument was used to sample exhaust from a 4.2-L, 90-kW (120-hp) Zetor 1505 (Zetor, Brno, Czech Republic) four-cylinder turbocharged tractor diesel engine with a mechanically controlled Motorpal inline fuel injection pump. The engine was certified to 0.3 g/kWh (0.22 g/bhp-hr) PM emissions (International Standardization Organization [ISO]-8178 test for nonroad engines). The engine was operated mostly in steady state in regimes defined in the ISO-8178 test for nonroad engines in “ordinary” (C-1) and low-load (C-2) schedules that were supplemented by several low-load points.
To evaluate the effects of varying fuel composition, the engine was operated on European highway diesel fuel and on nonesterified fuel-grade (German norm DIN V 51605) rapeseed oil (FabioProdukt, Holín, Czech Republic), the combustion and emissions characteristics of which are rather dissimilar from diesel fuel. The engine was fitted with a dual-fuel system allowing operation on diesel fuel or vegetable oil, with vegetable oil being heated by the engine coolant to 60–90 °C at the fuel injection pump inlet. Given the strong effects of the engine operating history on emissions during operation on vegetable oil,Citation29 the same test series sequence, including preconditioning, was always followed for both fuels. Two test series were run, with the instrument sampling points and overall test setup shown in
In one test series (test A), the exhaust was sampled from the laboratory exhaust duct near the tested engine with a dilution ratio of approximately 3:1 at full load. During this test series, a partial-flow dilution tunnel developed in-house was used for gravimetric PM measurements on 47-mm PallFlex T60A20 filters. Separate pairs of filters were used for each operating point of the engine. To clarify the results, this test series was later repeated with the size distribution spectra of the PM measured by a scanning mobility particulate sizer (SMPS) spectrometer coupled with a condensation particle counter (CPC; both from TSI, Inc.), which sampled the exhaust from the laboratory main exhaust duct, acting as an improvised full-flow dilution tunnel. Simultaneous measurements of concentrations of NOx and carbon dioxide (CO2) in the raw exhaust and in the main exhaust duct have demonstrated that the duct operates at a nearly constant flow of approximately 8000 m3/hr, corresponding to a dilution ratio of approximately 13:1 at full load and approximately 80:1 at idle.
During a second test series (test B), the experimental ionization chamber sampled the exhaust from the main exhaust duct through a 6-mm internal diameter pipe oriented parallel with the direction of the flow. At the same location, comparative particulate size distribution measurements in the range of 5–560 nm were performed by an engine exhaust particulate sizer (EEPS) spectrometer (model 3090, TSI, Inc.). This test series was also repeated with the engine fitted with a passive diesel particulate filter (DPF; Huss Umwelttechnik) with regeneration by a fuel-born catalyst (Ferrocene, Inno-spec, Inc., dosed at 50 parts per million iron by weight).
For each operating point in test series A, the ionization chamber analog output signal was averaged over a 150-sec period ending 30 sec before the end of the regime.For the test series B, the comparison was done on a second-by-second basis. The ionization chamber signal was subtracted from a base signal (“zero”) obtained by sampling laboratory air before and after the test series. For all tests, total particulate length was calculated from PM size distributions measured by a SMPS and an EEPS as a sum of the products of the mean particle diameter and PM concentration in each size bin. To facilitate comparison with the reference measurement (total PM mass or total PM length), the signal, measured in volts, was multiplied by an arbitrary constant of 7.1 for PM mass and 2.2 for PM length, which was chosen to yield comparable numerical values during the ISO-8178 C-1 test. These constants were then applied to all ionization chamber measurements for diesel fuel and heated rapeseed oil and for operation with and without a DPF. Because the EEPS and ionization chamber are capable of dynamic measurement, the comparison was made over the entire test run, including the transitions between the regimes. To avoid implications as to what property is measured by the ionization chamber, the adjusted ionization chamber signal is reported in relative units.
To evaluate the transient response, the ionization chamber measurements of raw, undiluted exhaust were evaluated for the engine transient cycle (ETC) run on a Euro 3 Iveco Tector diesel engine on an engine dynamometer, and after multiplying by intake airflow, a surrogate for exhaust flow, they were compared with concentrations in 100-nm size bins measured simultaneously by SMPS and CPC sampling from a dilution tunnel. To evaluate on-road performance, the ionization chamber signal was compared with a laser light-scattering instrument,Citation20 both sampling raw, undiluted exhaust from a 2007 Renault traffic light-duty van with a Euro 4 turbodiesel engine while operating on suburban roads. The transient response evaluation was only qualitative.
RESULTS
The comparison of the ionization chamber signal (in relative units) with the gravimetric measurement (in grams per hour) for diesel fuel runs in test A is given in For most regimes, the results show a good correlation between the two measurements. During all four regimes at zero load—two regimes of idle at nominal idle speed of 780 revolutions per minute (rpm) and two points of “idle” at an arbitrarily selected speed of 1240 rpm—the ionization chamber response was markedly higher than the gravimetric measurement. During one point marked on as “saturation,” condensation was observed in the ionization chamber outlet line.
Figure 3. Comparison of PM emissions measured by the ionization chamber (in arbitrary units) with gravimetric measurement at steady-state engine operation on diesel fuel at various speeds and loads.
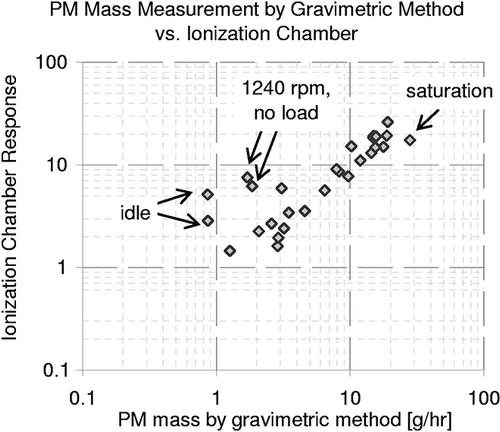
The different response of the ionization chamber during no-load operation has been correlated to markedly different particle size distribution during no-load compared with loaded operation, with no-load operation dominated by nanoparticles (peak number concentrations ∼20 nm) and loaded operation by accumulation-mode particles (peak number concentrations ∼100 nm). This is illustrated in , which shows particle size distribution spectra obtained by SMPS and CPC during idle and during four loaded regimes.
Figure 4. PM size distribution measured by SMPS and CPC during operation on diesel fuel at idle and at various loads.
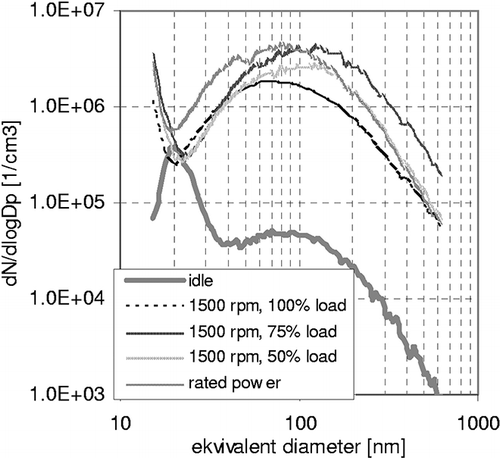
The comparison between the ionization chamber response and the total particulate length computed from the measurements by SMPS and CPC is given in and shows nearly a linear correlation with the exception of idle. It should be noted that these measurements were not done simultaneously but during two separate runs of the same test series, and that the two instruments were not sampling from the same location. The measurement uncertainties of both instruments were small (up to units of percent).
Figure 5. Comparison of total PM length measured by SMPS and CPC during steady-state engine operation on diesel fuel at various speeds and loads with the ionization chamber response.
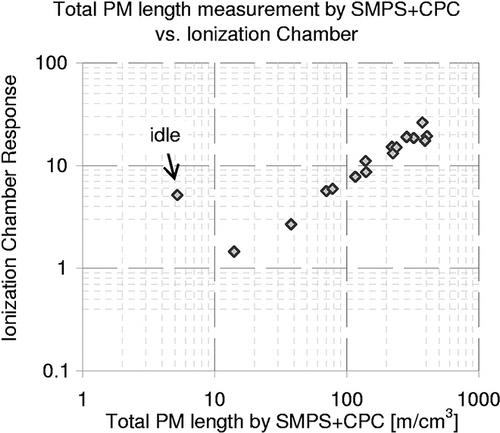
Comparison of ionization chamber and gravimetric measurements for diesel fuel and vegetable oil runs is given in and shows similar ionization chamber response for both fuels. The operating points where the ionization chamber response is higher generally correspond to the particle size distribution shifted, relative to diesel fuel operation, toward smaller particles.
Figure 6. Comparison of PM emissions measured by the ionization chamber (in arbitrary units) with gravimetric measurement at steady-state engine operation on diesel fuel and on heated fuel-grade rapeseed oil at various speeds and loads.
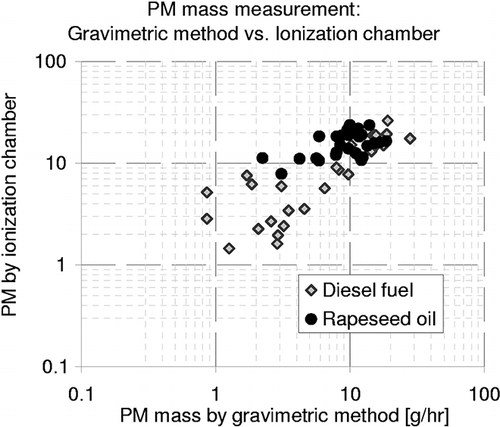
For the test series B, the engine rpm, torque, and total PM length are plotted in for operation on diesel fuel without DPF, on diesel fuel with DPF, on heated rapeseed oil without DPF, and on heated rapeseed oil with DPF. The same test sequence, without DPF, was also used in test series A.
Figure 7. Comparison of total PM length measured by EEPS with the ionization chamber response during a sequence of steady-state operating points run on diesel fuel and heated fuel-grade rapeseed oil with and without a DPF.
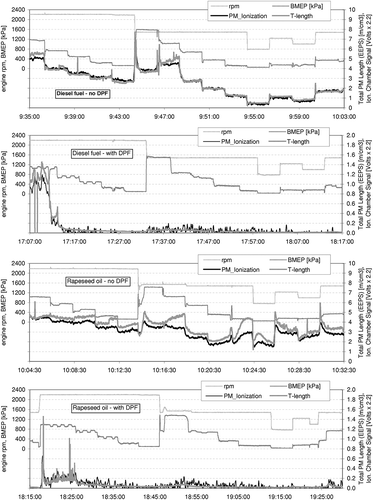
The graphs in and a scatterplot of second-by-second data in show an excellent correlation between the ionization chamber response and the total PM length measured by EEPS for operation on diesel fuel without the DPF. This correlation also holds for the operation on diesel fuel with the DPF, although the ionization chamber readings are within the noise level except for the beginning of the test run during and after the regeneration. (Note that the PM measurement y-axis scale range for operation with DPF is one fifth of the scale range on the graph showing results without DPF.)
Figure 8. Comparison of PM emissions measured by the ionization chamber (in arbitrary units) with total PM length calculated from EEPS measurements during operation on diesel fuel without and with a DPF.
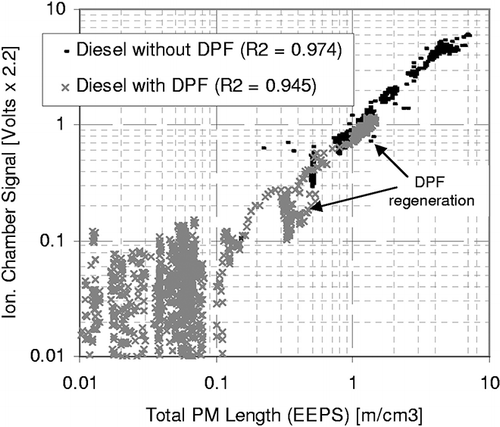
The correlation between the two measurements for rapeseed oil tests without the DPF, shown in , is worse than that for diesel fuel but is still relatively good. The correlation was not dramatically improved by shifting the base (zero) value of the ionization detector, by assuming logarithmic or power relationship, or by correlating to the combination of PM length and PM surface area. The correlation for rapeseed oil tests with DPF is reasonably good, with the ionization chamber readings being below the noise level during most of the test run. The Pearson correlation coefficients between the ionization chamber readings and total particle number, length, surface area, and volume measured by the EEPS during each second of the measurement (n = 1680) are shown in . The correlation of all EEPS and ionization data for both fuels, with and without DPF, is shown in
Table 1. Correlation of the second-by-second signal of the ionization chamber signal with EEPS measurements— operation without DPF
Figure 9. Comparison of PM emissions measured by the ionization chamber (in arbitrary units) with total PM length calculated from EEPS measurements during operation on heated rapeseed oil without and with a DPF.
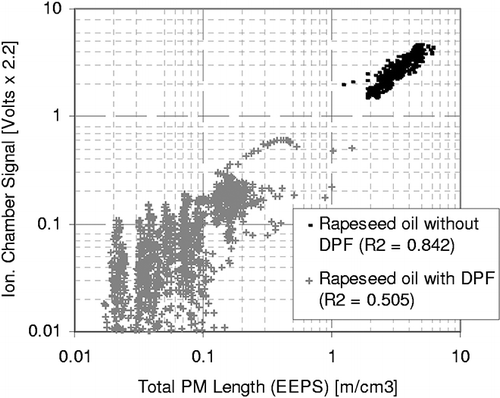
Figure 10. Comparison of PM emissions measured by the ionization chamber (in arbitrary units) with total PM length calculated from EEPS measurements—all tests.
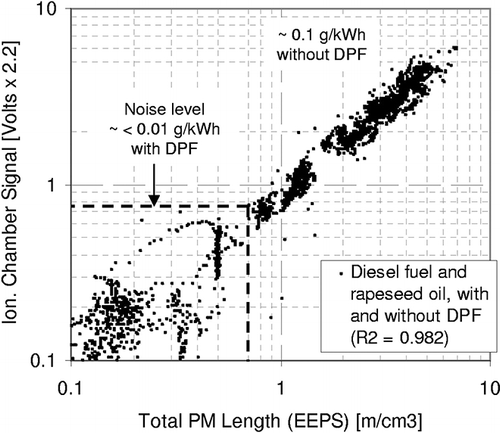
The ionization chamber signal measured during the ETC cycle, synchronized in time with and multiplied by intake airflow signal provided by the laboratory, is plotted against PM concentrations in the 100-nm size bin measured by SMPS and CPC in The ionization chamber signal measured during operation of the Renault van is compared with the light-scattering instrument signal in
DISCUSSION
The tests show remarkably good correlation between the rather crude ionization detector and total PM length. The correlation to the PM mass was good for regimes with comparable particle size distribution and deteriorated when the PM size distribution shifted, which is expected because the correlation between PM length and PM mass depends on the PM size distribution.
The ionization chamber operated (at higher dilution ratio) in the noise level during most of the operation with DPF, except for DPF regeneration. This is not necessarily a detriment because PM emissions are known to be very low during such operation, and the ionization chamber correctly followed any episodes with higher emissions. On the other side of the spectra, the only point identified as being above the ionization chamber range was when the detector was sampling exhaust at a relatively low dilution ratio during a relatively high PM regime (∼0.5 g/kWh), which might be acceptable for off-road and nonroad engines but can be considered excessive for modern highway engines. Therefore, the measurement range of the ionization chamber starts at levels that can be considered as acceptably low and not of concern and extends to levels beyond which the PM emissions levels can be considered excessive. Some adjustment of the scale is possible by the choice of the dilution ratio.
To a large extent, the correlation also held for operation on rapeseed oil, which is associated with much higher fractions of organic carbon and correspondingly lower fractions of black soot than diesel exhaust, showing that the ionization chamber is not overly selective to the fuel.
The comparison between the ionization chamber response and total PM length during operation on rapeseed oil without DPF, shown in , demonstrates that the discrepancies are always on the side of the ionization chamber reading being lower. One possible explanation for this is volatile particle losses in the ionization chamber or in the sampling system, neither of which was heated. Likewise, when the ionization chamber was sampling near the engine, at lower dilution ratios, the reading at idle (the only outlier in the comparison with PM length measured by SMPS and CPC; see ) might have been higher because of a nucleation event in an already cold exhaust in the unheated sampling train. It is likely that the measurement could be improved by following the proper aerosol handling techniques, something that has not been done because of budget restraints and because the instrument was intended for rather crude operation on-board a vehicle or in an improvised laboratory. The transient response of the ionization chamber, shown in and , appears to be qualitatively acceptable.
These findings suggest a possibility for dynamic PM length measurements in diesel engine exhaust using a simple, low-cost instrument—ionization smoke alarms for household use are mass-produced and are readily available on the market at costs in the $10–50 (U.S.) range. Therefore, an instrument using an ionization chamber, perhaps more optimized for vehicle emission monitoring than the household smoke detectors and capable of qualitative online measurements and discrimination between pre-DPF and post-DPF levels and identification of high-emitting vehicles, can probably be made available at a cost on the same order of magnitude as the currently used garage- and inspection-grade opacity meters and five-gas analyzers.
Although the ionization chamber uses a radioactive source, thus raising a wide range of concerns as to its handling, transportation, and disposal, the intensity of the source is relatively small. Similar ionization chambers used in household smoke detectors are readily available to the general public and are present throughout buildings at a quantity by far exceeding the anticipated quantities of instruments used for vehicular exhaust measurements.
Whether total PM length is the most desirable quantity to be measured remains to be determined and is likely to be a subject for future discussion in light of the ongoing and future research on the health effects of diesel PM. It can be argued that the total PM length is a quantity that is more representative of health effects than the currently used total PM mass. Also, on the basis of theory and the results obtained with a rather crude sample handling system, it can be argued that the measurement of total PM length is less sensitive to aerosol nucleation events and thus to aerosol handling procedures than the total PM number. Finally, it can be argued that the PM length measurements, in combination with existing opacity or other measurements, might provide a reasonable indication of the quantity of a wide range of PM properties.
CONCLUSIONS
In this study, an experimental instrument using the ionization chamber from an ordinary household smoke detector was used to measure the concentrations of PM in diesel engine exhaust during its operation at a range of speeds and loads on diesel fuel and rapeseed oil, with and without a DPF. The readings correlated remarkably well with total particle length measured by SMPS and CPC and by EEPS during operation without DPF as well as DPF regeneration events, whereas the readings during the rest of the DPF operation, in which the PM emissions were very low, were within the noise. The results suggest a potential for the possibility to be entertained that a small, low-cost instrument using an ionization chamber can be used for at least indicative dynamic real-time PM measurements for research purposes and as an inspection and maintenance tool for engines using a DPF and latest-generation engines compliant with U.S. Environmental Protection Agency 2007 and 2010 and Euro 5 standards for on-road engines.
ACKNOWLEDGMENTS
The experimental work on the Zetor engine was funded by Czech Science Foundation project number 101/08/ 1717 (“Optimization of combustion of vegetable oils in diesel engines”); the experimental instrument described here was originally developed and later used for tracking drifts in particulate emissions and transient response in engines powered by vegetable oils. On-road measurements and comparison testing on the transient cycle were carried on within the Ministry of Education of the Czech Republic project no. 1M6840770002 (“Josef Božek Research Centre for Engine and Vehicle Technologies II”) and the Czech Ministry of Transport project no. CG912-058-520 (“Methodology of quantification and assessment of the environmental and safety impacts of transport”). The authors thank Jiří Pochman from ICAS, Prague, Czech Republic, for providing the detectors; Otokar Vojtisek, VMK, Prague, Czech Republic, for extensive and helpful information on detector performance; and Richard Vacek from TUV-SUD Auto, Prague, Czech Republic, for SMPS measurements.
REFERENCES
- Maricq , M.M. 2007 . Chemical Characterization of Particulate Emissions from Diesel Engines: A Review . Aerosol Sci. , 38 : 1079 – 1118 .
- Hu , S. , Herner , J.D. , Shafer , M. , Roberston , W. , Schauer , J.J. , Dwyer , H. , Collins , J. , Huai , T. and Ayala , A. 2009 . Metals Emitted from Heavy-Duty Diesel Vehicles Equipped with Advanced PM and NOx Emission Controls . Atmos. Environ. , 43 : 2950 – 2959 .
- Giechaskiel , B. , Alföldy , B. and Drossinos , Y. 2009 . A Metric for Health Effects Studies of Diesel Exhaust Particles . Aerosol Sci. , 40 : 639 – 651 .
- Kittelson , D.B. 1998 . Engines and Nanoparticles: A Review . J. Aerosol Sci. , 29 : 575 – 588 .
- Kittelson , D.B. , Watts , W.F. and Johnson , J.P. 2006 . On-Road and Laboratory Evaluation of Combustion Aerosols Part 1: Summary of Diesel Engine Results . J. Aerosol Sci. , 37 : 913 – 930 .
- Gehr , P. , Blank , F. and Rothen-Rutishauser , B. 2006 . Fate of Inhaled Particles after Interaction with the Lung Surface . Paediatr. Respir. Rev. , 7 : S73 – S75 . Suppl. 1
- Peters, A. Epidemiology on Health Effects of Solid Nanoparticles . Proceedings of the 12th Swiss Federal Institute of Technology Zurich Conference on Combustion Generated Nanoparticles . Zurich , Switzerland. June .
- Lewtas , J. 2007 . Air Pollution Combustion Emissions: Characterization of Causative Agents and Mechanisms Associated with Cancer, Reproductive, and Cardiovascular Effects . Mutat. Res. , 636 : 95 – 133 .
- McEntee , J.C. and Ogneva-Himmelberger , Y. 2008 . Diesel Particulate Matter, Lung Cancer, and Asthma Incidences along Major Traffic Corridors in MA, USA: A GIS Analysis . Health & Place , 14 : 817 – 828 .
- Balmes , J.R. , Earnest , G. , Katz , P.P. , Yelin , E.H. , Eisner , M.D. , Chen , H. , Trupin , L. , Lurmann , F. and Blanc , P.D. 2009 . Exposure to Traffic: Lung Function and Health Status in Adults with Asthma . J. Allergy Clin. Immunol. , 123 : 626 – 631 .
- Rajan , S.C. 1996 . The Enigma of Automobility: Democratic Politics and Pollution , Pittsburgh , PA : University of Pittsburgh .
- Denis , M.S. and Lindner , J. 2005 . Review of Light-Duty Diesel and Heavy-Duty Diesel Gasoline Inspection Programs . Journal of the Air & Waste Management Association , 12 : 1876 – 1884 .
- Santos , G. , Behrendt , H. , Maconi , L. , Shirvani , T. and Teytelboym , A. 2010 . Part I: Externalities and Economic Policies in Road Transport . Res. Transport. Econ. , 28 : 2 – 45 .
- Bishop , G. and Stedman , D. 1990 . On-Road Carbon Monoxide Emission Measurement Comparisons for the 1988–1989 Colorado Oxy-Fuels Program . Environ. Sci. Technol. , 24 : 843 – 847 .
- Guenther , P.L. , Bishop , G.A. , Peterson , J.E. and Stedman , D.H. 1994 . Emissions from 200 000 Vehicles: A Remote Sensing Study . Sci. Total Environ. , 146 : 297 – 302 .
- Ross , M. 1994 . Automobile Fuel Consumption and Emissions—Effects of Vehicle and Driving Characteristics . Ann. Rev. Energy Environ. , 19 : 75 – 112 .
- Kelly , N.A. and Groblicki , P.J. 1993 . Real-World Emissions from a Modern Production Vehicle Driven in Los Angeles . Journal of the Air & Waste Management Association , 43 : 1351 – 1357 .
- St. Denis , M.J. , Cicero-Fernandez , P. and Winer , A.M. 1994 . Effects of In-Use Driving Conditions and Vehicle/Engine Operating Parameters on “Off-Cycle” Events: Comparison with FTP Conditions . Journal of the Air & Waste Management Association , 44 : 31 – 38 .
- Vojtíšek-Lom , M. and Cobb , J.T. On-Road Light-Duty Vehicle Emission Measurements Using a Novel Inexpensive On-Board Portable System . Presented at the 8th Coordinating Research Council On-Road Vehicle Emissions Workshop . San Diego , CA .
- Vojtíšek-Lom , M. , Fenkl , M. , Dufek , M. and Mareš , J. Off-Cycle, Real-World Emissions of Modern Light-Duty Diesel Vehicles . Society of Automotive Engineers (SAE) Technical Paper 2009-24-0148 . 2009 . SAE: Warrendale, PA
- Lenaers , G. , Pelkmans , L. and Debal , P. 2003 . The Realisation of an On-Board Emission Measuring System Serving as a R&D Tool for Ultra Low Emitting Vehicles . Int. J. Veh. Design , 31 : 253 – 268 .
- Dearth , M.A. , Butler , J.W. , Colvin , A. , Gierczak , C. , Kaberline , S. , Korniski , T. and Semtech , D. The Chassis Roll Evaluation of a Commercial Portable Emission Measurement System (PEMS) . Society of Automotive Engineers (SAE) Technical Paper 2005-01-0673 . 2005 . SAE: Warrendale, PA
- Breton , L. Real-Time On-Road Vehicle Exhaust Gas Modular Flowmeter and Emissions Reporting System . U.S. Patent 6148656 . 2000 .
- Nakamura , H. , Kihara , N. , Adachi , M. and Ishida , K. Development of a Wet-Based NDIR and Its Application to On-Board Emission Measurement System . Society of Automotive Engineers (SAE) Technical Paper 2002-01-0612 . 2002 . SAE: Warrendale, PA
- Vojtíšek-Lom , M. and Allsop , J.E. Development of Heavy-Duty Diesel Portable, On-Board Mass Exhaust Emissions Monitoring System with NOx, CO2 and Qualitative PM Capabilities . Society of Automotive Engineers (SAE) Technical Paper 2001-01-3641 . 2001 . SAE: Warrendale, PA
- Vojtíšek-Lom , M. , Wilson , P.J. and Julian , M. Enhanced Real-Time, Real-World Vehicle Emissions Measurement Using an On-Board FTIR Analyzer . Presented at the 14th Coordinating Research Council On-Road Vehicle Emissions Workshop . San Diego , CA .
- Daham , B. , Andrews , G.E. , Li , H. , Rosario , B. , Bell , M. , Tate , J. and Ropkins , K. Application of a Portable FTIR for Measuring On-Road Emissions . Society of Automotive Engineers (SAE) Technical Paper 2005-01-0676 . 2005 . SAE: Warrendale, PA
- Collins , J. , Sheperd , T. , Durbin , T. , Lents , J. , Norbeck , J.N. and Barth , M. 2007 . Measurements of In-Use Emissions from Modern Vehicles Using an On-Board Measurement System . Environ. Sci. Technol. , 41 : 6554 – 6561 .
- Investigation of Diesel Emission Control Technologies on Off-Road Construction Equipment at the World Trade Center and PATH Re-Development Site; Contract 426-03-001; Report prepared by M.J. Bradley & Associates for the Port Authority of New York and New Jersey, Materials Engineering Division: New York, 2004.
- Ropkins , K. , Beebe , J. , Li , H. , Daham , B. , Tate , J. , Bell , M. and Andrews , G. 2009 . Real-World Vehicle Exhaust Emissions Monitoring: Review and Critical Discussion . Crit. Rev. Environ. Sci. Technol. , 39 : 79 – 152 .
- Smit , R. , Ntziachristos , L. and Boulter , P. 2010 . Validation of Road Vehicle and Traffic Emissions Models—A Review and Meta-Analysis . Atmos. Environ. , 44 : 2943 – 2953 .
- Moosmueller , H. , Arnott , W.P. , Rogers , C.F. , Bowen , J.L. , Gillies , J.A. , Pierson , W.R. , Collins , J.F. , Durbin , T.D. and Norbeck , J.M. 2001 . Time Resolved Characterization of Diesel Particulate Emissions. 1. Instruments for Particle Mass Measurements . Environ. Sci. Technol. , 35 : 781 – 787 .
- Edwards , R. , Smith , K.R. , Kirby , B. , Allen , T. , Litton , C.D. and Hering , S. 2006 . An Inexpensive Dual-Chamber Particle Monitor: Laboratory Characterization . Journal of the Air & Waste Management Association , 56 : 789 – 799 .
- Litton , C.D. 2002 . The Use of Light Scattering and Ion Chamber Responses for the Detection of Fires in Diesel Contaminated Atmospheres . Fire Safety J. , 37 : 409 – 425 .
- Bernigau , N.G. and Luck , H.O. 1986 . The Principle of the Ionization Chamber in Aerosol Measurement Techniques—A Review . J. Aerosol Sci. , 17 : 511 – 515 .
- Aggarwal , S. and Motevalli , V. 1997 . Investigation of an Approach to Fuel Identification for Non-Flaming Sources Using Light-Scattering and Ionization Smoke Detector Response . Fire Safety J. , 29 : 99 – 112 .
- Litton , C.D. 2002 . Studies on the Measurement of Respirable Coal Dusts and Diesel Particulate Matter . Measure. Sci. Technol. , 13 : 365 – 374 .
- Litton , C.D. 2009 . Laboratory Evaluation of Smoke Detectors for Use in Underground Mines . Fire Safety J. , 44 : 387 – 393 .