ABSTRACT
From 2004 to 2009, aiming to better understand implications for its smelters, Rio Tinto Alcan conducted a detailed study of PM2.5 and PM10 (particulate matter [PM] ≤ 2.5 and 10 μm in aerodynamic diameter, respectively) in its facilities. This involved a two-level study: part 1, emission quantification; and part 2, assessment of aluminum smelter contribution to the surrounding environment. In the first part, U.S. Environmental Protection Agency Other Test Method (OTM) OTM27 and OTM28 are assessed as relevant and efficient methods for measuring fine particle emissions from aluminum smelter stacks. Rio Tinto Alcan has also developed a safe and robust method called CYCLEX to measure PM2.5 and condensable particulate matter (CPM) at the roof vents of potrooms. This work aims to determine the PM2.5 emission coefficients of 17, 55, and 417 g·t−1 of aluminum produced (including CPM) in anode baking furnace exhaust (fume treatment center), at potroom scrubber stacks (gas treatment centers), and at potroom roof vents, respectively. Results indicate that roof vents are the primary PM2.5 emitters (85% of all smelter emissions) and that 71% of all smelter PM2.5 comes from CPM. In the second part, preliminary inorganic speciation studies are conducted by scanning electron microscopy–energy-dispersive X-ray analysis and by isotopic ratios to track smelter emissions to their surrounding environment. This paper releases the first speciation results for an aluminum smelter, and the preliminary isotopic ratio study indicates a 3% impact in terms of PM2.5 emissions for a representative smelter in an urban area.
Aluminum smelters tend to continuously improve their competitiveness by incrementally increasing production. In this context, assessing the effect of major contaminants is overriding, and ambient air modeling is often the preferred way to do so. Fine particles fit this category, and the primary aluminum industry needs to accurately know their emission factors to obtain representative modeling. Moreover, not all aluminum smelters have a method to measure PM2.5 at roof vents, the primary emission outlets. Therefore, this paper describes the first-rate PM2.5 measurement methods for aluminum smelter roof vents without down-comers. It also provides insight for environmental managers for tracking PM2.5 emissions in plant surroundings.
INTRODUCTION
Airborne particulate matter (PM) is composed of primary aerosols (emitted as such, including condensable particulate matter [CPM]) and secondary aerosols originating from reactions among gases (oxides of sulfur and nitrogen, volatile organic compounds [VOCs], and ammonia).Citation1,Citation2 There is now consensus in the international community that PM less than 2.5 μm in aerodynamic diameter (PM2.5) can have adverse effects on human health and that, unfortunately, they are positively associated with hospital admissions.Citation3–6 However, it is unclear which PM2.5 components could be responsible for such toxicity.Citation7 Nevertheless, the U.S. Environmental Protection Agency (EPA) and the European Union (EU) are implementing regulations aiming to reduce mortality-related to PM2.5 exposure.Citation8 Only a few studies currently focus on PM2.5 emissions from heavy industries such as steel plants,Citation9 foundries,Citation10 and secondary aluminum smelters,Citation11 but the primary aluminum industry has yet to be the subject of study.
The process of aluminum production involves the electrolytic reduction of alumina in a cell that is dissolved in a molten bath mainly containing cryolite. Thus, hundred-meter-long potrooms are subject to dust emissions associated with combustion gases. Raw pot gases and dust are recovered right from the cells and later efficiently scrubbed at the gas treatment center (GTC), most often with bag filters. During operations on pots (anode replacements in particular), PM that is neither recovered nor treated is emitted as the hoods are opened. These emissions escape through the roof vents. Significant dust is also emitted from anode baking furnaces, where prebake technology anodes are prepared. During this process, gas and dust are collected and treated at the fume treatment center (FTC), also with bag filters.
In the aluminum industry, total particulate matter (PMtotal =PM>0.3 μm plus condensables) and usually PM10 (PM ≤ 10 μm in aerodynamic diameter) are monitored in the ambient air around plants, and PMtotal (but without condensables) is tracked at stacks and roof vents for reporting purposes. However, condensables are never taken into account, and the extent to which they are released is completely unknown. Furthermore, the measurement methods at stacks and roof vents in the aluminum industry are not up to date and should be reviewed in the light of scientific advancements. Many years ago EPA released stack methods for fine particle measurements; for example, filterable PM10 and PM2.5 are measured at stacks with EPA method Conditional Test Method (CTM) CTM040Citation12, and CPM is estimated with EPA202.Citation13 These methods are suitable for aluminum smelters with respect to their dry emissions and the sampling of unnecessary dilutions. EPA is now proposing two new methods for fine particle measurements: Other Test Method (OTM) OTM27Citation14 and OTM28.Citation15 OTM27 could be seen as the only official method for measuring PM10 and PM2.5 at stacks, superseding CTM040. It does not present any major changes. OTM28 is the new method for condensable measurements, in lieu of EPA202 and should not overestimate CPM as EPA202 does. Nevertheless, these methods are inapplicable for vented roofs of aluminum smelter pot-rooms. For smelters equipped with downcomers (collecting raw pot gases in potrooms—see ), method EPA14 can be adapted with CTM040 and EPA202 to measure PM10, PM2.5, and CPM at roof vents. For smelters without downcomers, Rio Tinto Alcan proposes a new method called CYCLEX.
Figure 1. Illustration of an electrolysis pot with the raw pot gases recovered and treated at the GTC with alumina. Unrecovered pot gases are vented through the roof of each potroom. This is where CYCLEX, which measures PM2.5 and CPM, is located.
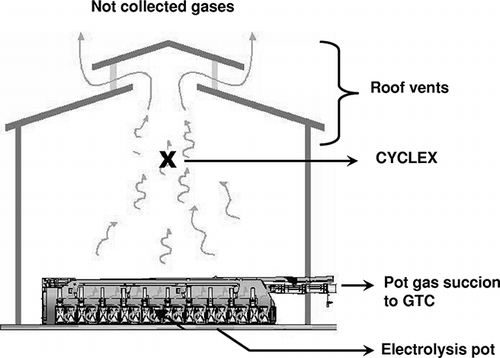
In anticipation of future PM2.5 regulations and to better understand the potential environmental footprint of the aluminum industry, Rio Tinto Alcan launched its PM2.5 program in 2004. For part 1 of the program, the objectives were (1) to identify the best PMtotal and PM2.5 measurement methods at stacks, and (2) to develop an innovative method called CYCLEX to quantify PMtotal and PM2.5 at roof vents. For part 2 of the program, the objectives were (1) to address the speciation of PM2.5 emissions from a typical smelter, and (2) to track PM emissions from the smelter to the environment. To address these questions, some state-of-the-art technologies were used, such as scanning electron microscopy (SEM) and stable and radiogenic isotope analyses.
EXPERIMENTAL PROCEDURES
Note that, in this paper, the metric system is used for measurement units. Also, all statistical tests were performed using GraphPad Prism, version 4.00 for Windows (GraphPad Software; http://www.graphpad.com).
Aluminum Smelters Studied
The two smelters studied are Sebree Works (Kentucky, United States) and Alma (Quebec, Canada), both operating prebake technologies (P-155 and AP-30, respectively) and producing 190 and 420 kt of aluminum per year, respectively. Operations are extremely stable in time and thus any time window along the year is acceptable for sampling.
Part 1: Quantification of PM2.5 Emitted by the Sebree Smelter
Part 1.1: Emissions from Stacks
To find out the PM2.5 emission factors for the Sebree Works GTC (reduction potlines) and FTC (anode baking furnace), a local contractor conducted initial trials according to EPA methods CTM040/202 in June 2006 (four and five replicates, respectively, at GTC and FTC). A new sampling campaign was run in October 2008 using EPA OTM27 and OTM28 (three replicates). The aim of this second campaign was to compare the effectiveness of wet (EPA 202) and dry impingers (OTM28). Indeed, an artifact is known to occur with high concentrations of sulfur dioxide (SO2),Citation16 the latter being prominent at GTC stacks (100–150 parts per million [ppm]).
Part 1.2: Emissions from Roof Vents
Concerning monitoring at smelter roof vents, Rio Tinto Alcan designated Sebree Works as an ideal place to test an innovative way (CYCLEX) to measure PM2.5. Compared with Canadian aluminum smelters, U.S. facilities are designed with sampling manifolds, or “downcomers,” at roof vents. These allow the smelters to measure PM emissions at roof vents with EPA M14Citation17/CTM040/202 methods (hereinafter M14*); in other words, downcomers are gas collectors in specific potroom sections merging into one main duct and finally exhausting like a regular stack. Rio Tinto Alcan can then compare its CYCLEX candidate method to this reference method in potrooms. But with no downcomers, it is impossible to use the EPA M14* method because of the absence of a stack and the strictly forbidden presence of water in potrooms (risk of explosion).
The CYCLEX method basically involves inserting a curved sampler inlet from method 5A in the APEX PM2.5 sizer (). The PM2.5 sizer may be attached to the end of a rod that can be swiveled from the catwalk. A pulley may be used where there are no cat-walks. A Teflon line then connects each modified APEX PM2.5 sizer to a sampling train with wet impingers (EPA 202; dry impingers were not tested). With this design, PM10 measurements are not possible. The setup involves one CYCLEX sampling train between two pots along an eight-pot section (four modified APEX PM2.5 sizers). The four sampling trains are centered on a gas velocity anemometer and located just above the cat-walk. depicts the location of one CYCLEX arrangement; a potroom in Sebree Works has 64 pots. Results are thus expressed taking into account (1) the average airspeed inside of the potroom, measured with anemometers (at Sebree usually ∼1.6 m·sec−1); and (2) the total open roof vent surface (at Sebree 4855 m2). CYCLEX tests were conducted side by side with the M14* method in 2007 (both 12 replicates) and 2008 (20 and 5 replicates, respectively). This candidate method is currently going through the approval process with Environment Canada and EPA Québec.
Part 2: Speciation of PM2.5 in the Surrounding Environment of the Alma Smelter
The purpose of this part is to obtain first insights into the aluminum industry's PM2.5 speciation. This study also aims to track PM2.5 emissions from raw anodes through baking (FTC emissions) and anode consumption (GTC emissions) and lastly to the ambient air by using isotopic ratios. To do so, anodes were sampled because they are one of the main sources of fine particle emissions; anodes are made of carbon and pitch. Next, the FTC and GTC stacks were sampled in triplicate at 16.7 L/min (6- and 24-hr samplings, respectively, with Hi-Vol PM10 retrofit to PM2.5); the urban monitoring stations 1A (218,421 m east; 5,381,784 m north; elevation 100 m; 2000 m from the smelter) and 3A (215,053 m east; 5,384,914 m north; elevation 110 m; 1750 m from the smelter) were the last two sites for Hi-Vol PM2.5 sampling (167 hr, including the FTC and GTC sampling periods, one replicate). These campaigns were conducted in May 2008; roof vents were not sampled because their emission signature is the same as that of the GTC. Prevailing winds and locations are shown in . Each collected filter (FTC, GTC, 1A, and 3A) was analyzed in four ways. First, one quarter of the cellulosic filters was analyzed by SEM–energy-dispersive X-ray (SEMEDX) analysis under low vacuum and uncoated (Arvida Research & Development Centre [ARDC] metallographic laboratory). Second, another quarter of the filter was acid digested for inductively coupled plasma (ICP)–atomic emission spectroscopy (AES) analysis, and a third quarter was caustic digested for fluoride analysis by a continuous flow analyzer (ARDC analytical team).
Figure 3. Location of Alma smelter and its two air monitoring stations. Arrows indicate the prevailing winds.
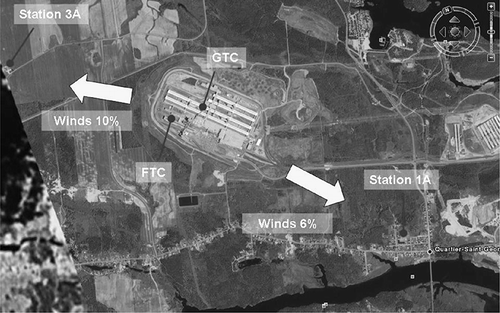
Stable and radiogenic isotopes were analyzed on the last quarter of the cellulosic filter by the Université du Québec à Montréal (UQAM-GEOTOP) as follows: (1) reverse polarity thermo-ionization mass spectrometry (N-TIMS) for osmium,Citation18 (2) multicollector–ICP–mass spec-trometry (MC-ICP-MS) for lead,Citation19 (3) isotope ratio mass spectrometry (IRMS) for carbon and nitrogen,Citation20 and (4) TIMS for strontium and neodymium.Citation21,Citation22
RESULTS AND DISCUSSION
Part 1: Quantification of PM2.5 Emitted by the Sebree Smelter
One should note that all results are expressed in grams of PM by metric ton of aluminum produced. This ratio approach is mandatory to adequately compare PM emissions between different sites of aluminum production. In addition, only results falling in the 80–120% isokinetics are considered in this study.
Part 1.1: Emissions from Stacks
First, clearly indicates that using method 202 or OTM28 for CPM measurements largely influences the results. Indeed, because CPM are included in PM2.5 and PMtotal, differences observed between the 2006 and 2008 results can only be explained by a 60% decrease in CPM results for GTC and FTC. This highlights the importance of an accurate CPM assessment, given that they make up 55 and 95% of GTC and FTC PMtotal emissions, respectively (). Furthermore, total CPM are reduced in parallel with inorganic CPM content (data not shown), which suggests that inorganic gases are the main source of secondary aerosols for aluminum smelters, the industry being well-known to emit hydrogen fluoride (HF) and SO2.Citation23
Table 1. Comparisons of mean PM emission coefficients ± standard error of the mean at the Sebree smelter between (1) EPA CTM040/202 and OTM27/28 at GTC and FTC, and between (2) the M14* and Rio Tinto Alcan CYCLEX candidate method at roof vents
Table 2. Ratios of CPM and PM2.5 to PMtotal for the Rio Tinto Alcan Sebree smelter
The difference between GTC and FTC ratios is also worth noting ( and ). GTC stacks emit 3–5 times more PM than FTC stacks as each treatment unit is linked to completely different processes. The FTC recovers fumes from the anode baking furnace (ABF), where green anodes from the paste plant – where the anode paste (pitch and coke) is crushed, ground, and mixed – are baked. The fumes are then scrubbed with fresh alumina. Considering the ABF process, several other gaseous species than dust are expected, such as polyaromatic hydrocarbon, VOC, HF, and SO2.Citation23 It is therefore not surprising that CPM accounts for 95% of PMtotal. As for potrooms, where electrolysis takes place - electric current passes through a carbon cathode and a prebaked anode (see above) to reduce alumina into molten aluminum. Process gases from cells are collected and dry-scrubbed with alumina at the GTC. Bath components, alumina, and carbon from consumed anodes are the main sources of primary PM, in a larger proportion than at the FTC. At the GTC exhaust, SO2 (∼ 120 ppm) and HF, in a smaller proportion (< 1 ppm), are precursors of CPM.
Part 1.2: Emissions from Roof Vents
At first, the M14* and M14/OTM27/OTM28 methods were tested at the roof vents (data not shown), but no statistical differences arose. This was expected because SO2 concentrations in the potrooms are rather diluted (<2 ppm) and hence should not cause any artifacts. Emission coefficients from the M14* method yield results approximately 10 times higher than at the GTC. By far, roof vents are undeniably the primary contributors (85%) of total PM2.5 released by an aluminum smelter (GTC + FTC + roof vents, other sectors such as anode butt recycling or paste plants are marginal in contribution—data not shown); 56% of this total is CPM, still only from roof vents ( and ). All primary PM emissions are essentially the result of cell operation (anode changes, metal tapping, alumina feeding, etc.) and condensables are attributable to imperfect gas suction efficiency in cells. Hence, lowering PM emissions relies on increasing pot gas suction to recover primary PM emissions and gases responsible for CPM, both leaking from the pots.
To determine if the M14* method and the Rio Tinto Alcan CYCLEX candidate method are equivalent whatever the year of sampling (H0), a statistical analysis was conducted. First, Gaussian distribution and variance homogeneity were confirmed by graphical examination of the residuals.Citation24 The systematic and random errors (bias and precision) of the two methods were considered as the same constant because every weighing was conducted on the same kind of analytical balance. This error constant does not then impact further comparisons of the two methods. It is also worth mentioning the lack of standard or reference samples for repeat sampling runs on PM2.5, making it impossible to determine a bias between the two methods. Therefore, a two-way ANOVA type I (data not matched) was performed. Next, how the PM2.5 measurements at roof vents are affected was assessed by two independent variables for the main effects: year of measurements (2007 vs. 2008) and the method (M14* vs. CYCLEX). Lastly, if one factor affected the other was checked, what is called the “interaction effect” (year x method). clearly shows that neither the interaction effect (year × method) nor the main effects have an effect on the model (P > 0.05). Also, the critical F-value (0.05, 1, 30) =4.171 indicates that H0 cannot be rejected; that is, F(0.05, 1, 30) > F. Therefore, there is no evidence that any of the predictors are linearly associated with the response. It is also interesting to note that the interaction effect accounts for only 1.03% of the total variance. In other words, whatever the year of measurements, here it is demonstrated that the M14* and CYCLEX methods are interchangeable.
Table 3. Two-say ANOVA on PM2.5 measuements at roof vents as affected by the year (2007 vs.2008 and the method (M14* vs. CYCLEX)
At this point, it should be noted that the CYCLEX method only seeks to give CPM and PM2.5 emission factors at roof vents, but its design also allows measuring PMtotal. Therefore, for PM2.5 estimation (including CPM) in aluminum smelters not equipped with downcomers, the CYCLEX method seems suitable.
Part 2: Speciation of PM2.5 in the Surrounding Environment of the Alma Smelter
Stations 3A and 1A () are respectively exposed to 10 and 6% of the winds passing through the smelter area, along with other emission sources that are located around these sampling sites (gas stations, pulp and paper mills, residential areas, farms). First, stations 3A and 1A show respective mean PM2.5 concentrations of 13.3 and 8.2 μg·m−3 over the same 14-day period. Considering winds blowing from the smelter directly to either station and that 100% of the PM2.5 comes from the smelter, station 3A and 1A measurements yield 1.3 and 0.49 μg·m−3, respectively, during the study period. Obviously, this calculation does not consider any other sources than the Alma smelter; however, farming heavily impacts station 3A, and cars and other urban activities affect station 1A.
Furthermore, from a toxicity perspective, determining the source profile for an aluminum smelter is worthwhile. Note that source apportionment calculations recognized by EPA (e.g., UNMIX, positive matrix factorization, or othersCitation25) were not used here because of a lack of long-term data, which these models require. As a first attempt, inorganic species in industrial versus ambient samples were measured (), and the results are expressed as PM2.5 mass fraction (species mass on the filter/total PM2.5 mass on the filter, in percent). From , it appears that species like Ga, Zr, Cd, and Be are neither detected at smelter stacks (FTC and GTC) nor in the ambient urban air. For Cr, Fe, Mg, Mn, Ca, Na, Li, Si, V, Ti, and Cu, there is an enrichment between stack emissions and the urban stations that suggests other sources (e.g., transportation, pulp and paper mills, etc.). For all other species, in particular Al, Pb, and F, mass fractions in the PM2.5 filters collected at stacks are higher than those collected in the ambient urban air, which clearly indicates emissions from the smelter to the surrounding environment.
Table 4. PM2.5 mass fraction of various inorganic species (species mass on the filter/total mass of PM2.5 on the filter—in percent) at the smelter stacks (FTC and GTC) and in its surrounding environment (urban stations 1A and 3 A)
Unfortunately, elemental and organic carbon contents as well as nitrates, sulfates, and ammonium were not determined on urban filters for technical reasons. Further analyses will be necessary to better assess the effects of the smelter on ambient concentrations of these species.
Secondly, SEM-EDX tests allowed us to pinpoint some specific species released or used in aluminum smelters. Thus, from a qualitative point of view, it was determined that fine particles collected in the surrounding environment contained particles related to the electrolytic bath (chiolite, cryolite, sodium aluminum tetrafluo-ride, fluorinated alumina). At the same time, the above speciation results were confirmed in that most EDX analyses reported other types of particles (i.e., not smelter related). Indeed, , a and b, shows EDX diagrams that match quite well for typical bath species in an aluminum smelter (same-intensity F and Na peaks and a higher Al peak), although shows particles that do not originate from the smelter (high Ca content and no Na).
Figure 4. SEM-EDX on GTC and urban station filters. Picture of spotted area on filters and diagram of elemental determination. EDX analysis on (a) the GTC filter, (b) the urban station 1A filter, and (c) the urban station 1A filter.
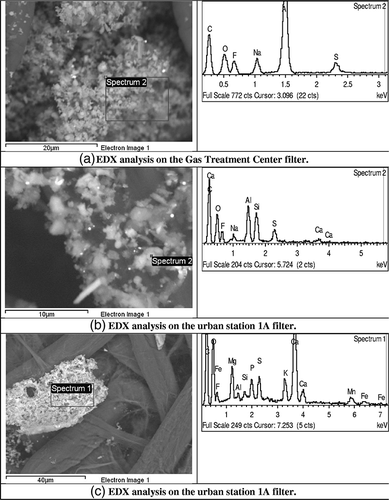
Lastly, preliminary isotope measurements demonstrate that this method could be used to track aluminum smelter PM2.5 emissions. Stable and radiogenic isotopes 13C/12C (δ13C = −26.3 ± 0.2), 87Sr/86Sr (0.71847 ± 0.00002), and 143Nd/144Nd (0.512197 ± 0.000015) cannot be distinguished from terrestrial plants (U.S. Geological Survey database), typical soil dust,Citation26 or weathering of till.Citation27 However, with osmium and lead, results are very promising. Analyses were done after the process, first on anodes (main carbon raw material in the electrolytic process), then at the GTC stack (point of emission of the electrolysis), and lastly in the surrounding environment (i.e., at field station 3A) (see ). In anodes, the ratio 187Os/188Os =2.393 ± 0.005 is significantly different from that of usual anthropogenic sources,Citation28 whereas ratios for 206Pb/204Pb =18.759 ± 0.005, 207Pb/204Pb = 15.625 ± 0.005, 208Pb/204Pb =38.542 ± 0.007, and 206Pb/207Pb =1.201 ± 0.002 are comparable with U.S. aerosol emissions for plant carbon sources originating from the Mississippi ValleyCitation29 (). This means that the anodes used at the Alma facility own a specific isotopic footprint once coupled with Os and Pb (). To evaluate smelter emissions contribution to the ambient air at station 3A, the Os ratio is considered as a mixture of smelter emissions and other sources. Considering Equationeq 1,Citation30
Figure 5. Lead isotope diagram for samples in the carbon raw material (anode), at the stack output (GTC), and in the surrounding environment of the smelter (station) compared with North American aerosols. Adapted with permission from Poirier.Citation29 Copyright 2006 Elsevier, Earth and Planetary Science Letters.
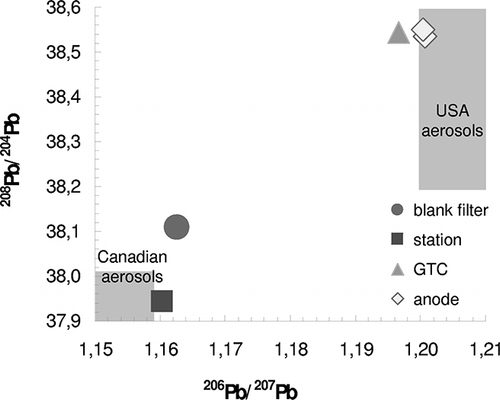
Figure 6. Osmium-lead isotope diagram for samples in the carbon raw material (anode), at the stack output (GTC), and in the surrounding environment of the smelter (station).
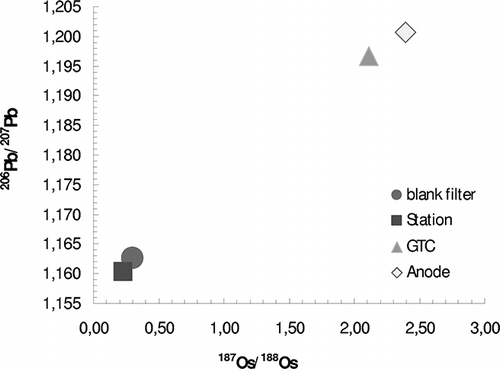
where R PM2.5 is the 187Os/188Os ratio at station 3A (0.23 measured). R sources is the sum of the R i·X i 187Os/188Os ratios for all other sources, from usual anthropogenic sources such as catalytic converters (0.175Citation31) to natural ones. In this case, one should note that the natural isotopic signature of Os is not considered. Indeed, the accepted value of 1.4Citation32 does not make a consensus because this value is not site specific.Citation33 Therefore, only the most common R sources ratio in the literature were used—catalytic converters. R smelter is the same Os ratio for smelter emissions (2.11 measured). X sources, which is actually equal to (1 – X smelter), and the unknown term X smelter are the contributing PM2.5 factors for Os ratios. With this kind of preliminary approach, the calculation yields a contribution, from the Alma smelter, of approximately 3% at urban station 3A.
CONCLUSIONS
Part 1: Quantification of PM2.5 Emitted by the Sebree Smelter
Part 1.1: Emissions from Stacks
All of the experience gained at Sebree Works led Rio Tinto Alcan to consider EPA OTM27 and OTM28 methods as the best approaches to quantify CPM, PM2.5, and PMtotal. Indeed, as mentioned in earlier studies,Citation34 the wet impinger approach overestimates CPM (EPA 202), especially for aluminum smelters, because of SO2 concentrations, whereas this is not the case with dry impingers (OTM28).
Part 1.2: Emissions from Roof Vents
For aluminum smelters without downcomers or for other industries with high ventilated building surfaces, the Rio Tinto Alcan CYCLEX candidate method is, to the best of the author's knowledge, the only way to achieve PM2.5 (including CPM) emission coefficients. It is also essential to consider a representative location of all of the potrooms during CYCLEX sampling campaigns and to measure over a full potroom cycle length.
Part 2: Speciation of PM2.5 in the Surrounding Environment of the Alma Smelter
This study provides a first but incomplete speciation of compounds released by an aluminum smelter to the surrounding environment. As expected, considering the electrolytic process, Al, Pb, and F are the main elements in PM2.5 originating from a smelter. Nevertheless, the carbon fraction (organic and elemental) and the anionic species are unknown; they require further work to focus on the source apportionment approach by species. A technique such as SEM-EDX is an interesting approach to qualitatively discriminate fine particles from one source to another, but it demands a thorough knowledge of source particle signature. On the contrary, the isotopic approach, especially with the ratio 187Os/188Os, reveals promising results to easily track PM2.5 smelter emissions in the ambient air because the isotopic ratio of the targeted source appears to be unique. In the future, Rio Tinto Alcan will focus its efforts on this technique.
ACKNOWLEDGMENTS
The author thanks Claude Perron (ARDC), Jacques Bélanger and Rock Emond (Alma Works), Ghassan Dugaish and Jamie Coomes (Sebree Works), and Paul Siegel and his team (Air Quality Services, Kentucky) for sampling and technical support. Rio Tinto Alcan also acknowledges Professor André Poirier from UQAM-GEOTOP for all of the work with isotopes. Rio Tinto Alcan also gives special thanks to Yvan Lavoie and Jacques Labrie (now retired from Rio Tinto Alcan) for the first works on the PM2.5 program.
REFERENCES
- Slanina , J. 2007 . Air Pollution: The Emission-Effect Relation . Rev. Environ. Sci. Biotechnol. , 6 : 353 – 374 .
- Pace , G.T. 1998 . “ Composition of PM2.5 in the Ambient Air ” . In Proceedings, PM2.5: A Fine Particle Standard , 3 – 12 . Pittsburgh , PA : A&WMA .
- Roman , H.A. , Walker , K.D. , Walsh , T.L. , Conner , L. , Richmond , H.M. , Hubbell , B.J. and Kinney , P.L. 2008 . Expert Judgment Assessment of the Mortality Impact of Changes in Ambient Fine Particulate Matter in the U.S . Environ. Sci. Technol. , 42 : 2268 – 2274 .
- Bennett , C.M. , Simpson , P. , Raven , J. , Skoric , B. , Powell , J. , Wolfe , R. , Walters , E.H. and Abramson , M.J. 2007 . Associations between Ambient PM2.5 Concentrations and Respiratory Symptoms in Melbourne, 1998–2005 . J. Toxicol. Env. Heal. A. , 70 : 1613 – 1618 .
- Englert , N. 2004 . Fine Particles and Human Health—a Review of Epidemio-logical Studies . Toxicol. Lett. , 149 : 235 – 242 .
- Gilmour , P.S. , Morrison , E.R. , Vickers , M.A. , Ford , I. , Ludlam , C.A. , Greaves , M. , Donaldson , K. and MacNee , K. 2005 . The Procoagulant Potential of Environmental Particles (PM10) . Occup. Environ. Med. , 62 : 164 – 170 .
- Schlesinger , R.B. 2007 . The Health Impact of Common Inorganic Components of Fine Particulate Matter (PM2.5) in Ambient Air: A Critical Review . Inhal. Toxicol. , 19 : 811 – 832 .
- Ballester , F. , Medina , S. , Boldo , E. , Goodman , P. , Neuberger , M. , Iñiguez , C. and Künzli , N. 2008 . Reducing Ambient Levels of Fine Particulates Could Substantially Improve Health: A Mortality Impact Assessment for 26 European Cities . J. Epidemiol. Commun. H. , 62 : 98 – 105 .
- Prati , P. , Zucchiatti , A. , Lucarelli , F. and Mandò , P.A. 2000 . Source Apportionment near a Steel Plant in Genoa (Italy) by Continuous Aerosol Sampling and PIXE Analysis . Atmos. Environ. , 34 : 3149 – 3157 .
- Sheya , S.A.N. , Glowacki , C. , Chang , M.-C.O. , Chow , J.C. and Watson , J.G. 2008 . Hot Filter/Impinger and Dilution Sampling for Fine Particulate Matter Characterization from Ferrous Metal Casting Processes . Journal of the Air & Waste Management Association , 58 : 553 – 561 . doi: 10.3155/1047-3289.58.4.553
- Kuo , S.-C. , Hsieh , L.-Y. , Tsai , C.-H. and Tsai , Y.I. 2007 . Characterization of PM2.5 Fugitive Metal in the Workplaces and the Surrounding Environment of a Secondary Aluminum Smelter . Atmos. Environ. , 41 : 6884 – 6900 .
- Conditional Test Method 040 (CTM040), Method for the Determination of PM10 and PM2.5 Emissions (Constant Sampling Rate Procedures); U.S. Environmental Protection Agency; 2002 http://www.epa.gov/ttn/emc/ctm/ctm-040.pdf (http://www.epa.gov/ttn/emc/ctm/ctm-040.pdf) (Accessed: 2010 ).
- Method 202—Condensable Particulate Matter; U.S. Environmental Protection Agency; 1996 http://www.epa.gov/ttn/emc/promgate/m-202.pdf (http://www.epa.gov/ttn/emc/promgate/m-202.pdf) (Accessed: 2010 ).
- Other Test Method 27 (OTM27)—Determining PM10 and PM2.5 Emissions from Stationary Sources (Supersedes CTM-040); U.S. Environmental Protection Agency; 2009 http://www.epa.gov/ttn/emc/prelim/otm27.pdf (http://www.epa.gov/ttn/emc/prelim/otm27.pdf) (Accessed: 2010 ).
- Other Test Method 28 (OTM28)—Dry Impinger Method for Determining Condensable Particulate Emissions from Stationary Sources; U.S. Environmental Protection Agency; 2009 http://www.epa.gov/ttn/emc/prelim/otm28.pdf (http://www.epa.gov/ttn/emc/prelim/otm28.pdf) (Accessed: 2010 ).
- Richards , J. , Holder , T. and Goshaw , D. Optimized Method 202 Sampling Train to Minimize the Biases Associated with Method 202 Measurement of Condensable Particulate Matter Emissions . Presented at the A&WMA Hazardous Waste Combustion Specialty Conference . St. Louis , MO .
- Method 14—Fluoride for Primary Aluminum Plants; U.S. Environmental Protection Agency; 2000 http://www.epa.gov/ttn/emc/promgate/m-14.pdf (http://www.epa.gov/ttn/emc/promgate/m-14.pdf) (Accessed: 2010 ).
- Creaser , R.A. , Papanastassiou , D.A. and Wasserburg , G. J. 1991 . Negative Thermal Ion Mass Spectrometry of Osmium, Rhenium and Iridium . Geochim. Cosmochim. Acta , 55 : 397 – 401 .
- Belshaw , N.S. , Freedman , P.A. , O'Nions , R. K. , Frank , M. and Guo , Y. 1998 . A New Variable Dispersion Double-Focusing Plasma Mass Spectrometer with Performance Illustrated for Pb Isotopes . Int. J. Mass Spectrom. , 181 : 51 – 58 .
- Hélie , J.F. 2009 . Elemental and Stable Isotopic Approaches for Studying the Organic and Inorganic Carbon Components in Natural Samples . IOP Conf. Series: Earth Environ. Sci. , 5 doi: 10.1088/1755–1307/5/1/012005
- Platzner , I.T. 1997 . Modern Isotope Ratio Mass Spectrometry , New York : Wiley .
- Richard , P. , Shimizu , N. and Allègre , C.J. 1976 . 143Nd/144Nd, a Natural Tracer: An Application to Oceanic Basalts . Earth Planet. Sci. Lett. , 31 : 269 – 278 .
- 2001 . Integrated Pollution Prevention and Control. Reference Document on Best Available Techniques in the Nonferrous Metals Industries , Brussels , , Belgium : European Commission .
- Solomon , P.A. , Hopke , P.K. , Froines , J. and Scheffe , R. 2008 . Key Scientific Findings and Policy- and Health-Relevant Insights from the U.S. Environmental Protection Agency's Particulate Matter Supersites Program and Related Studies: An Integration and Synthesis of Results . Journal of the Air & Waste Management Association , 58 S3–S92 doi: 10.3155/1047-3289.58.13.S-3
- Montgomery , D.C. 1991 . Design and Analysis of Experiments , Toronto, Ontario , , Canada : John Wiley & Sons .
- Simonetti , A. , Gariépy , C. and Carignan , J. 2000 . Pb and Sr Isotopic Evidence for Sources of Atmospheric Heavy Metals and Their Deposition Budgets in Northeastern North America . Geochim. Cosmochim. Acta , 64 : 3439 – 3452 .
- Ohlander , B. , Ingri , J. , Land , M. and Schöberg , H. 2000 . Change of Sm-Nd Isotope Composition during Weathering of Till . Geochim. Cosmochim. Acta , 64 : 813 – 820 .
- Peucker-Ehrenbrink , B. and Ravizza , G. 2000 . The Marine Osmium Isotope Record . Terra Nova , 12 : 205 – 219 .
- Poirier , A. 2006 . Re-Os and Pb Isotope Systematics in Reduced Fjord Sediments from Saanich Inlet (Western Canada) . Earth Planet. Sci. Lett. , 249 : 119 – 131 .
- De la Cruz , M.T. , Laborda , F. , Callén , M.S. , López , J.M. and Mastral , A.M. 2009 . Study of Pb Sources by Isotope Ratios in the Airborne PM10 of Zaragoza, Spain . J. Environ. Monitor. , 11 : 2052 – 2057 .
- Poirier , A. and Gariépy , C. 2005 . Isotopic Signature and Impact of Car Catalysts on the Anthropogenic Osmium Budget . Environ. Sci. Technol. , 39 : 4431 – 4434 .
- Meisel , T. , Walker , R.J. , Irving , A.J. and Lorand , J.P. 2001 . Osmium Isotopic Composition of Mantle Xenoliths: A Global Perspective . Geochim. Cosmochim. Acta , 8 : 1311 – 1323 .
- Rodushkin , I. , Engström , E. , Sörlin , D. , Pontér , C. and Baxter , D.C. 2007 . Osmium in Environmental Samples from Northeast Sweden. Part II. Identification of Anthropogenic Sources . Sci. Total Environ. , 386 : 159 – 168 .
- Condensable Particulate Matter Test Methods Stakeholder Meeting . Conference Proceeding, U.S. Environmental Protection Agency . Washington , DC .