ABSTRACT
The influence of sea-land breezes (SLBs) on the spatial distribution and temporal variation of particulate matter (PM) in the atmosphere was investigated over coastal Taiwan. PM was simultaneously sampled at inland and offshore locations during three intensive sampling periods. The intensive PM sampling protocol was continuously conducted over a 48-hr period. During this time, PM2.5 and PM2.5–10 (PM with aerodynamic diameters ≤2.5 μm and between 2.5 and 10 μm, respectively) were simultaneously measured with dichotomous samplers at four sites (two inland and two offshore sites) and PM10 (PM with aerodynamic diameters ≤10 μm) was measured with β-ray monitors at these same 4 sites and at 10 sites of the Taiwan Air Quality Monitoring Network. PM sampling on a mobile air quality monitoring boat was further conducted along the coastline to collect offshore PM using a β-ray monitor and a dichotomous sampler. Data obtained from the inland sites (n = 12) and offshore sites (n = 2) were applied to plot the PM10 concentration contour using Surfer software. This study also used a three-dimensional meteorological model (Pennsylvania State University/National Center for Atmospheric Research Meteorological Model 5) and the Comprehensive Air Quality Model with Extensions to simulate surface wind fields and spatial distribution of PM10 over the coastal region during the intensive sampling periods. Spatial distribution of PM10 concentration was further used in investigating the influence of SLBs on the transport of PM10 over the coastal region. Field measurement and model simulation results showed that PM10 was trans ported back and forth across the coastline. In particular, a high PM10 concentration was observed at the inland sites during the day because of sea breezes, whereas a high PM10 concentration was detected offshore at night because of land breezes. This study revealed that the accumulation of PM in the near-ocean region because of SLBs influenced the tempospatial distribution of PM10 over the coastal region.
Model simulation and field measurements show that PM was transported back and forth across the coastline because of SLBs and that this regularly influenced the tempospatial distribution of PM10 over the coastal region. A high PM10 concentration was observed at the inland sites because of daytime onshore breezes, whereas a high PM10concentration was detected over the ocean because of nighttime offshore breezes. The implications of these results suggest that relevant authorities should work to prevent pollution episodes by reducing regional PM emissions that lead to stagnant or recirculating air under specific weather conditions.
INTRODUCTION
Sea-land breezes (SLBs) play an important role in transporting air pollutants to and from urban areas on the coast.Citation1,Citation2 Previous studies have investigated the effects of SLBs on the spatial distribution and transport of ambient air pollutants, particularly for ozone episodes.Citation1,Citation3–6 How ever, only a few investigations have focused on the influences of SLBs on atmospheric aerosols. These studies investigated the origin of high PM10 (particulate matter [PM] with aerodynamic diameters ≤10 μm) and total suspended particulate concentrations at rural sites in Spain.Citation7,Citation8 Atmospheric coastal dynamics can exert a significant influence on the levels and composition of atmospheric PM.Citation7 The effects of breeze dynamics and thermal internal boundary layer formation are the main meteorological drivers of the recirculation and hourly evolution of PM levels.Citation8 In the daytime, sea breezes can penetrate deep inland and cause ozone episodes by early afternoon. These sea breezes are capable of transporting air pollutants from coastal urban areas to a distance 20–60 km inland.Citation9–11 Marine aerosols are generally higher in the daytime than at night.Citation1 Land breezes over the Dead Sea during the night bring new PM to the southeastern area of the Dead Sea that is responsible for the formation of a lower haze layer in the early morning.Citation5 In eastern Spain, transport of polluted air masses in the summer is influenced by breeze circulation and the development of an Iberian thermal low.Citation12 Under this scenario, the transport of air pollutants undergoes marked daily cycles. Thus, at coastal sites, the SLBs induce an inland transport of air pollutants during the daytime and a seaward return of air pollutants at nighttime, causing a recirculation of air pollutants back to inland regions each day. Moreover, the polluted air masses undergo vertical recirculation over the eastern coast of Spain because of several factors, including the inland upslope winds over the coastal ranges, the westerly winds over the top of the mountains, subsidence over the Mediterranean Sea, and the inland entry of sea breezes. Thus, air mass circulation is typically associated with high surface ozone concentrations.Citation12 In addition to these regional circulations, synoptic-scale meteorology induces frequent outbreaks of African dust in Spain during the summer.Citation7,Citation13 The influence of sea breeze circulation and primary particulate anthropogenic emissions on the relationship between urban aerosol concentrations has been studied in a coastal city.Citation14–16 The accumulation of air pollutants in the offshore region due to SLBs regularly influences the tempospatial distribution of air pollutants in the coastal region.Citation17 Moreover, according to hourly meteorology and measurement of air pollutant concentrations, the characteristics of air pollution episodes in the Taipei Basin have been shown to be influenced by frontal dust, the northeastern monsoon, southern wind, and SLBs.Citation16
High PM10 and ozone episodes frequently occur over southern Taiwan, a highly industrialized and polluted region.Citation17 Previous studies have investigated the effect of SLBs on the horizontal distribution of air pollutant concentrations in metropolitan Kaohsiung in Taiwan.Citation18 In the autumn, shallow northeasterly winds prevail after frontal passage; these are diverted by the Central Mountain Ridge because of its mean altitude of approximately 2600 m. Numerical results indicate that anthropogenic emissions from north of Kaohsiung could contribute as much as 41% of the ozone over the Kaohsiung metropolitan area and 24% of that over the inland rural area during the northerly flow.Citation19 Once a sea breeze develops, the strong onshore flow transports significant amounts of air mass containing preformed ozone and/or its precursors to the inland rural areas, resulting in the high ozone episodes that frequently occur over southern Taiwan during the autumn season. Numerical and field sampling studies have been undertaken to investigate the air pollution episodes in north, central, southwest, and southern Taiwan.Citation20–27 However, these studies have mainly investigated ozone episodes, their origins, and the influence of SLBs on the tempospatial distribution of ozone. Less research has been conducted on the effects of SLBs on the tempospatial distribution of PM10 during PM episodes in southern Taiwan. Offshore sampling of air pollutants has particularly not been exerted along the coastline of this area. Although previous numerical studies have revealed that air pollutants have a tendency to transport back and forth across the southern coastline,Citation17,Citation19 theaccumulation of air pollutants in the offshore region causing air pollution episodes due to SLBs has not yet been thoroughly investigated through field measurements. This is one gap in the literature that the study presented here seeks to address.
EXPERIMENTAL METHODS
In this study, PM was simultaneously sampled inland and offshore during three intensive sampling periods taking place August 16–18 and November 2–4, 2006, and May 2–4, 2007. The first intensive sampling period was selected to investigate the influence of SLBs on the tempospatial variation of atmospheric aerosols in the summer season. However, the second intensive sampling period was selected to investigate the influences of SLBs and the northeastern monsoon on the spatial and temporal variation of PM during the winter season. The third intensive sampling period was part of the late spring season, during which sea breezes always occur during the daytime. The location of inland and offshore sites for sampling PM around the coastal region of southern Taiwan is shown in .
Inland PM sampling was conducted at 12 sites located in southern Taiwan, including 10 Taiwan Air Quality Monitoring Network (TAQMN) sites and at two others selected for this particular study. The two latter sites for intensive sampling were located at the National Kaohsiung University (NKU) and at Fooyin College (FYC). In these inland sampling sites, two mobile air quality monitoring vehicles were used to simultaneously collect ambient PM (PM2.5 [PM ≤ 2.5 μm], PM2.5–10 [PM between 2.5 and 10 μm], and PM10) with β-ray monitors (Metone, BAM1020) and dichotomous samplers (Anderson, model series 241). Offshore PM sampling was conducted at two offshore locations, including Hsiau-Liu-Chiou (HLC), an island approximately 10 km away from the southwestern coast of Kaohsiung City. In addition, a mobile air quality monitoring boat navigated positions approximately 5–12 km away from the coastline. During each intensive sampling period of 48 hr, offshore PM sampling conducted as inland PM sampling was carried out simultaneously. This was done even in the northeastern monsoon winds because marine PM (PM2.5, PM2.5–10, and PM10) was sampled at the top floor on the front deck on the boat to prevent interference from the exhaust tail gases of the boat and from oceanic spray. Like the inland PM sampling, offshore PM was also sampled using β -ray monitors and dichotomous samplers. The size distribution of atmospheric aerosols was measured with micro-orifice uniform deposit impactors (MOUDIs) at FYC and HLC for inland and offshore sampling sites.
During the intensive sampling periods, PM10 concentrations were measured each hour by β-ray monitors over a 48-hr period, and PM2.5 and PM2.5–10 were sampled by dichotomous samplers at 12-hr intervals (quartz filters were changed at 8:00 a.m. and 8:00 p.m.) at each sampling site. At each β-ray monitoring interval, the mass of ambient particles deposited on the fibrous filter tape was detected by measuring β-ray transmission before and after PM10 sampling.Citation28 The dichotomous sampler was operated with a total flow rate of 16.7 L/min using 37-mm quartz filters (Pallflex 2500 QAT-UP) supported by polyolefin rings. To reduce the carbonaceous species background level in the filter, filters were pre-heated before sampling at 900 °C for 90 min and then placed in clean polyethylene Petri dishes.Citation29 Before and after field sampling, the quartz filters were weighed on an electrical microbalance (Sartorius WMC6014) with a reading precision of 1 μg to determine the mass concentration. These filters were initially conditioned at 25 ± 2 °C and 40% ± 5% relative humidity for 24 hr. After weighing, the filters were stored in a 4 °C environment before chemical analysis to avoid the loss of semi-volatile species. During the intensive sampling periods, the size distribution of atmospheric aerosols was measured with a MOUDI with a flow rate of 30 L/min. This consisted of 10 stages with cutoff diameters ranging from 0.056 to 18 μm, respectively.Citation30 The quartz filters of 47- and 37-mm diameter (for the last stage only) were used as substrate material for a 24-hr sampling starting at 8:00 a.m. The weighing and conditioning procedures were the same as those used for the dichotomous sampler.
A mesoscale three-dimensional (3D) high-resolution meteorological model (Pennsylvania State University/National Center for Atmospheric Research Meteorological Model 5 [MM5]) was used in this study to simulate SLBs and monsoon wind movements and to investigate their effects on the transport of PM over the coastal region. In addition, a backward trajectory model was further applied to plot the transport routes of PM in southern Taiwan. A two-dimensional (2D) spatial plotting software, Surfer, was used to plot the PM10 concentration contour around the coastline using field measurement data obtained from the inland and offshore sites. Moreover, the spatial distribution of PM10 concentration was further simulated by a 3D Eulerian nested grid model, CAMx (Comprehensive Air Quality Model with Extensions). This model has been widely used to simulate the spatial distribution and temporal variation of air pollution episodes in many metropolitan areas around the world.
The MM5 simulations described herein used four grids with spatial resolutions of 27, 9, 3, and 1 km. The coarsest resolution grid (27 × 27 km) covered nearly all of Northeast Asia, whereas the finest grid (1 × 1 km) covered only southern Taiwan. The center of all four grids was located at 22.41591° north latitude, 119.94126° east longitude. The Central Weather Bureau of Taiwan (CWBT) provided the meteorological dataset. Topography and land use distribution was obtained from the database of the Taiwan Geophysical Data Center; the difference between the real orography and the interpolated one for grid 4 was a less than 15–25% reduction in the mean height of the modeling topography. Global analysis data were used for the initialization and nudging of MM5 meteorological fields during each run. To prevent the influence of global analysis data on mesoscale features, several methods were used to initialize grid 4 as follows:
(1) grid 4 was started after grids 1–3, having a 12-hr spin-up period; (2) the first three grids were run separately, and grid 3 output data were used as input to grid 4 via one-way nesting; and (3) in the first step, only grid 1 was run with the input of global analysis data—in the second step, grids 2 and 3 were initialized using the grid 1 output, and in the third step grid 4 was run with input data from grid 3.
The CAMx model has been widely used to simulate the effects of a meteorological field on ambient air quality. The southern Taiwan gridded emission data of PM10 have a resolution of 1 km and were presented using a geographic information system (GIS). This study used the emission inventory for the CAMx model, which is dominated by power plants, steel plants, petrochemical refineries, cement plants, biomass burning, motor vehicles, and fugitive road dusts. These are main contributors to PM, accounting for approximately 85% of the overall PM10 emissions in southern Taiwan. The emission inventory of southern Taiwan supplied by the Taiwan Environmental Protection Administration (TEPA) provided daily basis in the period of August 2006 to May 2007. The modeling for this study is limited to 48-hr intervals in three periods (August 16–18 and November 2–4, 2006; May 2–4, 2007). All CAMx simulations in southern Taiwan were initialized at 8:00 a.m. Chinese Standard Time and conducted over a 48-hr period.
Backward trajectories were simulated with an Air Quality Trajectory Model (version 1.1) developed by TEPA. The assimilation method, which incorporates the Barnes objective method to interpolate spatial values and the variation-kinematical model, was adopted to correct the effects of complex terrain and to produce hourly wind field data using 24 surface stations spread over southern TaiwanCitation31,Citation32; these included two meteorological stations from CWBT and 22 ambient air quality monitoring stations from TAQMN. By utilizing the hourly wind fields over the surface, backward trajectories were simulated for 10 hr at seven selected air quality monitoring stations. The trajectories were constructed using only the horizontal wind components, had a segment resolution of 1 hr, and the interpolation was linear in time and space. The initial time of the backward trajectories for each monitoring station was set when the maximum hourly PM10 concentrations were observed.
RESULTS AND DISCUSSION
Mass Concentration of PM
The concentration of PM2.5, PM2.5–10, and PM10 col lected at four selected sampling sites (two inland and two offshore) in the coastal region of southern Taiwan are summarized in . Ambient PM levels at the inland sites (NKU and FYC) were approximately 1.3–1.6 times higher than those at the offshore sites (offshore boat [OSB] and HLC) because industrial and mobile sources in the inland region are the major sources of particulate emissions. These results agreed with those of previous research, indicating that PM concentration during the sea breeze periods is relatively low compared with those during the land breeze periods.Citation12 In the daytime, the highest PM10 levels (up to 90.79 ± 41.12 μg/m3) were observed at NKU, which has previously claimed to have the best air quality in the Kaohsiung metropolitan area. The averaged concentrations of PM2.5 and PM2.5–10 in the daytime were higher than those collected at night at the inland sites. During the night, the FIC site had the highest average PM2.5 con centration (42.67 ± 26.26 μg/m3), whereas the OSB site had the highest average PM2.5–10 concentration (33.68 ± 11.76 μg/m3).
Table 1. Concentrations of PM2.5, PM2.5–10, PM10, and PM2.5/PM10 at the sampling sites in the coastal region of southern Taiwan during the intensive sampling periods
The ratio of PM2.5 to PM10 (PM2.5/PM10) observed at the inland sites ranged from 0.50 to 0.63, with an average of 0.54, whereas the PM2.5/PM10 observed at the offshore sites ranged from 0.40 to 0.55, with an average of 0.47. These results indicate that the inland sites have a higher proportion of fine particles (PM2.5), whereas the offshore sites had a higher proportion of coarse particles (PM2.5–10). This result is attributed to the fact that marine aerosols are generally abundant in the coarse particles. Some previous studies concluded that the concentration of PM2.5 was approximately 60–62% of the PM10 concentration in the urban areas of Kolkata, India, and Birmingham, United Kingdom.Citation33,Citation34 In addition, a previous study found that 60–70% of urban PM10 mass in Leeds, United Kingdom, is typically in the PM2.5 (PM ≤ 2.5 μm in aerodynamic diameter) fraction, and 50% is below approximately 1.5 μm.Citation35
The particle size distribution of atmospheric aerosols was simultaneously measured with a 10-stage MOUDI (0.056–18 μm) at inland and offshore sites during the SLB periods. The variations in particle size distribution for August 16–17 and November 2–3, 2006, and May 2–4, 2007, are illustrated in . In general, a bimodal size distribution was observed for ambient aerosol particles measured at the inland and offshore sites. The particle size ranges with the highest concentration of fine and coarse particles were 0.56–1 and 3.2–5.6 μm, respectively, at the inland sites. At the offshore sites, the particle size ranges with the highest concentration of fine and coarse particles were 0.56–1 and 5.6–10 μm, respectively. The results obtained from the comparison of particle size distribution and mass concentration of PM indicate that the SLBs contributed significantly more coarse than fine particles at the offshore sites. The increase in coarse particle concentration can be used to validate the existence of SLBs because it could provide more detailed information about variation in particle size. The mass concentration of coarse particles at the offshore sites may be elevated simply because of the emission of coarse particles from oceanic sprays. During the investigation periods, the increase in fine particles at the inland sites was attributed mainly to vehicle traffic and industrial sources.
Spatial Distribution and Temporal Variation of PM
During the investigation periods, SLBs were regularly observed at offshore and inland sites in southern Taiwan. The relationship between wind speed and variations in PM10 concentration at the HLC, NKU, and FYC sites is illustrated in . The OSB site is not included in the figure because wind speed could not be measured while the boat navigated offshore. During the first intensive sampling period, PM10 levels in samples taken at the inland and offshore sites were usually lower than 70 μg/m3. Unlike the other two sites, the wind speed at the HLC site varied very little. Field measurement results showed that the highest wind speed occurred in the afternoon, when sea breezes were blowing toward the inland sites. During the second intensive sampling period, the highest PM10 concentration occurred on the first day when the wind speed was relatively low at the inland sites. At night, PM10 and its precursors over the coastal region had a tendency to flow from the land to the sea because of land breezes, resulting in relatively higher PM10 concentrations at the HLC offshore site. During the third intensive sampling period, PM10 concentration peaks up to 200 μg/m3 were frequently observed between 1:00 and 5:00 a.m. at the NKU site. illustrates the diurnal variation of inland and offshore PM10 concentrations over the south coast of Taiwan during the intensive sampling periods. At inland and offshore sites, the PM10 concentrations measured in the first intensive sampling period (August 16–18, 2006) were lower than those measured in the second and third intensive sampling periods (November 2–4, 2006 and May 2–4, 2007). This is because the atmosphere in summer is generally less stable than it is in late autumn and late spring, which causes better dispersion of air pollutants. In some cases, the offshore sites had an even higher PM10 concentration than the inland sites. A higher PM10 concentration at the offshore sites was usually observed at night. This implies that the accumulation of PM10 over the ocean because of nighttime land breezes, particularly during the first intensive period, was not uncommon over coastal Taiwan.
Figure 3. Variation in PM10 concentration with wind speed over the coastal region of southern Taiwan during the intensive sampling periods for the (a) HLC, (b) NKU, and (c) FYC sites.
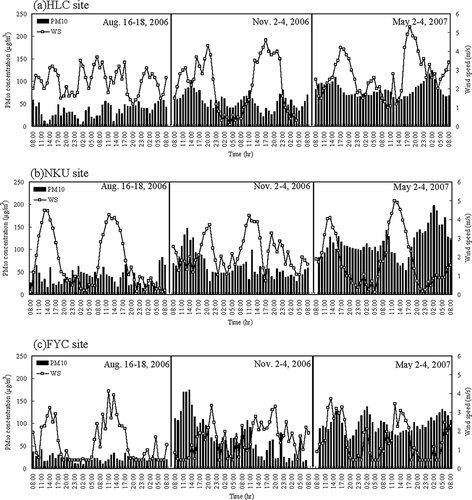
shows the effects of wind speed in diluting PM10 concentrations. PM10 concentration and wind speed at three sites had an almost negative relationship. The correlation coefficients ranged from 0.21 to -0.48, indicating a weak correlation between PM10 concentration and wind speed. Exceptions occurred at the NKU site (r = −0.48) during the third intensive sampling period and at the FYC site (r = −0.45) in the second intensive sampling period. Coarse PM (PM2.5–10) over the coastline was contributed mainly by oceanic sprays blown in from offshore.Citation36 Daytime PM concentrations were always higher than those measured at night, particularly at the offshore sites. In addition, PM10 concentrations were dominated mainly by PM2.5–10 concentrations at the offshore sites. In the study presented here, strong land breezes were regularly observed at night during the first intensive sampling period of August 16–18, 2006 (see ). High PM10 concentrations at the offshore sites were measured primarily as a result of particulate blown from the inland PM emission sources. Using wind direction measurement and wind field modeling air mass, recirculation was observed and simulated along the coastline as shown in and . The summer southwesterly flows show stronger sea breezes, with wind speeds increasing from less than 2 to 4 m/sec and wind directions confined to the sector of 200–320° from 8:00 a.m. to 8:00 p.m. Temperature contrasts between the land and sea drive sea breezes (i.e., when the land is strongly heated, such as it would be under cloudless skies with calm conditions). Strong land breezes were regularly observed at night (8:00 p.m. to 8:00 a.m.) during the first and third intensive sampling periods in August and May (see ). However, no significant SLBs appeared in the coastal region of southern Taiwan during the second intensive sampling period, from November 2–4, 2006. The wind field was dominated by the northeastern monsoon, with wind speeds of approximately 2 m/sec remaining steady throughout the afternoon and a wind direction sector of 270–300°.
Figure 4. Hourly variation of wind direction over the coastal region of southern Taiwan during the intensive sampling periods: (a) August 16–18, 2006; (b) November 2–4, 2006; and (c) May 2–4, 2007.
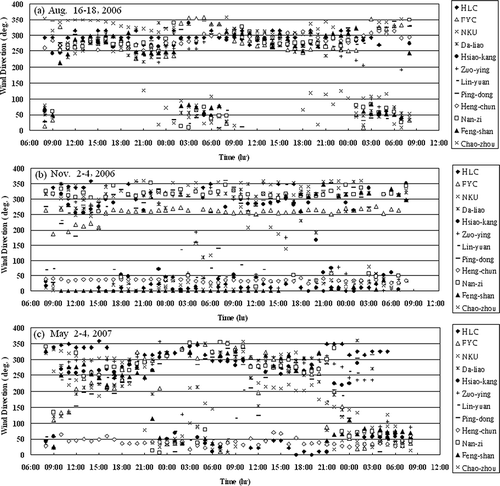
Figure 7. Variations in surface wind field simulated by MM5 over the coastal region of southern Taiwan during the intensive sampling periods: (a) August 16 at 2:00 p.m., (b) August 17 at 2:00 a.m., (c) August 17 at 2:00 p.m., (d) August 18 at 2:00 a.m. (e) November 2 at 2:00 p.m., (f) November 3 at 2:00 a.m., (g) November 3 at 2:00 p.m., (h) November 4 at 2:00 a.m. (i) May 2 at 2:00 p.m., (j) May 3 at 2:00 a.m., (k) May 3 at 2:00 p.m., and (l) May 4 at 2:00 a.m.
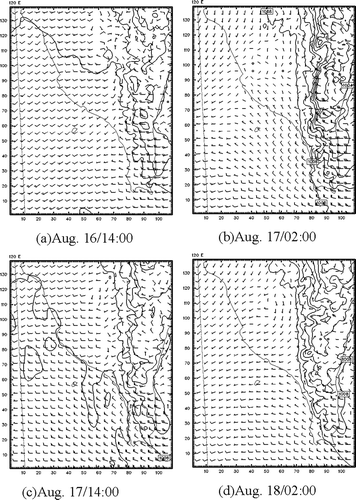
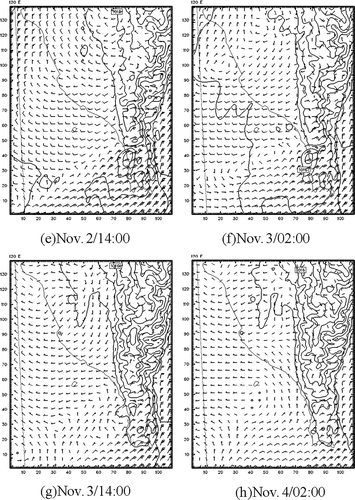
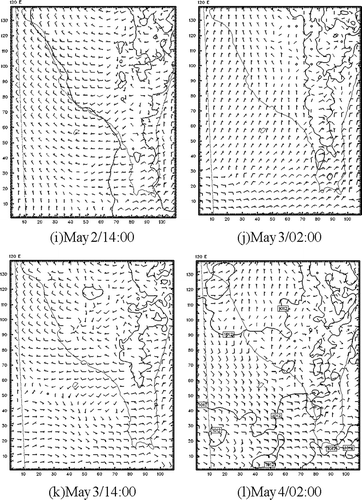
Field measurement data obtained from the inland (12 sites) and offshore (2 sites) sites were applied to plot the PM10 concentration contour with Surfer 2D plotting software. This software has previously been widely applied in studies of atmospheric heavy metal deposition and agricultural development.Citation37–39 The stations analyzed are part of the TAQMN, which was established by the TEPA in 1993. These ambient air quality monitoring stations are generally situated in populated areas and are intended to provide information pertaining to population exposure. The Kriging algorithm was used to interpolate data from 14 ambient air quality monitoring stations. The spatial distribution of the hourly PM10 concentration was further used to investigate the influences of SLBs on the transport of PM over the south coastal region of Taiwan. The results obtained from field measurement and model simulation showed that PM10 can be transported back and forth across the coastline in the particular region studied (see ).Similarly, high PM10 concentrations at the inland sites were observed in the daytime because of sea breezes, whereas high PM10 concentrations at the offshore sites were detected at night because of land breezes. In particular, PM10 was transported northeasterly to the inland region, covering a huge area of the entire inland region in autumn. Therefore, the northeastern monsoon and SLBs influenced the temporal distribution and spatial variation of PM10 over southern Taiwan's coastal region.
Figure 5. PM10 concentration contour over the coastal region of southern Taiwan during the intensive sampling periods (x- and y-axis legends are UTM [Universal Transverse Mercator] units in kilometers): (a) August 16 at 2:00 p.m., (b) August 17 at 2:00 a.m., (c) August 17 at 2:00 p.m., (d) August 18 at 2:00 a.m. (e) November 3 at 2:00 p.m., (f) November 4 at 2:00 a.m., (g) November 3 at 2:00 p.m., (h) November 4 at 2:00 a.m. (i) May 2 at 2:00 p.m., (j) May 3 at 2:00 a.m., (k) May 3 at 2:00 p.m., and (l) May 4 at 2:00 a.m.
![Figure 5. PM10 concentration contour over the coastal region of southern Taiwan during the intensive sampling periods (x- and y-axis legends are UTM [Universal Transverse Mercator] units in kilometers): (a) August 16 at 2:00 p.m., (b) August 17 at 2:00 a.m., (c) August 17 at 2:00 p.m., (d) August 18 at 2:00 a.m. (e) November 3 at 2:00 p.m., (f) November 4 at 2:00 a.m., (g) November 3 at 2:00 p.m., (h) November 4 at 2:00 a.m. (i) May 2 at 2:00 p.m., (j) May 3 at 2:00 a.m., (k) May 3 at 2:00 p.m., and (l) May 4 at 2:00 a.m.](/cms/asset/6622b09c-7eb8-4459-90a2-cbd306ac8fa1/uawm_a_10412104_o_f0005g.gif)
![Figure 5. PM10 concentration contour over the coastal region of southern Taiwan during the intensive sampling periods (x- and y-axis legends are UTM [Universal Transverse Mercator] units in kilometers): (a) August 16 at 2:00 p.m., (b) August 17 at 2:00 a.m., (c) August 17 at 2:00 p.m., (d) August 18 at 2:00 a.m. (e) November 3 at 2:00 p.m., (f) November 4 at 2:00 a.m., (g) November 3 at 2:00 p.m., (h) November 4 at 2:00 a.m. (i) May 2 at 2:00 p.m., (j) May 3 at 2:00 a.m., (k) May 3 at 2:00 p.m., and (l) May 4 at 2:00 a.m.](/cms/asset/81449323-e428-43f5-a26b-9ee3d2f1365f/uawm_a_10412104_o_f0015g.gif)
![Figure 5. PM10 concentration contour over the coastal region of southern Taiwan during the intensive sampling periods (x- and y-axis legends are UTM [Universal Transverse Mercator] units in kilometers): (a) August 16 at 2:00 p.m., (b) August 17 at 2:00 a.m., (c) August 17 at 2:00 p.m., (d) August 18 at 2:00 a.m. (e) November 3 at 2:00 p.m., (f) November 4 at 2:00 a.m., (g) November 3 at 2:00 p.m., (h) November 4 at 2:00 a.m. (i) May 2 at 2:00 p.m., (j) May 3 at 2:00 a.m., (k) May 3 at 2:00 p.m., and (l) May 4 at 2:00 a.m.](/cms/asset/87d8a12b-7745-4c41-aa65-7dcb845088cf/uawm_a_10412104_o_f0016g.gif)
As illustrated in and , once sea breezes developed, high levels of PM10 concentration in the inland region were observed (e.g., 46 μg/m3 at 2:00 p.m. on August 16, 2006) during the first intensive sampling period. At nighttime, PM10 concentrations in the southern coastal region of Kaohsiung City (∼46–52 μg/m3) were higher than those in the inland region (see and ). It is suggested that the PM10 concentrations in southern Taiwan were highly influenced by SLBs that transported PM10 back and forth across the coastline. During the second intensive sampling period, very high levels of PM10 concentration (110–175 μg/m3) were observed in the southeastern inland region in the daytime (see and ), whereas relatively high PM10 concentrations of up to 58–74 μg/m3 were monitored at the southern coastal region of Kaohsiung City. This also occurred in the offshore region at night (see and ). High concentrations of PM10 moving to the southern coastal and inland region of Kaohsiung City during daytime and nighttime hours were dominated by the northeastern monsoon. and illustrate that during the third intensive sampling period, the PM10 concentration was also affected by sea breezes, with a high PM10 concentration of 104–160 μg/m3 accumulating in the inland region of southern Taiwan in the daytime. At night, inland PM10 concentrations were at a much higher level (150–180 μg/m3) than those in the daytime (see and ), mainly because of a more stable atmosphere and lower wind speed. The paired t test was used to compare the daytime PM10 concentrations with those at night according to data collected at inland and offshore sites. The P values of NKU, FYC, OSB, and HLC are summarized in . In this work, P values less than 0.05 indicated that the daytime and nighttime PM10 concentrations were significantly influenced by SLBs. At the inland NKU and FYC sites, the daytime PM10 concentrations were significantly different from the nighttime PM10 concentrations. At the offshore OSB and HLC sites, the differences between daytime and nighttime PM10 concentrations were not as significant as those at the inland sites. During the sampling periods of this study, the sea breezes significantly influenced daytime and nighttime PM10 concentrations on the south coast of Taiwan, particularly at the inland sites.
Table 2. Results of the paired t test for comparing the daytime and nighttime PM10 concentrations at inland and offshore sites
Effects of SLBs
The backward trajectories of the air parcels transported toward the inland air quality sampling sites over the coastal region of southern Taiwan during the three intensive sampling periods are plotted in . Previous studies have applied backward trajectory models to investtigate Asian and African dust storms.Citation30,Citation40,Citation41 The results of the study presented here illustrate that during the first intensive sampling period sea breezes blown in the early morning transported the offshore PM10 back to the inland sites in the Kaohsiung metropolitan area, resulting in a higher PM10 concentration at these inland sites in the afternoon. In contrast, the high PM10 concentration observed during the second intensive sampling period was brought to the Kaohsiung metropolitan area mainly by a northerly wind that transported PM10 originating in the northern region (i.e., Tainan and Yunlin counties). The backward trajectory modeling of November 2006 suggested that the winds in this period were dominated by the northeastern monsoon and as such likely inhibited the influences of SLBs. This concurred with the wind direction measurements (see ) and the surface wind field simulated by MM5 (see ). In addition, backward trajectories indicated that the SLBs significantly influenced the transport of PM10 in late summer, whereas the northeastern monsoon blew PM10 across the boundary from the northern region and dominated the spatial distribution of PM10 in the autumn.
Figure 6. Backward trajectories of air parcels transported to the inland sampling sites over the coastal region of southern Taiwan during the intensive sampling periods (x- and y-axis legends are UTM in kilometers): (a) August 16–18, 2006; (b) November 2–4, 2006; and (c) May 2–4, 2007.
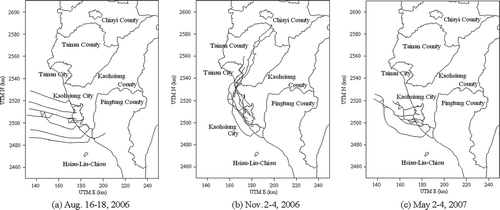
The surface wind fields simulated by the 3D meteorological model MM5 over the coastal region of southern Taiwan during all three intensive sampling periods are illustrated in . The resolution of the wind field plots was set with four layers: 27 × 27 km, 9 × 9 km, 3 × 3 km, and 1 × 1 km. MM5 was used to analyze the role of SLBs on PM in the coastal and polluted inland areas. Similar to and , shows regular SLBs over the coastal region of southern Taiwan and indicates that the complex terrain far inland to the east interferes with the wind fields. The wind fields around the coastal region of southern Taiwan were for the most part relatively uniform during all three intensive sampling periods. Local surface air mass circulation over southern Taiwan obtained from MM5 grid 4 showed the daytime onshore breezes (see and ) and nighttime offshore breezes (see and ) during the first intensive sampling period. In addition to the 2D graphics of a simulated surface wind field (see ) and hourly variations in wind direction (see ), the vertical wind field profile from the coast to inland areas 85 km from the coastline were also simulated (see ). As shown in , sea breezes blew inland from the coast on the surface and recirculated back to the coast within the top atmospheric layer in the afternoon. At night, weak land breezes blew from inland to the coast. and illustrate that air mass recirculation was frequently observed in the coastal region of southern Taiwan. The weather in November in southern Taiwan during the second intensive sampling period was occasionally influenced by the northeastern monsoon and complex terrain. illustrate typical daytime onshore and nighttime offshore cyclogenesis in southern Taiwan. During the third intensive sampling period, also show apparent sea breezes in the afternoon and land breezes at night. The results of the MM5 model demonstrate the diurnal variation of wind direction in the coastal region of southern Taiwan.
Figure 8. The vertical wind field profile in the coastal region of southern Taiwan on August 16 at (a) 2:00 p.m. and (b) 8:00 p.m.
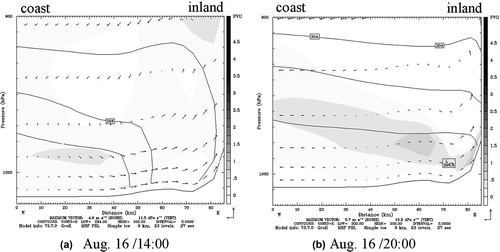
MM5 was used in conjunction with CAMx in this work to simulate PM10 dispersion for a period of 48 hr in southern Taiwan. The temporal and spatial distributions of PM10 concentrations simulated by the CAMx model are plotted in . During the first intensive sampling period, a high PM10 concentration occurred in far eastern rural regions in the afternoon because of strong sea breezes (see and ). However, the polluted air mass moved southerly to the southeastern region of Kaohsiung City (see and ), suggesting that land breezes were relatively weak. In the early morning (8:00 a.m.) of August 17, a PM10 hot spot moved southerly to the southeastern coastal region of Kaohsiung City, a highly polluted industrial region. A similar pattern of high PM10 concentration variation over the coastal region of Kaohsiung City was also observed on August 17–18, 2006. During the second intensive sampling period (see ), a strong northeastern monsoon blew PM10 from the north to the southern region in the morning. At night, land breezes blew PM10 to the coast, resulting in a high PM10 concentration over the southern coastal region of Kaohsiung City. Similarly, during the afternoon of November 3, PM10 was transported to the southeastern inland region and further covered the southern coastal region at night. Accordingly, the northeastern monsoon dominated the temporal and spatial distribution of PM10 over the southern Taiwan coast. During the third intensive sampling period, average simulated hourly concentrations of PM10 over southern Taiwan ranged from 120 to 140 μg/m3 in the afternoon (see and ). These were affected mainly by sea breezes (see ) and air mass recirculation (see ). At night, relatively higher PM10 concentrations extended westward offshore from southern Taiwan because of land breezes (see and ). The MM5 model was used in conjunction with the CAMx model in this work to simulate PM10 concentrations in southern Taiwan. The model simulation has been compared with field-measured PM10 concentrations for inland and offshore regions (see ). As shown in , the model simulation generally agreed with the field measurements with correlation coefficients (R) of 0.66–0.74 and 0.63–0.67 for inland and offshore regions, respectively (see ). Many studies indicated that the sea breezes transport pollution from coastal urban areas and spread air pollutants to an inland distance of approximately 20–60 km.Citation9–11 The implication of results of the study presented here suggest that relevant authorities should work to prevent pollution episodes that under specific weather conditions as outlined in this study lead to stagnant or recirculating air. Reducing regional PM emissions and identifying the influential region of SLBs that transport pollution over the coastal regions of southern Taiwan could achieve this.
Table 3. Correlation coefficients of the model simulation and the measurement at inland and offshore sites during the sampling periods
Figure 9. Spatial distribution of PM10 concentration simulated by CAMx over the coastal region of southern Taiwan during the intensive sampling periods (x- and y-axis legends are UTM in kilometers): (a) August 16 at 2:00 p.m., (b) August 17 at 2:00 a.m., (c) August 17 at 2:00 p.m., (d) August 18 at 2:00 a.m. (e) November 2 at 2:00 p.m., (f) November 3 at 2:00 a.m., (g) November 3 at 2:00 p.m., (h) November 4 at 2:00 a.m. (i) May 2 at 2:00 p.m., (j) May 3 at 2:00 a.m., (k) May 3 at 2:00 p.m., and (l) May 4 at 2:00 a.m.
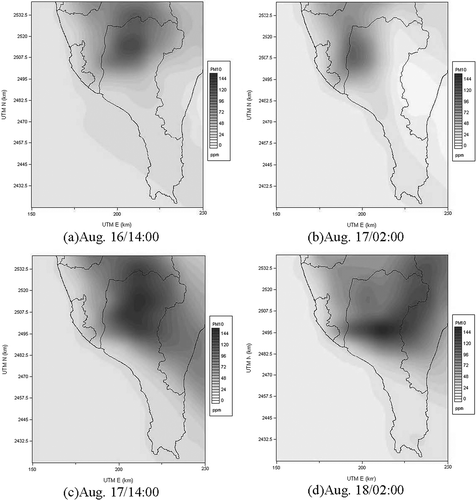
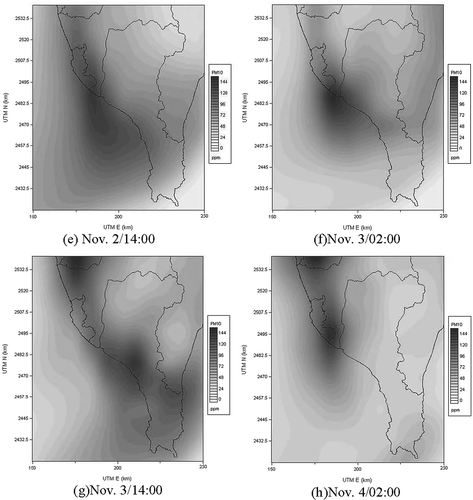
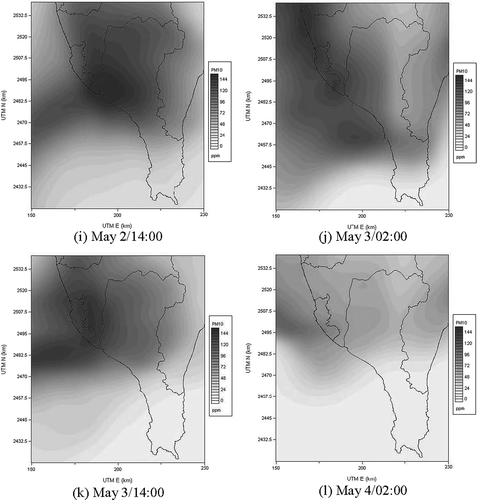
CONCLUSIONS
This study investigated the influence of SLBs on the tempospatial distribution of atmospheric aerosols over the coastal region of southern Taiwan. The results indicated that the inland sites had a higher portion of PM2.5, whereas the offshore sites had higher portion of PM2.5–10. This is because anthropogenic aerosols are comprised of mostly fine particles and marine aerosols are generally made up of coarser ones. Model simulation and field measurement results show that PM10 can be transported back and forth across the coastline of the region under investigation. High PM10 concentrations were observed at the inland sites in the daytime because of sea breezes, whereas high PM10 concentrations were detected over the ocean at night because of land breezes. Backward trajectories illustrated that SLBs significantly influenced the transport of PM10 in the autumn, whereas the northeastern monsoon blew PM10 across the boundary from the north of Kaohsiung City. This dominated the spatial distribution of PM10 in southern Taiwan. This study determined that the accumulation of PM in the near-ocean region due to SLBs has regular influence on the tempospatial distribution of PM10 in the coastal region of southern Taiwan. Moreover, SLBs in summer have a stronger influence on the tempospatial distribution of PM10 than the winds observed in other seasons.
REFERENCES
- Ding , A. , Tao , W. , Zhao , M. , Wang , T. and Li , Z. 2004 . Simulation of Sea-Land Breezes and a Discussion of Their Implications on the Transport of Air Pollution during a Multi-Day Ozone Episode in the Pearl River Delta of China . Atmos. Environ. , 38 : 6737 – 6750 .
- Oh , I.-B. , Kim , Y.-K. , Lee , H.W. and Lim , C.H. 2006 . An Observational and Numerical Study of the Effects of the Late Sea Breeze on Ozone Distributions in the Busan Metropolitan Area, Korea . Atmos. Environ. , 40 : 1284 – 1298 .
- Nester , K. 1995 . Influence of Sea Breeze Flows on Air Pollution over the Attica Peninsula . Atmos. Environ. , 29 : 3655 – 3670 .
- Venkatesan , R. , Mathiyarasu , R. and Somayaji , K.M. 2002 . A Study of Atmospheric Dispersion of Radionucleides at a Coastal Site Using a Modified Gaussian Model and a Meso-Scale Sea Breeze Model . Atmos. Environ. , 36 : 2933 – 2942 .
- Levin , Z. , Gershon , H. and Ganor , E. 2005 . Vertical Distribution of Physical and Chemical Properties of Haze Particles in the Dead Sea Valley . Atmos. Environ. , 39 : 4937 – 4945 .
- Evtyugina , M.G. , Nunes , T. , Pio , C. and Costa , C.S. 2006 . Photochemical Pollution under Sea Breeze Conditions, during Summer, at the Portuguese West Coast . Atmos. Environ. , 40 : 6277 – 6293 .
- Rodŕiguez , S. , Querol , X. , Alastuey , A. and Mantilla , E. 2002 . Origin of High Summer PM10 and TSP Concentrations at Rural Sites in Eastern Spain . Atmos. Environ. , 36 : 3101 – 3112 .
- Viana , M. , Perez , C. , Querol , X. , Alastuey , A. , Nickovic , S. and Baldasano , J.M. 2005 . Spatial and Temporal Variability of PM Levels and Composition in a Complex Summer Atmospheric Scenario in Barcelona (NE Spain) . Atmos. Environ. , 39 : 5343 – 5361 .
- Hastie , D.R. , Narayan , J. , Schiller , C , Niki , H , Shepson , P.B. , Sills , D.M.L. , Taylor , P.A. , Moroz , W.J. , Druummond , J.W. , Reid , N. , Taylor , R. , Roussel , P.B. and Melo , O.T. 1999 . Observational Evidence for the Impact of the Lake Breeze Circulation on Ozone Concentration in Southern Ontario . Atmos. Environ. , 33 : 323 – 335 .
- Kitada , T. and Kitagawa , E. 1990 . Numerical Analysis of the Role of Sea Breeze Front on Air Quality in Coastal and Inland Polluted Areas . Atmos. Environ. , 24 : 1545 – 1559 .
- Kambezidis , H.D. , Weidauer , D. , Melas , D. and Ulbricht , M. 1998 . Air Quality in the Athens Basin during Sea Breeze and Non-Sea Breeze Days Using Laser-Remote-Sensing Technique . Atmos. Environ. , 32 : 2173 – 2182 .
- Millán , M.M. , Salvador , R. , Mantilla , E. and Kallos , G. 1997 . Photo-Oxidant Dynamics in the Mediterranean Basin in Summer: Results from European Research Projects . J. Geophys. Res. , 102 : 8811 – 8823 .
- Rodŕiguez , S. , Querol , X. , Alastuey , A. , Kallos , G. and Kakaliagou , O. 2001 . Saharan Dust Contributions to PM10 and TSP Levels in Southern and Eastern Spain . Atmos. Environ. , 35 : 2433 – 2447 .
- Querol , X. , Andrés , A. , Rodriguez , S. , Plana , F. , Mantilla , E. and Ruiz , C.R. 2001 . Monitoring of PM10 and PM2.5 around Primary Particulate Anthropogenic Emission Sources . Atmos. Environ. , 35 : 845 – 858 .
- Rodríguez , S. , Cuevas , E. , González , Y. , Ramos , R. , Romero , P.M. , érez , P , Querol , N. and X.; Alastuey , A. 2008 . Influence of Sea Breeze Circulation and Road Traffic Emissions on the Relationship between Particle Number, Black Carbon, PM1, PM2.5 and PM2.5–10 Concentrations in a Coastal City . Atmos. Environ. , 42 : 6523 – 6534 .
- Chang , S.Y. , Fang , G.C. , Chou , C.C.K. and Chen , W.N. 2006 . Source Identifications of PM10 Aerosols Depending on Hourly Measurements of Soluble Components Characterization among Different Events in Taipei Basin during Spring Season of 2004 . Chemosphere , 65 : 792 – 801 .
- Tsai , H.H. , Ti , T.H. , Yuan , C.S. , Hung , C.H. and Lin , C. 2008 . Effects of Sea-Land Breezes on the Spatial and Temporal Distribution of Gaseous Air Pollutants at the Coastal Region of Southern Taiwan . J. Environ. Eng. Manage. , 18 : 387 – 396 .
- Liu , C.M. , Huang , C.Y. , Shieh , S.L. and Wu , C.C. 1994 . Important Meteorological Parameters for Ozone Episodes Experienced in the Taipei Basin . Atmos. Environ. , 28 : 159 – 173 .
- Lin , C.Y. , Wang , Z. , Chou , C.C.K. , Chang , C.C. and Liu , S.C. 2007 . A Numerical Study of an Autumn High Ozone Episode over Southwestern Taiwan . Atmos. Environ. , 41 : 3684 – 3701 .
- Cheng , W.L. 2002 . Ozone Distribution in Coastal Central Taiwan under Sea-Breeze Conditions . Atmos. Environ. , 36 : 3445 – 3459 .
- Liu , K.Y. , Wang , Z. and Hsiao , L.F. 2002 . A Modeling of the Sea Breeze and Its Impacts on Ozone Distribution in Northern Taiwan . Environ. Modell. Softw. , 17 : 21 – 27 .
- Tsai , Y.I. and Chen , C.L. 2006 . Atmospheric Aerosol Composition and Source Apportionments to Aerosols in Southern Taiwan . Atmos. Environ. , 40 : 4751 – 4763 .
- Yu , T.I. and Chang , L.F.W. 2000 . Selection of the Scenarios of Ozone Pollution at Southern Taiwan Area Utilizing Principal Component Analysis . Atmos. Environ. , 34 : 4499 – 4509 .
- Hsu , Y.C. , Lai , M.H. , Wang , W.C. , Chiang , H.L. and Shieh , Z.X. 2008 . Characteristics of Water-Soluble Ionic Species in Fine (PM2.5) and Coarse Particulate Matter (PM10–2.5) in Kaohsiung, Southern Taiwan . Journal of the Air & Waste Management Association , 58 : 1579 – 1589 . doi: 10.3155/1047-3289.58.12.1579
- Chen , K.S. , Wang , H.K. , Peng , Y.P. , Wang , W.C. , Chen , C.H. and Lai , C.H. 2008 . Effects of Open Burning of Rice Straw on Concentrations of Atmospheric Polycyclic Aromatic Hydrocarbons in Central Taiwan . Journal of the Air & Waste Management Association , 58 : 1318 – 1327 . doi: 10.3155/1047-3289.58.10.1318
- Lin , C.H. 2008 . Impact of Downward-Mixing Ozone on Surface Ozone Accumulation in Southern Taiwan . Journal of the Air & Waste Management Association , 58 : 562 – 579 . doi: 10.3155/1047-3289.58.4.562
- Liu , P.W.G. 2007 . Establishment of a Box–Jenkins Multivariate Time-Series Model to Simulate Ground-Level Peak Daily One-Hour Ozone Concentrations at Ta-Liao in Taiwan . Journal of the Air & Waste Management Association , 57 : 1078 – 1090 . doi: 10.3155/1047-3289.57.9.1078
- Lee , C.G. , Yuan , C.S. , Chang , J.C. and Yuan , C. 2005 . Effects of Aerosol Species on Atmospheric Visibility in Kaohsiung City, Taiwan . Journal of the Air & Waste Management Association , 55 : 1031 – 1041 .
- Lin , J.J. 2002 . Characterization of the Major Chemical Species in PM2.5 in the Kaohsiung City, Taiwan . Atmos. Environ , 36 : 1911 – 1920 .
- Yuan , C.S. , Lee , C.G. , Liu , S.H. , Chang , J.C. , Yuan , C. and Yang , H.Y. 2006 . Correlation of Atmospheric Visibility with Chemical Composition of Kaohsiung Aerosols . Atmos. Res. , 82 : 663 – 679 .
- Barnes , S.L. 1973 . Mesoscale Objective Map Analysis Using Weighted Time Series Observations; Technical Memo ERL NSSL-62; National Oceanic and Atmospheric Administration Washington , DC
- Chang , L.F.W. , Hwang , R.R. and Lin , S.C. 1983 . A Variation-Kinematic Model for Flow over Complex Terrain . Annual Report of Institute of Physics; Acad. Sinica, Taiwan , 13 : 89 – 102 .
- Gupta , A.K. and Nag , S. 2006 . Mukhopadhyay, U.K. Characterisation of PM10, PM2.5 and Benzene Soluble Organic Fraction of Particulate Matter in an Urban Area of Kolkata, India . Environ. Monit. Assess. , 115 : 205 – 222 .
- Harrison , R.M. , Deacon , A.R. , Jones , M.R. and Appleby , R.S. 1997 . Sources and Processes Affecting Concentrations of PM10 and PM2.5 Particulate Matter in Birmingham (UK) . Atmos. Environ. , 31 : 4103 – 4107 .
- Clarke , A.G. , Azadi-Boogar , G.A. and Andrews , G.E. 1999 . Particle Size and Chemical Composition of Urban Aerosols . Sci. Total Environ. , 235 : 15 – 24 .
- Chio , C.P. , Cheng , M. and T; Wang , C.F. 2004 . Source Apportionment to PM10 in Different Air Quality Conditions for Taichung Urban and Coastal Areas, Taiwan . Atmos. Environ. , 38 : 6893 – 6905 .
- Tsai , H.H. , Yuan , C.S. , Lin , H.Y. and Huang , M.H. 2006; Paper AB–14 . “ Physicochemical Characteristics of Suspended Particles and Hot-Spot Identification at a Highly Polluted Region ” . In Proceeding of A&WMA's 99th Annual Meeting & Exhibition , Pittsburgh , PA : New Orleans, LA, June 22–28, 2006; A&WMA .
- Ötvös , E. , Pázmándi , T. and Tuba , Z. 2003 . First National Survey of Atmospheric Heavy Metal Deposition in Hungary by the Analysis of Mosses . Sci. Total Environ. , 309 : 151 – 160 .
- Iqbaluddin , Saifuddin , Javed , A. and Moni , M. 1999 . Terrain Mapping Unit-Based Approach for Sustainable Agricultural Development in India—an Example from Plains Ecosystem at Semi-Arid Interface . J. Arid. Environ. , 42 : 319 – 330 .
- Özsoy , T. and Saydam , A.C. 2000 . Acidic and Alkaline Precipitation in the Cilician Basin, North-Eastern Mediterranean Sea . Sci. Total Environ. , 253 : 93 – 109 .
- Lee , B.K. , Lee , H.K. and Jun , N.Y. 2006 . Analysis of Regional and Temporal Characteristics of PM10 during an Asian Dust Episode in Korea . Chemosphere , 63 : 1106 – 1115 .