ABSTRACT
The Windsor, Ontario Exposure Assessment Study evaluated the contribution of ambient air pollutants to personal and indoor exposures of adults and asthmatic children living in Windsor, Ontario, Canada. In addition, the role of personal, indoor, and outdoor air pollution exposures upon asthmatic children's respiratory health was assessed. Several active and passive sampling methods were applied, or adapted, for personal, indoor, and outdoor residential monitoring of nitrogen dioxide, volatile organic compounds, particulate matter (PM; PM ≤ 2.5 μm [PM2.5] and ≤ 10 μm [PM10] in aerodynamic diameter),elemental carbon, ultrafine particles, ozone, air exchange rates, allergens in settled dust, and particulate-associated metals. Participants completed five consecutive days of monitoring during the winter and summer of 2005 and 2006. During 2006, in addition to undertaking the air pollution measurements, asthmatic children completed respiratory health measurements (including peak flow meter tests and exhaled breath condensate) and tracked respiratory symptoms in a diary. Extensive quality assurance and quality control steps were implemented, including the collocation of instruments at the National Air Pollution Surveillance site operated by Environment Canada and at the Michigan Department of Environmental Quality site in Allen Park, Detroit, MI. During field sampling, duplicate and blank samples were also completed and these data are reported. In total, 50 adults and 51 asthmatic children were recruited to participate, resulting in 922 participant days of data. When comparing the methods used in the study with standard reference methods, field blanks were low and bias was acceptable, with most methods being within 20% of reference methods. Duplicates were typically within less than 10% of each other, indicating that study results can be used with confidence. This paper covers study design, recruitment, methodology, time activity diary, surveys, and quality assurance and control results for the different methods used.
It is important to obtain data to identify any factors that can influence the relationships among personal, indoor, and outdoor concentrations for a range of air pollutants. Ensuring that the methods used are valid and comparable to reference methods used in typical air pollution, monitoring is crucial for data to be of use to regulators. These exposure data can then be used to develop risk management policies that reduce personal and indoor exposures to air pollutants.
INTRODUCTION
The challenges of assigning exposure on an individual basis have received increasing commentary in the literature with several studies being conducted to better assess the impact of exposure misclassification in health effects research.Citation1–3 The impact of air pollutants on the health of susceptible populations such as the elderly,Citation4 diabetics,Citation5 children,Citation6 and asthmaticsCitation7–10 indicate that exposure sources and baseline health are important factors for guiding regulatory decisions related to ambient or indoor air quality guidelines or standards.
Human exposure to air pollution is influenced by indoor and outdoor sources as well as personal activities; the complex interplay of these factors complicates the interpretation of personal exposures. This research will address these challenges by assessing exposures for adult populations and asthmatic children.
There are several air pollutants known to have potential human health impacts, including particulate matter (PM; PM ≤ 2.5 μm [PM2.5] and ≤ 10 μm [PM10] in aerodynamic diameter), nitrogen dioxide (NO2), allergens in settled dust, volatile organic compounds (VOCs), and ozone (O3), as well as constituents of PM2.5 such as elemental carbon (EC), ultrafine particles (UFPs), and metals. It is important to identify all potential sources of personal exposures to these pollutants in an effort to understand source-specific impacts as well as the potential for misclassification of exposure and the role that this may have on discerning health impacts. Outdoor sources include traffic emissions, industrial emissions, long-range transport, secondary formation of pollutants in the atmosphere, and personal activities such as refueling vehicles and using a barbecue. Outdoor-generated pollutants also affect indoor environments via infiltrationCitation11–13; therefore, housing characteristics that affect infiltration are important to consider. Indoor sources of exposure also include off-gassing of building materials, combustion processes such as cooking, use of personal care and cleaning products, cigarette smoke, and presence of pets.Citation14 Several studies that have included personal, indoor, and outdoor pollutant measurements have found that personal exposures can exceed indoor and outdoor concentrations.Citation15–18 These findings suggest that not all personal exposures are captured by residential indoor and outdoor measurements. In the case of particulate air pollution, simple movement by residents in the home can resuspend particles from clothes or carpeting. Being close to gas stoves may increase exposure to NO2 indoors. Recent literature suggests that time spent in traffic can contribute significantly to personal exposures for various air pollutants.Citation19–21 In addition, locations such as work, school, restaurants, and other indoor locations are also known to contribute to personal exposures.Citation22,Citation23
As part of the Border Air Quality Strategy (BAQS), the Windsor, Ontario Exposure Assessment Study (WOEAS) was conducted in 2005 and 2006 in Windsor, Ontario, to understand air quality issues in this area. One objective was to examine the relationships between indoor and outdoor concentrations and personal exposures to various air pollutants, including PM, O3, NO2, and VOCs. The goal of this research was to develop a better understanding of factors affecting these relationships for adults and asthmatic children. A second objective was to examine the role of personal, indoor, and outdoor air pollution exposures upon asthmatic children's respiratory health with the goal of identifying what sources of exposure had the greatest impact upon health.
Windsor is a relatively small geographical area (120.6 km2) that is impacted by various ambient air pollution sources. It is the site of one of the major border crossings to the United States and is therefore affected by large volumes of commercial truck traffic. Windsor also has several local industries such as automobile manufacturing. Finally, there is long-range transportation of pollutants from the United States.Citation24,Citation25
This manuscript presents the study design and methodology as well as important data for method validations including quality assurance and control. It includes summary results for recruitment, time activity diaries, and surveys.
MATERIALS AND METHODS
Study Design
Health Canada and the University of Windsor conducted a personal exposure study with Windsor adults in 2005 and asthmatic children in 2006. Personal and residential indoor and outdoor exposures were assessed over a period of 10 days, with a total of 5 sampling days each in the winter (January to March) and summer (July to August) of each year. Pollutants included in the study were NO2, VOCs, O3, UFPs, EC, PM2.5, PM10, and components of PM including EC, nitrate, and metals. Ancillary measurements included air exchange rates (AERs), temperature, relative humidity (RH), and settled dust as well as respiratory health measures collected for asthmatic children in 2006. Sampling began Monday evenings at approximately 4:00 p.m. and ended on Saturday evenings at approximately the same time. At the end of each 24 ± 3-hr interval, teams of two technicians visited each home to refurbish sampling equipment, check for mechanical malfunctions, and administer questionnaires. All data were collected over 8 consecutive weeks per season, with a total of six homes being sampled concurrently. Personal sampling was conducted by having participants carry a small backpack housing air pollution monitoring equipment. Participants were asked to keep the backpack with them throughout their daily activities, although no direct methods were applied to assess compliance. If they were in one location for an extended period of time (i.e., at school or work) they were instructed to place the backpack close to them with the sampling inlet facing up. Indoor residential monitoring was conducted by placing equipment inside of the participant's home (i.e., typically within the family or living room where participants spent a substantial amount of time). Outdoor residential monitoring was located in the backyard several meters away from the home and away from any combustion sources such as barbecues and driveways. Indoor and outdoor residential sampling was conducted at breathing height (1.5 m). The WOEAS design paralleled that of the U.S. Environmental Protection Agency's (EPA) Detroit Exposure and Aerosol Research Study (DEARS) with respect to sampling periods, days of the week, and survey and questionnaire designCitation18 so that environmental data from both cities could be used to investigate border air quality issues in the region. provides details for the methods and instrumentation used in the WOEAS.
Table 1. Target variables and instrumentation
Approval was obtained from Health Canada and the University of Windsor Research Ethics Boards to conduct this study and all personal information is protected according to the Canadian Access to Information Act and the Privacy Act.
Sample Population: Recruitment and Retention
In 2005, an initial pool of potential volunteer participant families was identified from the Windsor Children's Respiratory Health Study.Citation7 From the pool of potential participants, homes meeting inclusion criteria were randomly selected and their adult residents were approached for participation. Adults were considered eligible for study inclusion if they were nonsmoking, living in a detached home, had an asthmatic child, were not occupationally exposed to VOCs, and did not have any workplace restrictions on carrying the personal monitoring equipment. Using these criteria, a pool of 90 eligible volunteers was established. Among the eligible volunteers, preference was subsequently given to households that were spatially distributed across Windsor.
In 2006, eligible participants included physician-diagnosed asthmatic children between 10 and 13 yr of age. Of the available pool of candidates drawn from a previous study of 186 asthmatic children,Citation4 further consideration was given to ensuring an approximately even spatial distribution of homes across Windsor. identifies residential locations.
Given the above mentioned eligibility criteria and the need to ensure that there would be sufficient statistical power to assess the role of personal, indoor, and outdoor air pollution exposures upon asthmatic children's respiratory health, a power calculation was conducted. Statistical power estimates were calculated and applied for both years by taking into account the repeated measures design of the study. Because of correlations within the data, standard power calculations were adapted to account for dependencies. Using a methodology described by Killip et al.,Citation26 the effective sample size was first calculated. This involved estimating the intraclass correlation coefficient (ICC) for the study participants using personal monitoring data collected in previous publications; their values of the variance of forced expiratory volume in 1 sec (FEV1) scores in asthmatic children were also used.Citation27,Citation28
The ICC is a ratio of the variability between subjects to the total variability,
The effective sample size was then estimated by dividing the total number of planned observations (n = 480) each year by the design effect. As outlined by Killip et al.,Citation26 the design effect was estimated as
On the basis of these calculations, 48 participants were recruited in each of the years. The study design was not intended to recruit a representative selection of the population but rather to identify homes of susceptible individuals.
Participant retention was encouraged through several techniques. Before recruitment, two technicians visited each residence to demonstrate the monitoring equipment as well as to answer any questions. During this visit, consent was obtained, the baseline housing questionnaire was completed, visit schedules were discussed, and suitable locations for the indoor and outdoor monitoring equipment were identified. At the end of each season, participants were provided with a personalized report describing their individual data in comparison to others who were monitored during the same week. These reports contained guidance material from the Canadian Mortgage and Housing Corporation and Health Canada on different air pollutants. These were provided at a meeting where the principal investigator discussed study findings and was available to answer participants' questions. Between sampling seasons in 2006, children were invited to a pizza party with their parents to provide them with the opportunity to meet other participating children and discuss their experiences.
Passive Samplers
During 2005 and 2006, personal, indoor, and outdoor Ogawa passive samplers (Ogawa & Company) were used to measure exposures to O3 and NO2. The O3 badge was only used for personal and outdoor monitoring. The sampler used a single nitrite-coated quartz-fiber filter purchased from the manufacturer. When O3 is present in the sampled air, it diffuses into the filter and oxidizes the nitrite to nitrate on an equimolar basis. The NO2 badge uses a single carbonate-coated quartz-fiber filter (also purchased from the manufacturer) to trap NO2.Citation18 Sampling times were approximately 24 ± 3 hr for each badge. The badges were located within the breathing zone for the personal samples and were placed in a manifold-type device that housed all of the active and passive samplers, thus ensuring constant airflow across the face of the passive badges. demonstrates the personal, indoor, and outdoor monitoring equipment setup. All Ogawa badges were refrigerated during storage and shipping.
Figure 2. Picture of equipment setup: (a) indoor and outdoor monitors, (b) personal setup, and (c) VOC canister.
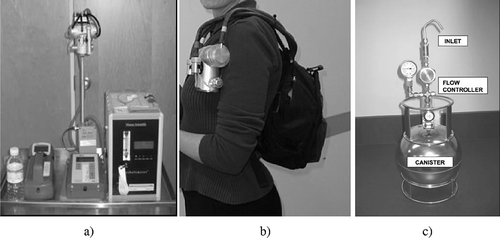
The Ogawa filters were analyzed according to the Ogawa Standard Operating Protocols. The only deviation from the O3 method was that the protocol assumes two filters were used for measurements and in the Windsor situation there was only one filter. The sampling rate was therefore half of the rate cited in the protocol. After exposure, the O3 nitrite-coated filter was extracted with ultrapure (Milli-Q) water, whereas the NO2 carbonate-coated filter was extracted with 0.09% (v/v) hydrogen peroxide. Both extracts were analyzed by ion chromatography (IC) using Dionex DX-300 or DX-600 IC systems. Nitrate from the extracts of the O3 filter was analyzed using a Dionex IonPacAS15 column and gradient elution, whereas nitrate and other anions extracted from the NO2 filter was analyzed using a Dionex-AS4A column with carbonate/bicarbonate eluent. Calibration checks were performed daily before analysis of the field samples and once in every 10–15 samples using standards prepared from National Institute for Standards and Technology (NIST) traceable standards.
Selected VOCs in air were collected using cleaned and evacuated Summa canisters. During both years, indoor and outdoor measurements were made at each of the residences using 6-L canisters deployed every 24 hr. During 2005, the adult participants also carried a 1-L Summa canister within the padded backpack to monitor their personal exposures. The canisters sampled at flow rates of 3.5 and 0.5 mL/min for the 6- and 1-L canisters, respectively. The passive canister sampling systems included four basic components: an in-line Swagelok filter with a 2-μm stainless-steel sintered filter to eliminate particulates, a restrictor, a Veriflow SC423XL back-pressure flow regulator, and a vacuum gauge. The back-pressure flow regulator ensured that approximately a 0.5- to 1-psi pressure drop across the restrictor was maintained until the canister was within 1 to 2 psi of reaching atmospheric pressure, after which the regulator no longer maintained a 1-psi differential across the orifice, resulting in a drop in flow rate. The flow controllers were assembled in the laboratory and leak tested. EPA Compendium Method TO-15 requires that the flow controllers be certified clean before use. The flow controllers were certified as clean by passing humidified, high-purity air through the flow controller into evacuated canisters that were then analyzed by gas chromatography–mass spectrometry; if no VOC concentrations were greater than 0.2 parts per billion by volume (ppbv), the flow controllers were determined to be clean. The certified flow controllers were then capped with Swagelok fittings and shipped to the site for sampling. The Summa canister analysis methods followed the EPA Compendium Method TO-15. The 2005 VOC analytical methods and quality assurance data have been published elsewhere.Citation17
Continuous Measurements
Continuous measurements of PM2.5, UFPs, EC, and temperature/RH were collected indoors and outdoors at each residence using DustTrak (model 8520, TSI), PTrak (model 8525, TSI), Aethalometer (AE-42, Magee Scientific Company), and Smart Reader Plus 2 (ACR Systems, Inc.) monitors, respectively. These methods and their validation are included in a companion manuscript detailing their performance,Citation29 and precision and limits of detection (LOD) calculation methods are summarized briefly below.
Two DustTrak instruments (one indoors and one outdoors) were deployed at each residence; these are optical instruments capable of measuring particles from approximately 0.3 μm in diameter up to 2.5 μm. The PTrak measurements of UFP number concentrations were also conducted indoors and outdoors but for only 10 min/hr in each location (twenty 30-sec averages) because of their limited alcohol storage reservoir volume. Although PTraks count all particles from 20 nm to 1 μm, the instrument is considered to monitor mainly UFP because approximately 80–99% of these particles are below 0.1 μm.Citation30
The Aethalometer measures light absorption from particles collected on a quartz fiber tape. Because only a limited number of Aethalometers were available, only one unit was operated at each residence to sample EC indoors and outdoors; the intake was programmed to switch between indoor sampling and outdoor sampling every 30 min during the day and hourly at night. The PTrak instruments were programmed to synchronize with the Aethalometer as it switched from an indoor to an outdoor air intake.
In 2006, the asthmatic children carried an active sampling personal DataRAM (pDR) (Thermo Scientific) to measure continuous PM2.5; the cut size was ensured by pumping the intake air through a 1.8-L/min PM2.5 per sonal environmental monitor (PEM; Chempass System R&P/Thermo) with no filter present. Like the DustTrak, the pDR uses optical means to measure particles smaller than 2.5 μm and is laboratory calibrated to a NIST particle standard.Citation22 The pDR uses a laser at higher frequency (i.e., lower wavelength) than the DustTrak; therefore, the highest sensitivity regions for these instruments occurred at somewhat different diameters.
Active Samplers
PM (PM2.5 and PM10) was measured using the R&P Chempass multipollutant PEM (Chempass System R&P/Thermo) as described in Demokritou et al.Citation31 Teflon filters were preconditioned for 24 hr before mass measurement, following EPA quality assurance guidelines,Citation32 at Health Canada's Archimedes M3 buoyancy-corrected gravimetric analysis facility.Citation33 Average daily SD of blank reference filter measurements was typically ±0.5–0.6 μg (n = 10–14), resulting in daily laboratory detection limits (LDLs) of 1.5–1.6 μg on the basis of 3 times the standard deviation (SD).Citation34 Method detection limits for the study presented here are based on variability introduced throughout all preweighing and postweighing steps, including storage for the entire time elapsed from removal of the filter from its packaging to the final postweighing (typically 1–2 months).
PM2.5 and PM10 measurements were collected in all three locations (indoor, outdoor, and personal) during the 2005 monitoring. During 2006, PM10 was measured only indoors and outdoors using the PEM; the personal PM10 inlet was replaced by an active pDR as described above. Technicians ensured that the PM2.5 and PM10 tar get flow rates were 4 L/min with an acceptable range of ±20%. Flow rates were assessed before and after sampling every 24 hr using a soap bubble flow meter (AP Buck).
In addition to the PEM samplers, there were two low-flow particulate samplers operating at 0.8 L/min. One collected EC/organic carbon (OC) onto a prefired quartz-fiber 15-mm filter (Pall-Gelman) and the other collected particulate-associated nitrate through a denuder, which scrubbed gas-phase nitrates before collecting the particulates on precoated 15-mm glass-fiber filters (Pall Gelman). The EC/OC filter analysis has yet to be completed and will not be included in this paper. The nitrate denuders were unreliable in 2005 and part of the winter 2006 because of problems with field laboratory protocols; therefore, only the acceptable duplicate data are included in this paper.
During the winter and summer of 2006, a prefired quartz 37-mm fiber filter (Pall-Gelman) was placed in line after the pDR to collect the preseparated particulates (PM2.5) that passed through the PEM inlet and the pDR unit. This filter was in place for all 5 days of each season at a flow rate of 1.8 L/min. During summer 2006, the same filter type was also located indoors for the same 5-day period. The filters will be analyzed for traffic markers (hopanes) in an attempt to understand longer-term exposure to traffic-related air pollutants. Analysis of these filters is still pending, so details are not included in this paper.
Settled dust was sampled by technicians using the high-volume surface sampling system (HVS3) vacuum.Citation35 Samples were typically collected during visits scheduled 1 week after the conclusion of winter monitoring to ensure that the request not to vacuum for a week before the settled dust collection did not interfere with the air pollution monitoring. The sampling required a minimum of 2 m2 of the floor in the living area to be vacuumed for a 4-min period. Technicians were asked to ensure the collection of at least 1 g of dust. A small weighing scale was used to pre- and postweigh the amber glass bottle used to collect the sample in the field. In the event that 1 g of dust could not be collected from the 2 m2 (e.g., on hardwood floors), further living space was sampled to ensure sufficient sample for all intended analyses, with technicians noting any increase in sample area. Samples were stored in amber glass jars and a brown paper bag for shipping.
On receipt of the samples, the dust was sieved before extraction with a nonmetallic 300-μm sieve to remove any coarse material. Dust samples were further sieved into 300- to 150-μm and <150-μm size fractions and recombined for analysis of house dust mite allergen (DerP1 and DerF1), cat allergen (Feld1), endotoxin, and (1 →3)- β-D-glucan.
Allergens were assayed by monoclonal enzyme-linked immunosorbent assay. Endotoxin analyses were completed in accordance with the 1996 version of the American Industrial Hygiene Association protocol described in the Field Guide for the Determination of Biological Contaminants in Environmental Samples. (1 →3)-βD-glucan was analyzed using the limulus ameobocyte lysate-based method.
Metals in airborne PM and in the settled dust were determined using inductively coupled plasma (ICP)–mass spectrometry.Citation36–38
Fixed-Site Monitors
Environment Canada maintains two outdoor National Air Pollution Surveillance (NAPS) sites in the city of Windsor. These sites monitor various pollutants, including PM and criteria gases such as NO2, O3, and VOCs. The College Road East site, located to be representative of urban ambient air pollution found in Windsor, was used for conducting most of the WOEAS duplicate sampler deployment and instrument comparisons to assess bias and precision. Duplicate 24-hr samples for all WOEAS active and passive samplers, excluding the continuous instruments, were collocated at this NAPS site in the summer of 2005 and 2006 and in the winter of 2006. The site was being refurbished during winter 2005 and was therefore not available for that sampling season.
The Environment Canada method for measuring continuous PM2.5 at the Windsor NAPS sites was a tapered element oscillating microbalance (TEOM; Thermo Fisher Scientific) with an inlet heater temperature of 40 °C using the sample equilibrium system (SES) sample dryer. The SES dryer contains specially designed Nafion tubing inlets on the main flow to minimize potential for particle loss. The dryer lowers the RH in the main flow and allows for mass transducer operation at 5 °C above the peak air monitoring station temperature. NO2 was measured by Environment Canada using the chemiluminescence method (Thermo model 42 NO2 analyzers, Thermo Electron Instruments, Inc. [TEI]). O3 was measured by Environment Canada using the ultraviolet (UV) photometric method (Thermo model 49C, TEI). There were no Environment Canada NAPS instruments available to measure UFPs, PM10, or EC. All NAPS instruments operated on a continuous basis and time-averaged values were calculated to correspond directly with the WOEAS instrument measurements.
The WOEAS duplicate passive and active samplers included the Chempass PM system and the Ogawa badges. These were set up by technicians at the Windsor NAPS site and timed to correspond with the rest of the study's personal sampling periods, typically 4:00 p.m. to 4:00 p.m.Citation39 Duplicate indoor and personal samples were also deployed for PM10 for five 24-hr periods in winter 2006.Citation38 Duplicate personal samples were collected by technicians who were located in Windsor and replicated typical participant activities.
The WOEAS Chempass filter-based particulate samples were also collected at the Michigan Department of Environmental Quality (MDEQ) site at Allen Park, Detroit, MI. These measurements were undertaken to ensure the comparability of WOEAS and DEARS methods and to enable comparison of WOEAS particulate samplers with a dichotomous sampler located at this site.Citation18 All samples were collected for 24 hr from 9:00 a.m. to 9:00 a.m. for 2 days per week on Tuesday and Wednesday. Data from this location were used to examine possible bias between the PEM used in this study and the dichotomous sampler measuring PM2.5 and PM10- PM2.5, which is an equivalent Federal Reference Method (FRM). This represents the only comparison of the PEM used in this study with a FRM. The filters used in the FRM dichotomous sampler underwent gravimetric analysis using a Mettler UT20 balance. The filters were placed in Petri dishes in a controlled environmental chamber for a minimum of 24 hr to allow the filters to equilibrate. The temperature remained between 19 and 23 °C ± 2 °C) and the humidity was kept between 30 and 40% ±5%) for a minimum of 24 hr. The balance was warmed up for a minimum of 1 hr before use. After the internal balance calibration procedure, a manual audit of the balance was performed using a 100-mg mass (American Society for Testing and Materials Class 1 or National Bureau of Standards Class P weight). The balance was viewed as operational if the audit indicated the result was within 10 μg of the expected value. In addition, a laboratory reference filter was then analyzed. The reference filter mass had to be within 20 μg of the expected value. At the end of every five measurements, the precision for the balance had to be less than 1 μg, and the precision test was repeated until this was achieved. Further tests involved analyzing a laboratory filter blank for long- and short-term drift of filter weights. After the analysis of every 25th filter, a random re-weigh of at least one filter was performed. The original and re-weigh values had to differ by less than ±]6 μg of the original weight. If not, all of the 25 filters were re-weighed until the required precision was achieved.
Comparison of the personal monitors to a reference method is a crucial requirement in determining the reliability of the data. Two regression methods were used to compare the samplers. Ordinary least-squares (OLS) regression minimizes the vertical distance between the data points and the line of best fit. This type of regression is sometimes said to assume no error in the x-axis measurements; however, it is also the best estimate of the y-axis values given the x-coordinate although the x-coordinate is in error.Citation40 Orthogonal regression recognizes that error may occur in both measurement methods; therefore, it minimizes the perpendicular distance to the line of best fit. However, this approach assumes equal variance in the two measurement methods, which is seldom the case. Reduced major axis (RMA) regression is a form of weighted orthogonal regression in which the ratio of the variances is used to modify the angle between a data point and the line of best fit. The RMA method results is the best estimate of the “true” relationship between the two methods. It has been recommended as the best way to determine whether candidate methods can be certified as reference methods for environmental measurements.Citation41 Therefore, OLS and RMA regression methods were carried out on the PEM2.5 and PEM10 monitors versus the dichotomous sampler.
AERs
A daily assessment of air exchange was undertaken in each of the homes using perfluorocarbon tracer (PFT) gas.Citation42 Four sources of the tracer gas were set up on the main floor of the home at the beginning of the first day to allow the gas to equilibrate for 2 hr during equipment setup. The emitters were deployed for the duration of the 5-day sampling period in each season. One receptor (capillary adsorption tube [CAT]) was installed daily at a location away from any potential ventilation or heating sources. The total amount of the tracer gas absorbed by the receptors combined with the square footage of each home as determined from property tax records and the emission rate of the emitters was used to calculate a daily average AER for each home.
Questionnaires and Time Activity Diaries
Adult participants in 2005 and the parents of asthmatic children in 2006 completed two different questionnaires to assess potential sources of exposure: (1) a baseline questionnaire to obtain information on housing characteristics that did not change over time, and (2) a daily questionnaire to obtain information on daily activities such as cooking and cleaning. During the second season, the recruitment technician administered a shorter baseline survey to assess any new renovations and changes undertaken in the residence since the last season of sampling. In both years participants completed a time activity diary throughout the day, noting their activities and their presence in various locations in 15- and 30-min intervals for the adults and children, respectively. The adult diary included details on whether the individual cooked or cleaned (this was deemed less likely for the children and was removed from their diaries). Both diaries included information on whether the participants were in close proximity to any smokers and for how long because this was deemed important in terms of increased exposure to air pollutants. Key locations noted on the diaries included at home, outside at home, in transit, at work/school, outside away from home, and inside away from home. Multiple responses could be included if activities or locations changed within the time interval. The activities were then coded for all diaries and coding was confirmed by manually assessing 10% of all diaries to ensure consistency in interpretation of the descriptions. All surveys and diaries were independently entered twice and compared electronically to each other to identify any discrepancies in the data entry.
Health Measurements
During the 2006 sampling period, asthmatic children also conducted respiratory health assessments, including FEV1, which was estimated using a PiKo-1 electronic peak expiratory flow/FEV1 meter (Ferraris Medical). These instruments have comparable responsiveness with pneumotachographs.Citation43 Twice daily (first thing in the morning, again at bedtime), three consecutive FEV measurements were made before taking any asthma medications. During the technician visit in the evenings, an exhaled breath condensate sample was collected using an RTube (Respiratory Research, Inc.). Participants sat and orally breathed at tidal volumes into a mouthpiece attached to a cold condenser for 10 min. Approximately 1 mL of breath condensate was collected. The condensed breath was then transferred to several microtubes and stored at −20 °C and then −80 °C until analyses. The sample was first analyzed for amylase to test for saliva contamination. This was followed by the measurement of the oxidative stress bio-markers thiobarbituric acid reactive substances and 8-isoprostane and the inflammatory cytokine interleukin-6. All laboratory methods are described in detail in Liu et al.Citation4 Children's time activity diaries included space to report any symptoms, including cough, wheeze, chest tightness, and difficulty breathing. These symptoms were scored from 0 to 4 where 0 indicated no symptom and 4 indicated the worst symptoms. Scoring was explained to the participants at the start of the study, and technicians reviewed the diaries each day to assess completeness.
Quality Assurance
LDLs were estimated as 3 times the SD of the laboratory blanks, with field detection limits (FDLs) being defined as 3 times the SD of the field blanks. Field blanks comprised approximately 10% of all samples. Duplicate outdoor samples were collected at the NAPS sites.
The quality assurance program included the calibration of flow rates, leak tests, collection of routine field blanks, and determination of precision and accuracy during sampling as well as for the chemical analyses. Various quality control samples were used to determine accuracy and precision of the chemical analyses and to diagnose any sources of contamination.Citation36,Citation38
Blank corrections were applied when more than 50% of the field blanks were greater than the LDL. In these situations, a FDL was then calculated as being 3 times the SD of the field blanks. Sample data were then adjusted by subtracting the median of the field blanks. Any resulting values that were lower than the LDL were substituted with 1½2LDL. Samples that were above the LDL but below the FDLs were not changed.
All data were assessed for validity using the following criteria. Any samples requiring a specific flow rate were tested at the beginning and end of each 24-hr sampling period; if the end flow rate was operating at a flow more than 20% above or below the target flow rate, they were deemed invalid. Samples were also deemed invalid if they were deployed for more than 30 or less than 18 hr. Other criteria for invalidating samples included presence of insects found during assembly, evidence that filters were mishandled in the field or laboratory, or noted sources of contamination in the field or the laboratory.
Duplicate comparisons for all methods, where these data exist, were used to assess precision estimates. Data from standardized methods used at the NAPS and MDEQ were used to yield estimates of bias.
The following bias definition, also frequently referred to as the fractional or percent difference, was utilized:
A precision calculation of two identical instruments is often defined as the absolute value (Abs) of the difference between one instrument reading and the mean of the two, divided by that mean, which works out to be the difference divided by the sum:
The idea in this definition is that when the true value is unknown, it can be assumed that it is near the average of the two instruments.
In many cases in this study, it was not just the comparison of two instruments, but rather several, because all continuous instruments underwentbefore and after side-by-side intercomparisons. It was determined that a reasonable approach would be to compare the continuous instruments to the median of their readings for any given 3-min sampling period. In this case, the median was assumed to be close to the “true” reading. The resulting formula for the bias-corrected precision is
For each pollutant, the laboratory results were combined with log-sheet data to calculate concentrations. Several coding flags were included to address any field or sample-specific issues that arose during the sample collection: Each sample was coded as being valid, flagged, or invalid. All analyses were conducted using SAS version 9.1 (SAS Institute, Inc.).
RESULTS
Participant Retention Rates
Forty-eight adults were originally recruited for the winter and summer 2005 sampling sessions. However, because five participants withdrew from the study after the winter session because of moving, renovating homes, or summer travel plans, two new additional participants were recruited for the summer. Therefore, the total sample size was 48 and 45, in winter and summer, respectively, with 43 of the same homes participating in both seasons. There were 5 male and 45 female adult participants in total.
During the winter and summer of 2006, the total sample size for the asthmatic children was 48 and 48, respectively, with 45 individuals participating in both seasons. In total, 51 asthmatic children were recruited for this study with one winter and two summer sets of siblings being followed. The children were between 10 and 13 yr of age with most (n = 31) being male. This age range was selected because children of this age are able to participate in personal monitoring and complete time activity diaries with minimal supervision.
A total of 922 participant days were completed. This was only 4% less than the intended 960 days, and was due to the small number of participants who were unavailable in the second season. The compliance for the data collection, including wearing the backpack and completing the diaries, was high. Participants reported to the technicians daily and highlighted any noncompliance due to restrictive activities.
NO2
The mean WOEAS NO2 level over 2 yr of sampling at the central NAPS site was 20 ppb for the Ogawa badges, compared with 16 ppb for the collocated Environment Canada chemiluminescence method (). The Ogawa badges had a median bias compared with the NAPS method of 17% and a median precision of 7%.
Table 2. Relative bias and precision of collocated samplers at NAPS
O3
O3 sampling was only undertaken during the summer periods. The mean value for both years was 36 ppb for the Environment Canada UV photometric method compared with 26 ppb for the WOEAS O3 collocated at the NAPS site (), resulting in a median bias of −24%. The Ogawa O3 badges had a median precision of 9%.
VOCs
VOC sampler deployment was intended to be 1395 samplers in 2005 (total number of indoor, outdoor, and personal samplers for all participants) and 930 samplers in 2006 (total number of indoor and outdoor samplers for all participants). However, the few participant retention issues described previously resulted in slightly lower numbers of deployed canisters. In addition, a few deployed VOC canisters were deemed invalid and therefore excluded from the analysis because of flow gauge failures, which was determined as a canister sampling time of less than 18 hr and/or the canister not being collected within 30 hr of deployment. As a result, the total sample size for VOC canisters was 1294 in 2005 and 872 in 2006. A Health Canada report including the full descriptive statistics for the 2005 and 2006 VOC data is available upon request.Citation44
Continuous Instruments
Results from the continuous instruments are presented in a companion paper.Citation29 Briefly, for both years and both seasons there were 902 and 834 person days of indoor and outdoor data, respectively, collected for the Dust-Traks, 656 and 657 person days for the Aethalometers, 666 and 659 person days for the PTraks, and during 2006 personal pDR data resulted in 358 person days of data. The DustTrak and pDR had positive biases of a factor of approximately 2.5 and 1.6, respectively, compared with the PEM. However, their average bias-corrected precisions were within 10%, indicating that a proper correction for bias would bring them into very good agreement with standard methods. Both instruments had LODs of approximately 5 μg/m3. Although no standard methods exist to establish the bias of the Aethalometer and PTrak, their precision estimates were within 20% for the Aethalometer and within 10% for the PTrak.
Chempass PM2.5 and PM10 PEM
Allen Park Site
Intercomparisons between the two different size fractions of the PEM PM2.5 and PM10 with the FRM dichotomous sampler were conducted over the four seasons. Because of missing data in either of the two size fractions of the FRM dichotomous sampler, a total of 38 days were available for comparison of both methods. The slopes and intercepts of the two lines of best fit are provided in , a and b. As can be seen, because of the excellent agreement of both PEMs with the two dichotomous fractions, the OLS and RMA lines virtually overlap. The PEM PM2.5 showed very good agreement (R2 = 0.98) with the collocated Allen Park FRM dichotomous sampler (, ), although with a small positive median bias of 9%. The bias was significant (95% confidence interval 1.07–1.15), and only 3 of the 38 paired samples were below the 1:1 line. The PM2.5 PEM showed good precision (median 7%). The PM10 PEM sampler also showed excellent agreement (R2 = 0.97) with the reference dichotomous sampler, with a median positive bias of 9% and a median precision of 6% (, ). The PEM estimate of coarse particle concentrations (PM10- PM2.5) agreed well with the dichotomous sampler, with a small positive bias of 9% and a slightly worse median precision of 12% ().
Table 3. Comparison of gravimetric PEM to dichotomous reference sampler at Allen Park location: Bias and precision
Figure 3. (a) Comparison of the PEM2.5 sampler with the Allen Park dichotomous sampler. (b) Comparison of the PEM10 sampler with the Allen Park Dichotomous sampler.
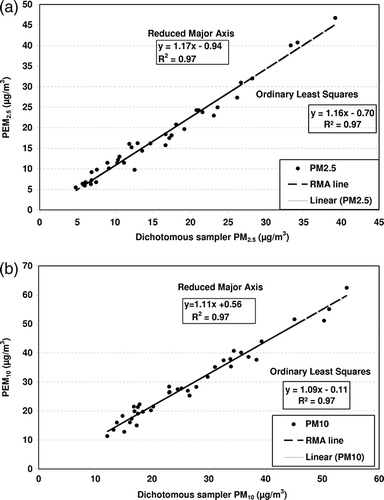
Details of the method evaluation for metals in airborne PM in WOEAS have been described previously and outline quality assurance procedures during sampling, handling, and analysis; analytical method comparisons; and collocated duplicates.Citation36,Citation37
NAPS Site
On the basis of its excellent performance in comparison with the FRM dichotomous sampler, the decision was made to use the PEM as the standard method and then compare it with the TEOM at the Windsor NAPS site. As found in other studies,Citation45 the TEOM displayed strong losses in the one winter season with collocated data having a median negative bias of 51% (). The summer median bias was still negative but less so at -26%.
Table 4. Comparison of gravimetric PM2.5 PEM with collocated TEOM at NAPS location: TEOM bias
Particulate-Associated Nitrate
The mini-PEM samplers for measuring particulate-associated nitrate required the coating of a denuder each time the samplers were used. Because of field laboratory problems, the consistency of the coating was unreliable and all data from 2005 and part of winter 2006 had to be discarded. The median bias for the NAPS-based WOEAS duplicate samplers was 7%. There was no reference method available at the NAPS site for bias calculations.
Settled Dust
A single dust sample was collected from each of the residences in 2005 and 2006, resulting in a total of 93 samples. Samples from the separated settled dust of grain size less than 300 μm were analyzed for dust mite allergens DerP1 and DerF1, cat allergen Feld1, endotoxin, and (133)-D-glucan. For all of these analyses, only the DerP1 samples were found to have most of the samples below the LDL, 35 and 23 of all of the samples collected in 2005 and 2006, respectively, and as such, the replacement of 1½2LDL was not applied. For the remainder of the allergen analyses, 1½2LDL was applied when any were below the LDL.
AER
The average daily AER for each residence was calculated using the calculated house volume and the known amount of PFT that was emitted and trapped on the corresponding daily CAT. A total of 30 and 45 homes in the winter and summer, respectively, of 2005 and 33 and 46 homes in winter and summer, respectively, of 2006 had valid daily AER calculations. Homes missed in the winter season of 2005 and 2006 were because of difficulties in financial contracting that resulted in the late start of the AER sampling.
Questionnaire and Time Activity Diary
Most homes were detached, single-family dwellings (n = 92) with electric stoves (n = 77). Approximately half of the homes had an attached garage. Summary statistics are provided in .
Table 5. Descriptive statistics of the study population by year
Data obtained via the daily questionnaire on activities that occurred in the residence included information on daily cooking, cleaning, presence of smokers, ventilation use, and candle use. Of all of the homes included in the study, only four homes had a smoker present at any point in the study, 257 sampling days had open windows, and on 55 of the sampling days candles were used.
The adults' activity patterns did not alter significantly between winter and summer; therefore, both seasons were combined in . Adults spent approximately 80% of their time indoors at home or indoors away from home, 10% of their time at work, 5.5% of their time in transit, and approximately 4.5% of their time outdoors. The children spent significantly more time indoors at home in the summer than in the winter (77.4% versus 68.7%) and indoors away from home (9.8% versus 5.8%). In winter, the children spent significantly more time at school (18.5%) compared with summer (0.6%). These children were also found to spend significantly more time outdoors in the summer than during the winter, likely because of more favorable weather conditions (23.5 °C vs. 0.5 °C mean temperatures in each season). The children spent 4.8% of their time in the yard at home or close to home in the summer compared with only 0.7% in winter. No significant differences in the average time spent in transit were observed (3.7% in winter and 3.4% in summer).
Health Measurements
During 2006, the asthmatic children completed peak flow measures, provided exhaled breath condensate samples, and noted any symptoms in their diaries. The best three FEV1 and forced vital capacity (FVC) trials were used. A total of 422 morning and 425 evening FEV1 measurements were made together with 424 morning and 428 evening FVC measurements. The exhaled breath condensate sample was only completed each evening with technician supervision, resulting in 458 samples.
The children used the time activity diary to record symptom prevalence, and this indicated that only a small percentage of children reported any cough (2.6%), wheeze(1.6%), chest tightness (1.7%), or difficulty breathing (1.3%) at any point during the 10 days of sampling.
DISCUSSION
There are several personal exposure studies that have included healthy adults and asthmatic children, and of these studies the EPA DEARS conducted at the same time as WOEAS is the most comparable in terms of the adult population and the methods used.Citation18 The main difference in the study designs was that DEARS included a randomly selected population. The WOEAS 2006 asthmatic children represent a susceptible population with a similar age group to other personal monitoring studies that have been conducted.Citation28,Citation46,Citation47 The sample size for the 2006 WOEAS health measurements was determined using a power calculation that was based on results obtained from Delfino et al.Citation28 The Delfino et al.Citation28 study had a population of 19 participants that completed a total of 710 FEV1 maneuvers; this is comparable with the Windsor results in which there were a total of 847 FEV1 maneuvers available for analyses. Children in the age range of 10–13 yr have been shown to be capable of carrying out study activities and complying with study requirements. Retention of participants in both years was high (43 of 48 in 2005 and 2006 completed two seasons of data collection); the small numbers of losses were due to home renovations and scheduling, with no losses attributed to study fatigue. Spatial representativeness was more difficult to ensure seasonally because of participants' scheduling requirements.
The Ogawa NO2 badge median precision of 7% in this study is slightly higher than the findings by Mukherjee et al.,Citation48 who reported that eight paired duplicate samples collected at two different locations (four pairs at each site) over 3, 4, and 7 days had percent relative SD values less than 3.6%. The Quebec City study conducted by Gilbert et al.Citation49 found 7-day Ogawa samples had an average precision of 4.5%.
Similarly, the Ogawa O3 method had a precision of 9%, although it underestimated the concentrations in comparison to the NAPS measurements with a median bias of -24%. Possible reasons for this bias are under investigation. Varns et al.Citation50 also used the Ogawa samplers for O3 monitoring and conducted duplicate analyses as well as an estimate of bias compared with Dasibi model 1008 continuous monitors. Their duplicate data indicated that there was a median absolute difference of 1.38 parts per billion (ppb) and a negative bias that ranged from 2.7 to 4.7 ppb over the four locations used. Typical O3 concentrations were 20–70 ppb in Texas, which is similar to the values found in Windsor. Another paper by Gibson et al.Citation51 showed their duplicate analyses had an overall precision of 5.4%, whereas comparisons at three locations with TEI model 49C continuous O3 analyzers had R2 values ranging from 0.82 to 0.95.
The VOC Summa canisters proved to be an acceptable method of obtaining 24-hr indoor and outdoor residential measurements in both years of sampling. Personal VOC measurements were restricted to the 2005 adult population because the combined weight of the canister, personal pump, and battery was too heavy for the children to carry. The total sample size for VOC canisters was 1294 in 2005 and 872 in 2006, which was 93 and 94% of the intended 1395 and 930 samples, respectively, and represents one of the largest VOC datasets using this method.
The Chempass PM2.5 PEM compared well with the FRM dichotomous sampler over 2 yr of side-by-side measurements at the Allen Park MDEQ site in Detroit. The PEM had an overall bias of +11% compared with the FRM sampler. A regression of the PEM on the FRM showed a small intercept and an R2 of 97%. The positive bias of 11% for the Windsor PEM PM2.5 is an improve ment on the 18% positive bias noted for the Marple PEM2.5 in Ozkaynak et al.Citation15 However, Liu et al.,Citation52 after initially using oiled impactors and noting a large positive bias averaging 7.7 μg/m3, switched to greased impactors and reported a negligible positive bias of 0.4 μg/m3. Williams et al.Citation53 reported that their PEM had a 10–20% higher mass concentration than the FRM, probably as a result of retention of semi-volatile organic compounds (SVOCs) by the PEMs that are blown off by high filter face velocity samplers such as the dichotomous sampler.
Winter and summer comparisons for the study presented here found that the PEM was approximately 52 and 26% higher, respectively, than the TEOM. Differences between the PEM and the TEOM have been previously attributed to evaporation of PM volatiles in the TEOM measurements.Citation45 The TEOM reads lower than other filter-based methods because of its elevated inlet temperature, which causes a proportion of the volatiles in the particulates to be vaporized on intake. This bias will vary depending on the proportion of volatiles in the particulates.Citation54 During the winter, when the temperature difference between ambient air and the filter is greater, there may be greater volatilization than during the summer.Citation45 The DustTrak and pDR PM2.5 agreed well with the gravimetric PEM PM2.5 method, (R2 of 87 and 71%, respectively). These continuous data are important indicators of peak exposures, which can be identified through the location and activity data available from the time activity diaries.
Time activity patterns are similar to those reported in the Canadian Human Activity Pattern SurveyCitation55 and those reported within the United StatesCitation56 and other developed countries.Citation57 Some of the biggest seasonal differences were due to the children being in school during the winter sampling period compared with the summer when school was not taking place; the adult population did not have equivalent differences. Time spent outdoors was also influenced by season and both populations spent more time outdoors in summer ( ∼10% of their total day).
CONCLUSIONS
Most WOEAS data can be used with confidence to examine the relationships among personal, indoor, and outdoor concentrations for the range of pollutants listed. Predictors of these relationships can be determined using the questionnaires and time activity diary data, which were reviewed by the technicians on a daily basis with the participants to ensure accuracy and compliance. The study can also be used to understand the impact that ambient air pollution has on personal and indoor residential exposures. When the data are combined with the health effects data collected in 2006, it will be possible to investigate the effects of personal, indoor, and outdoor air pollution exposures on respiratory health. These WOEAS data can be used with confidence for developing risk management policies to reduce personal and indoor exposures to air pollutants.
ACKNOWLEDGMENTS
The participants and their families are gratefully thanked for their contributions to these 2 yr of data collection; without them, none of this research would have been possible. The careful field work undertaken by the numerous field technicians from Health Canada and the University of Windsor is appreciated. The authors would like to acknowledge contributions from Dr. Paul Villeneuve (Health Canada) for the power calculation; Alice Grgicak-Mannion (Great Lakes Institute for Environmental Research, University of Windsor) for the map of residential locations; Mark Davey (University of Washington), Steve Ferguson, and Dr. Mike Wolfson (Harvard School of Public Health) for their guidance on methods and laboratory analyses; Sandy Benetti of Environment Canada for her help in the initial coordination of the laboratory activities and sample analyses; Don Fugler of the Canadian Mortgage and Housing Corporation and Dr. Russell Dietz of Brookhaven National Laboratory for their support with AER measurements; Environment Canada and MDEQ for permitting the authors to locate instruments at the College Road and Allen Park sites and access to their data for the method comparisons; and Dr. Markey Johnson and Dr. Scott Weichenthal from Health Canada for conducting the internal review. BAQS provided funding through Health Canada. Although this work was reviewed by EPA and approved for publication, it may not necessarily reflect official agency policy.
REFERENCES
- Sarnat , J. , Wilson , W. , Strand , M. , Brook , J. , Wyzga , R. and Lumley , T. 2007 . Panel Discussion review: Session One: Exposure Assessment and Related Errors in Air Pollution Epidemiologic Studies . J. Expo. Sci. Environ. Epidemiol. , 17 : S75 – S82 .
- Sarnat , S.E. , Klein , M. , Sarnat , J.A. , Flanders , W.D. , Waller , L.A. , Mul-holland , J.A. , Russell , A.G. and Tolbert , P.E. 2010 . An Examination of Exposure Measurement Error from Air Pollutant Spatial Variability in Time-Series Studies . J. Expo. Sci. Environ. Epidemiol. , 20 : 135 – 146 .
- Schwartz , J. , Sarnat , J.A. , Coull , B.A. and Wilson , W.E. 2007 . Effects of Exposure Measurement Error on Particle Matter Epidemiology: A Simulation Using Data from a Panel Study in Baltimore, MD . J. Expo. Sci. Environ. Epidemiol. , 17 ( Suppl 2 ) : S2 – S10 .
- Liu , L. , Poon , R. , Chen , L. , Frescura , A. , Montuschi , P. , Ciabattoni , G. , Wheeler , A.J. and Dales , R. 2009 . Acute Effects of Air Pollution on Pulmonary Function, Airway Inflammation, and Oxidative Stress in Asthmatic Children . Environ. Health Perspect. , 117 : 668 – 674 .
- Liu , L. , Ruddy , T. , Dalipaj , M. , Szyszkowicz , M. , You , H. , Poon , R. , Wheeler , A.J. and Dales , R. 2007 . Influence of Personal Exposure to Particulate Air Pollution on Cardiovascular Physiology and Biomarkers of Inflammation and Oxidative Stress in Subjects with Diabetes . J. Occup. Environ. Med. , 49 : 258 – 265 .
- Gauderman , W.J. , McConnell , R. , Gilliland , F. , London , S. , Thomas , D. , Avol , E. , Vora , H. , Berhane , K. , Rappaport , E.B. , Lurmann , F. , Margolis , H.G. and Peters , J. 2000 . Association between Air Pollution and Lung Function Growth in Southern California Children . Am. J. Respir. Crit. Care Med. , 162 : 1383 – 1390 .
- Dales , R. , Wheeler , A.J. , Mahmud , M. , Frescura , A.-M. and Liu , L. 2009 . The Influence of Neighborhood Roadways on Respiratory Symptoms among Elementary Schoolchildren . J. Occup. Environ. Med. , 51 : 654 – 660 .
- Dales , R. , Wheeler , A.J. , Mahmud , M. , Frescura , A.M. , Smith-Doiron , M. , Nethery , E. and Liu , L. 2008 . The Influence of Living near Roadways on Spirometry and Exhaled Nitric Oxide in Elementary Schoolchildren . Environ. Health Perspect. , 116 : 1423 – 1427 .
- Delfino , R.J. , Staimer , N. , Tjoa , T. , Gillen , D. , Kleinman , M.T. and Sioutas , C. 2008 . Personal and Ambient Air Pollution Exposures and Lung Function Decrements in Children with Asthma . Environ. Health Perspect. , 116 : 550 – 558 .
- McConnell , R. , Berhane , K. , Gilliland , F. , Islam , T. , Gauderman , W.J. , London , S.J. , Avol , E. , Rappaport , E.B. , Margolis , H.G. and Peters , J.M. 2002 . Indoor Risk Factors for Asthma in a Prospective Study of Adolescents . Epidemiology , 13 : 288 – 295 .
- Allen , R. , Larson , T. , Sheppard , L. , Wallace , L. and Liu , L.J.S. 2003 . Use of Real-Time Light Scattering Data to Estimate the Contribution of Infiltrated and Indoor-Generated Particles to Indoor Air . Environ. Sci. Technol. , 37 : 3484 – 3492 .
- Sarnat , J.A. , Long , C.M. , Koutrakis , P. , Coull , B.A. , Schwartz , J. and Suh , H.H. 2002 . Using Sulfur as a Tracer of Outdoor Fine Particulate Matter . Environ. Sci. Technol. , 36 : 5305 – 5314 .
- Wallace , L.A. and Williams , R. 2005 . Use of Personal-Indoor-Outdoor Sulfur Concentrations to Estimate the Infiltration Factor and Outdoor Exposure Factor for Individual Homes and Persons . Environ. Sci. Technol. , 39 : 1707 – 1714 .
- Dales , R. , Liu , L. , Wheeler , A.J. and Gilbert , N. 2008 . Quality of Indoor Residential Air and Health . CMAJ , 179 : 147 – 152 .
- Ozkaynak , H. , Xue , J. , Spengler , J. , Wallace , L. , Pellizzari , E. and Jenkins , P. 1996 . Personal Exposure to Airborne Particles and Metals: Results from the Particle TEAM Study in Riverside, California . J. Expo. Anal. Environ. Epidemiol. , 6 : 57 – 78 .
- Sarnat , J.A. , Koutrakis , P. and Suh , H.H. 2000 . Assessing the Relationship between Personal Particulate and Gaseous Exposures of Senior Citizens Living in Baltimore, MD . Journal of the Air & Waste Management Association , 50 : 1184 – 1198 .
- Stocco , C. , Macneill , M. , Wang , D. , Xu , X. , Guay , M. , Brook , J.R. and Wheeler , A J. 2008 . Predicting Personal Exposure of Windsor, Ontario Residents to Volatile Organic Compounds Using Indoor Measurements and Survey Data . Atmos. Environ. , 42 : 5905 – 5912 .
- Williams , R. , Rea , A. , Vette , A. , Croghan , C. , Whitaker , D. , Stevens , C. , McDow , S. , Fortmann , R. , Sheldon , L. , Wilson , H. , Thornburg , J. , Phillips , M. , Lawless , P. , Rodes , C. and Daughtrey , H. 2008 . The Design and Field Implementation of the Detroit Exposure and Aerosol Research Study . J. Expo. Anal. Environ. Epidemiol. , 19 : 643 – 659 .
- McCreanor , J. , Cullinan , P. , Nieuwenhuijsen , M.J. , Stewart-Evans , J. , Malliarou , E. , Jarup , L. , Harrington , R. , Svartengren , M. , Han , I.-K. , Ohman-Strickland , P. , Chung , K.F. and Zhang , J. 2007 . Respiratory Effects of Exposure to Diesel Traffic in Persons with Asthma . N. Engl. J. Med. , 357 : 2348 – 2358 .
- Sabin , L.D. , Behrentz , E. , Winer , A.M. , Jeong , S. , Fitz , D.R. , Pankratz , D.V. , Colome , S.D. and Fruin , S.A. 2005 . Characterizing the Range of Children's Air Pollutant Exposure during School Bus Commutes . J. Expo. Sci. Environ. Epidemiol. , 15 : 377 – 387 .
- Strak , M. , Boogaard , H. , Meliefste , K. , Oldenwening , M. , Zuurbier , M. , Brunekreef , B. and Hoek , G. 2010 . Respiratory Health Effects of Ultrafine and Fine Particle Exposure in Cyclists . Occup. Environ. Med. , 67 : 118 – 124 .
- Rea , A.W. , Zufall , M.J. , Williams , R.W. , Howard-Reed , C. and Sheldon , L. 2001 . The Influence of Human Activity Patterns on Personal PM Exposure: A Comparative Analysis of Filter-Based and Continuous Particle Measurements . Journal of the Air & Waste Management Association , 51 : 1271 – 1279 .
- Sexton , K. , Mongin , S.J. , Adgate , J.L. , Pratt , G.C. , Ramachandran , G. , Stock , T.H. and Morandi , M.T. 2007 . Estimating Volatile Organic Compound Concentrations in Selected Microenvironments Using Time-Activity and Personal Exposure Data . J. Toxicol. Environ. Health , 70 : 465 – 476 .
- Diamond , G. and Parker , M. 2004 . Preliminary Air Quality Assessment Related to Traffic Congestion at Windsor's Ambassador Bridge , Toronto, Ontario , , Canada : Ontario Ministry of Environment .
- Particulate Matter; Ontario Ministry of Environment http://www.ene.gov.on.ca/en/air/info/pm.php (http://www.ene.gov.on.ca/en/air/info/pm.php) (Accessed: 2 January 2007 ).
- Killip , S. , Mahfoud , Z. and Pearce , K. 2004 . What Is an Intracluster Correlation Coefficient? Crucial Concepts for Primary Care Researchers . Ann. Fam. Med. , 2 : 204 – 208 .
- Sette , L. , Del Col , G. , Comis , A. , Milic-Emili , J. , Rossi , A. and Boner , A.L. 1996 . Effect of Pattern of Preceding Inspiration on FEV1 in Asthmatic Children . Eur. Respir. J. , 9 : 1902 – 1906 .
- Delfino , R.J. , Quintana , P.J.E. , Floro , J. , Gastanaga , V.M. , Samini , B.S. , Kleinman , M.T. , Liu , L.-J.S.L. , Bufalino , C. , Wu , C.-F. and McLaren , C.E. 2004 . Association of FEV1 in Asthmatic Children with Personal and Microenvironmental Exposure to Airborne Particulate Matter . Environ. Health Perspect. , 8 : 932 – 941 .
- Wallace, L.; Wheeler, A.J.; Kearney, J.; Van Ryswyk, K.; You, H.; Kulka, R.; Rasmussen, P.; Brook, J.R.; Xu, X. Validation of Continuous Particle Monitors for Personal, Indoor, and Outdoor Exposures; J. Expo. Anal. Environ. Epidemiol. 2010 http://www.nature.com/jes/journal/vaop/ncurrent/index.html#05052010 (http://www.nature.com/jes/journal/vaop/ncurrent/index.html#05052010) (Accessed: 2010 ).
- Wallace , L.A. and Howard-Reed , C.H. 2002 . Continuous Monitoring of Ultra-fine, Fine, and Coarse Particles in a Residence for 18 Months in 1999–2000 . Journal of the Air & Waste Management Association , 52 : 828 – 844 .
- Demokritou , P. , Kavouras , I. , Ferguson , S. and Koutrakis , P. 2001 . Development and Laboratory Performance Evaluation of a Multipollutant Sampler for Simultaneous Measurements for Particulate and Gaseous Pollutants . Aerosol Sci. Technol. , 35 : 741 – 752 .
- 1998 . Quality Assurance Guidance Document 2.12: Monitoring PM2.5 in Ambient Air Using Designated Reference or Class 1 Equivalent Methods , Research Triangle Park , NC : U.S. Environmental Protection Agency; Human Exposure and Atmospheric Sciences Division .
- Rasmussen , P.E. , MacIntyre , D.J. and Guenette , J. Buoyancy-Corrected Gravimetric Analysis System . U.S. Patent 7357045 . 2008 .
- Rasmussen , P.E. , Gardner , H.D. and Niu , J. 2010 . Buoyancy Corrected Gravimetric Analysis of Lightly Loaded Filters . Journal of the Air & Waste Management Association , 60 : 1065 – 1077 . doi: 10.3155/1047-3289.60.9.1065
- Development of a High Volume Surface Sampler for Pesticides in Floor Dust . Report No. 600/4-89/036 . U.S. Environmental Protection Agency; National Exposure Research Laboratory: Research Triangle Park, NC, 1989
- Rasmussen , P.E. , Wheeler , A.J. , Hassan , N.M. , Filiatreault , A. and Lanouette , M. 2007 . Monitoring Personal and Residential Exposures to Metals in Airborne Particulate Matter: Risks of Contamination during Sampling, Handling and Analysis . Atmos. Environ. , 41 : 5897 – 5907 .
- Rasmussen , P.E. , Niu , J. , Chénier , M. , Wheeler , A. , Nugent , M. and Gardner , H.D. Refined Analysis and Characterization Methods for Metals in Urban Residential Air . Proceedings of the Metals in the Human Environment (NSERC MITHE-SN) Annual Symposium . January 20–21 , Aylmer , Quebec , Canada.
- Niu , J. , Rasmussen , P.E. , Wheeler , A.J. , Williams , R. and Chénier , M. 2010 . Evaluation of Airborne Particulate Matter and Metals Data in Personal, Indoor and Outdoor Environments Using ED-XRF and ICP-MS and Collocated Duplicate Samples . Atmos. Environ. , 44 : 235 – 245 .
- Kulka , R. , Stocco , C. , Kearney , J. , Van-Ryswyk , K. , Van-Rijswijk , D. , Bellack , N. , You , H. , Xu , X. , Brook , J. , Rasmussen , P. and Wheeler , A.J. An Analysis of PM2.5 Sampler Intercomparisons Performed in Exposure Assessment Studies by Health Canada . Presented at the Air & Waste Management Association Conference . Chapel Hill , NC .
- Draper , N.R. and Smith , H. 1981 . Applied Regression Analysis , 2nd , New York : Wiley .
- Ayers , G.P. 2001 . Comment on Regression Analysis of Air Quality Data . Atmos. Environ. , 35 : 2423 – 2425 .
- Dietz , R.N. and Cote , E.A. 1982 . Air Infiltration Measurements in a Home Using a Convenient Perfluorocarbon Tracer Technique . Environ. Int. , 8 : 419 – 433 .
- Rodríguez-Pascual , L. , Cordero-Guevara , J. and Viejo-Bañuelosa , J.L. 2006 . Agreement between Pneumotachograph and PiKo-1 Measurements of PEF and FEV1 . Arch Bronconeumol. , 42 : 144 – 147 .
- Windsor Ontario Exposure Assessment Study: VOC Sampling Data Summary (2005, 2006) , Ottawa, Ontario , , Canada : Draft Report; Health Canada .
- Allen , G. , Sioutas , C. , Koutrakis , P. , Reiss , R. , Lurmann , F.W. and Roberts , P.T. 1997 . Evaluation of the TEOM Method for Measurement of Ambient Particulate Mass in Urban Areas . Journal of the Air & Waste Management Association , 47 : 682 – 689 .
- Liu , L.J. , Box , M. , Kalman , D. , Kaufman , J. , Koenig , J. , Larson , T. , Lumley , T. , Sheppard , L. and Wallace , L. 2003 . Exposure Assessment of Particulate Matter for Susceptible Populations in Seattle . Environ. Health Perspect. , 111 : 909 – 918 .
- Janssen , N.A.H. , Hoek , G. , Harssema , H. and Brunekreef , B. 1997 . Childhood Exposure to PM10: Relation between Personal, Classroom and Outdoor Concentrations . Occup. Environ. Med. , 54 : 888 – 894 .
- Mukerjee , S. , Smith , L. , Norris , G. , Morandi , M. , Gonzales , M. , Noble , C. , Neas , L. and Ozkaynak , H. 2004 . Field Method Comparison between Passive Air Samplers and Continuous Monitors for VOCs and NO2 in El Paso, Texas . Journal of the Air & Waste Management Association , 54 : 307 – 319 .
- Gilbert , N.L. , Gauvin , D. , Guay , M. , Heroux , M.E. , Dupuis , G. , Legris , M. , Chan , C.C. , Dietz , R.N. and Levesque , B. 2006 . Housing Characteristics and Indoor Concentrations of Nitrogen Dioxide and Formaldehyde in Quebec City . J. Can. Environ. Res. , 102 : 1 – 8 .
- Varns , J. , Mulik , J. , Sather , M. , Glen , G. , Smith , L. and Stallings , C. 2001 . Passive Ozone Network of Dallas: A Modeling Opportunity with Community Involvement . Environ. Sci. Technol. , 35 : 845 – 855 .
- Gibson , M.D. , Guernsey , J.R. , Beauchamp , S. , Waugh , D. , Heal , M.R. , Brook , J.R. , Maher , R. , Gagnon , G.A. , McPherson , J.P. , Bryden , B. , Gould , R. and Terashima , M. 2009 . Quantifying the Spatial and Temporal Variation of Ground-Level Ozone in the Rural Annapolis Valley, Nova Scotia, Canada Using Nitrite-Impregnated Passive Samplers . Journal of the Air & Waste Management Association , 59 : 310 – 320 . doi: 10.3155/1047-3289.59.3.310
- Liu , L.-J.S. , Box , M. , Kalman , D. , Kaufman , J. , Koenig , J. , Larson , T. , Lumley , T. , Sheppard , L. and Wallace , L. 2003 . Exposure Assessment of Particulate Matter for Susceptible Populations in Seattle, WA . Environ. Health Perspect. , 111 : 909 – 918 .
- Williams , R. , Suggs , J. , Zweidinger , R. , Evans , G. , Creason , J. , Kwok , R. , Rodes , C. , Lawless , P. and Sheldon , L. 2000 . The 1998 Baltimore Particulate Matter Epidemiology Exposure Study: Part 1. Comparison of Ambient, Residential Outdoor, Indoor and Apartment Particulate Matter Monitoring . J. Expo. Anal. Environ. Epidemiol. , 10 : 518 – 532 .
- Meyer , M.B. , Lijek , J. and Ono , D. 1992 . “ Continuous PM10 Measurements in a Woodsmoke Environment ” . In Proceedings of the 1992 PM10 Standards and Nontraditional Particulate Source Control , 24 – 38 . Pittsburgh , PA : A&WMA .
- Leech , J.A. , Nelson , W.C. , Burnett , R.T. , Aaron , S. and Raizenne , M.E. 2002 . It's about Time: A Comparison of Canadian and American Time-Activity Patterns . J. Expo. Anal. Environ. Epidemiol. , 12 : 427 – 432 .
- Klepeis , N.E. , Nelson , W.C. , Ott , W.R. , Robinson , J.P. , Tsang , A.M. , Switzer , P. , Behar , J.V. , Hern , S.C. and Engelmann , W.H. 2001 . The National Human Activity Pattern Survey (NHAPS): A Resource for Assessing Exposure to Environmental Pollutants . J. Expo. Anal. Environ. Epidemiol. , 11 : 231 – 252 .
- Schweizer , C. , Edwards , R.D. , Bayer-Oglesby , L. , Gauderman , W.J. , Ilacqua , V. , Jantunen , M.J. , Lai , H.K. , Nieuwenhuijsen , M. and Kunzli , N. 2007 . Indoor Time-Microenvironment-Activity Patterns in Seven Regions of Europe . J. Expo. Sci. Environ. Epidemiol. , 17 : 170 – 181 .