ABSTRACT
A major source of particle number emissions is road traffic. However, scientific knowledge concerning secondary particle formation and growth of ultrafine particles within vehicle exhaust plumes is still very limited. Volatile nanoparticle formation and subsequent growth conditions were analyzed here to gain a better understanding of “real-world” dilution conditions. Coupled computational fluid dynamics and aerosol microphysics models together with measured size distributions within the exhaust plume of a diesel car were used. The impact of soot particles on nucleation, acting as a condensational sink, and the possible role of low-volatile organic components in growth were assessed. A prescribed reduction of soot particle emissions by 2 orders of magnitude (to capture the effect of a diesel particle filter) resulted in concentrations of nucleation-mode particles within the exhaust plume that were approximately 1 order of magnitude larger. Simulations for simplified sulfuric acid-water vapor gas-oil containing nucleation-mode particles show that the largest particle growth is located in a recirculation zone in the wake of the car. Growth of particles within the vehicle exhaust plume up to detectable size depends crucially on the relationship between the mass rate of gaseous precursor emissions and rapid dilution. Chassis dynamometer measurements indicate that emissions of possible hydrocarbon precursors are significantly enhanced under high engine load conditions and high engine speed. On the basis of results obtained for a diesel passenger car, the contributions from light diesel vehicles to the observed abundance of measured nucleation-mode particles near busy roads might be attributable to the impact of two different time scales: (1) a short one within the plume, marked by sufficient precursor emissions and rapid dilution; and (2) a second and comparatively long time scale resulting from the mix of different precursor sources and the impact of atmospheric chemistry.
Volatile nucleation-mode particles still dominate curbside size distributions. In contrast to nonvolatile vehicle particle number emission factors, the formation of volatile curbside particle number concentrations depends on processes that cannot be reproduced on vehicle test benches in a reasonably economic way. Greater understanding of formation processes and subsequent growth, chemical composition, and the impact of volatile precursor mix is needed to properly evaluate health effects. An integrated approach is necessary when assessing emissions from different sources and measures.
INTRODUCTION
Two major reasons for studying ultrafine particles (UFPs: d p < 100 nm) are their impact on the earth radiation budgetCitation1,Citation2 and their impact on health.Citation3,Citation4 Health effect studies indicate that the particle number concentrations to which an individual is exposed may be more important than their mass.Citation5–7 For this reason, and the fact that the smallest particles contribute much to number and little to mass emissions, it is important to study the formation of new particles, the dilution and growth of UFPs in the exhaust plume of vehicles, and their subsequent fate.
Diesel engines in particular are a major and persistent source of exhaust particle (number) emissions. Diesel engines primarily emit aerosol precursor gases such as sulfur dioxide (SO2), sulfur trioxide (SO3), sulfuric acid (H2SO4), oxides of nitrogen (NOx), water vapor (H2O), low- and semi-volatile organic species, and soot particles. Particle size distribution measurements made on several light diesel vehicle exhausts under comparable operating conditions produced similar results, except for cases in which particle formation by nucleation occurred. Measurements revealed a consistent soot mode at a particle mobility diameter of 50–100 nm.Citation8 The European Commission has added a particle number limit to its Euro 5/6 emission standards for light vehicles. The proposed number emission limit is 5 × 10Citation11 solid particles (soot-mode particles) per kilometer driven.
Dependent on fuel composition, engine operating conditions, exhaust aftertreatment, exhaust dilution, and sampling methods, the size distribution can exhibit a second highly variable nucleation mode with diameters from 10 to 20 nm.Citation9–11 Kittelson et al.Citation12 investigated size distributions and chemical composition emitted from diesel engines in on-road and laboratory tests. Significant differences were found. On-road measurements revealed relatively high UFP concentrations and nucleation in all cases, whereas laboratory measurements showed lower UFP concentrations and often the absence of nucleation. The so-called nucleation-mode particles often dominate measured particle size distributions at curbsides (i.e., the exposure to UFP).Citation13,Citation14 Organic compounds are considered to determine the growth of nucleation-mode particles in diesel exhaust plumes.Citation12,Citation15–17 The U.S. Environmental Protection Agency has estimated that although more than 20,000 individual chemical compounds are emitted from diesel-fueled vehicles, only a few hundred have been identified so far.Citation18
Because of the high exhaust temperatures, a key factor for volatile nanoparticle formation and growth is the dilution of exhaust gases. During dilution, aerosol precursor gases cool and the saturation ratios of semi-volatile species may rapidly increase. This may lead to increased condensational growth, or where critical supersaturation ratios are reached, new particles are formed by nucleation.
The dilution conditions may be significantly different for laboratory and on-road measurements. Real-world within-exhaust plume measurements are rare and are limited to relatively few points within the plume.Citation10,Citation13 A fur ther major scientific challenge lies in improving understanding of the subsequent growth of nucleation-mode particles up to detectable sizes in particle diameter. This depends crucially on the conditions of dilution, available condensable mass, and the surface area of pre-existing particles within the exhaust plume.
The paper presented here is based on earlier work covering the measurements of number size distributions of exhaust particles and thermodynamic parameters under real traffic conditionsCitation13 and on work modeling concurrent dilution and aerosol dynamics within a diesel car exhaust under on-road conditions.Citation19 The intention here is to provide further details on volatile nanoparticle formation and subsequent growth under real-world conditions given dilution on short temporal and spatial scales. The interference (in the form of condensational sink) of soot particle emissions on nucleation is also assessed. Soot particle emissions are reduced by 2 orders of magnitude to evaluate the reduction effect of a diesel particle filter on particle formation. On the basis of simulations and additional measurements, the interaction of low-volatile emissions and flow pattern on particle growth and the associated time scales are discussed in more detail. Additional hydrocarbon (HC) measurements from a chassis dynamometer are used as an indicator for low- and semi-volatile organic precursor species in subsequent growth. A general in-plume “nucleation/growth process” concept is suggested. This is discussed with respect to volatile particle formation and growth within diesel car exhaust and allows for a summary of the main findings. Finally, the limitations encountered are discussed in the context of urban driving conditions and size distribution measurements near busy roads.
SIMULATION SETUP
FLUENT 6 (http://www.fluent.com) software, and the add-on aerosol model FPM (Fine Particle Model, http://www.aerosolmodeling.com) were used to compute three-dimensional (3D) particle concentrations. FPM and FLUENT together represent a full Eulerian solution of the particle general dynamics equation. In contrast to previous studiesCitation20–23 in which particle dynamics were only computed along selected streamlines, use of the FPM permitted a fully coupled Eulerian/Eulerian (flow/gas and particle phase) simulation of the aerosol dynamics on all grid cells. The model system is described in Uhrner et al.Citation19 and was validated by means of point measurements (temperature, relative humidity [RH], carbon dioxide [CO2], scanning mobility particle sizers [SMPS], flow velocity). Boundary conditions were chosen according to measurements.Citation13,Citation19
A 3D geometry of the test car was created and placed in a computational wind tunnel using approximately 260,000 grid cells. The grid cells range from a few millimeters in size at the tail pipe up to several centimeters in size at the inflow and outflow region. A k-ϵ turbulence model was used. A model system for the simulation of H2SO4-H2O-soot particles (core-mantle) was developed. Detailed H2SO4-H2O thermodynamics24–28 for nucleation and growth/evaporation were used. Nucleation was calculated based on Vehkamäki et al.Citation24 The H2SO4-H2O growth thermodynamics were calculated based on Zeleznik et al.Citation25 and further adapted for exhaust plume conditions in the temperature range of 300–400 K (for details, see Uhrner et al.l9). User-defined functions were coded to account for H2SO4-H2O growth, simplified organic growth, and H2SO4-H2O nucleation. Important underlying assumptions for the model are that
• | Nucleation particles can be formed by H2SO4-H2O nucleation and can further grow by condensation or coagulation. | ||||
• | To estimate the contributions of simplified organics, an additional noninteracting phase consisting of gas-oil can be added. | ||||
• | Soot particles are directly emitted from the exhaust (prescribed as a flux) and are able to grow by coagulation and condensation of H2SO4, H2O, and gas-oil assuming a core mantle spherical particle shape. Gas-oil is used as a “surrogate chemical species” to represent a low-volatile organic phase. |
Exhaust gas flow, composition, and soot-mode particle fluxes were prescribed based on vehicle operating conditions and exhaust temperature measurements in the wake of the test car. The emission factors (EFs) ofsoot-mode particles were determined by adjusting the simulated soot-mode particle flux to obtain the best fit between simulated and measured soottj;2 particle concentrations recorded at 45 and 90 cm from the exhaust pipe.Citation13,Citation19 The gaseous H2SO4exhaust flux was used as a free parameter and was related to fuel sulfur content (FSC) and the SO2-to-SO3 conversion rate. The FSC was set to 10 ppm (in mass), and the SO2-to-SO3 conversion rate was set to 1. The conversion rate setting is discussed in detail in Uhrner et al.Citation19 The nucleation rateCitation24 shows strong sensitivity with respect to temperature, RH, and H2SO4. This means that the dilution processes within the exhaust plume must be presented as accurately as possible.Citation19
MEASUREMENT SETUP
A detailed description of the measurement setup and the measurement program is given in Wehner et al.Citation13 Only a brief description is given here. Inlets and sensors were mounted on a modified bicycle rack; the measurement equipment was placed in the trunk of the test car. The horizontal distance of the sensors and inlets was adjustable in a range between 45 and 90 cm to the tailpipe. The measurement equipment consisted of one SMPS with a measurement range from 7 to 400 nm and a time resolution of 90 sec, two RH sensors, one Lyman-α hygrometer to measure the absolute humidity, three thermocouples to measure exhaust temperature and temperature at two other points within the plume, one Prandtl pitot tube to measure the flow speed within the plume, and one gas emission analyzer (NO/NOx/NO2 and CO2). In addition, an on-board diagnostic system was utilized to record car speed, engine speed, and air mass flow. The results obtained with the measurement setup were used first to validate 3D computational fluid dynamics (CFD) calculations,Citation19 thereafter to obtain boundary conditions for coupled steady-state CFD aerosol dynamics simulations, and then to derive EFs.Citation13
The test car (2l-74 KW, oxidation catalyst, Euro 3) was run with low-sulfur fuel (<10 ppm by weight), which has been regular fuel in Germany since 2004. The test car was equipped with an oxidation catalyst but not with a diesel particulate filter.
Measurements were performed on a 40-km long stretch of a new, relatively unfrequented four-lane public freeway that traversed mainly farmland, and low background concentrations could be assumed. The freeway section was driven several times in both directions, and driving conditions were kept constant during any one leg but changed from one leg to the next. The horizontal distance of the inlet system to the tailpipe (either 45 or 90 cm) was changed once during the day.
Urban measurements on public roads were also performed in the city of Leipzig (population ∼500,000 people). A circuit, approximately 4 km in length with several traffic lights, was chosen and driven several times. Because of the sampling time of the SMPS system, the focus was placed on studying average values resulting from highly variable car operation conditions.
RESULTS AND DISCUSSION
Taking previous researchCitation13,Citation19 as its starting point, the paper presented here studies and discusses further details of nucleation and particle growth using simulation and measurement results. An important factor related to the formation of new particles is the pre-existing particle surface area. Because of their larger size compared with nucleation particles, soot particles cover most of the total surface area within a diesel car exhaust plume. The preexisting particle surface area competes in the particle nucleation process for condensable vapors.Citation28,Citation29 Nowadays, according to diesel car manufacturer information, use of state-of-the-art diesel particle filters (Euro 4 and higher) reduces nonvolatile particle number emissions by up to two orders of magnitude. The numerical setup described in Uhrner et al.Citation19 for 148 km/hr and 4000 rpm was used as the control run (Ctrl) (i.e., nucleation-mode particles may be composed of H2SO4-H2O; soot-mode particles may be composed of H2SO4-H2O and soot cores). As described in Uhrner et al.,Citation19 the highest particle numbers were simulated within the exhaust pipe plane at approximately 0.5–1 m for the Ctrl; the highest nucleation rates were found in those areas where the gradients of H2SO4, temperature, and H2O were strongest and dilution gradients, as indicated by the CO2 dilution ratio, were highest. The Ctrl was based on “real-world” measurements where high nucleation particle concentrations (>1013 m−3) were recorded under high vehicle speed (∼150 km/hr) and high rpm (∼4000 rpm).Citation13,Citation19 The boundary conditions (see ) of the Ctrl were altered to assess the impact of a significantly reduced surface area on new particle formation. This meant that the simulated exhaust soot particle emission of the Ctrl was reduced by a factor of 100 (see ). The boundary conditions of the Ctrl and the numerical experiments (Exp-1 and Exp-2) are given in and . It should be noted that although an identical numerical setup is used in all three cases, two important boundary conditions are changed in Exp-1 and Exp-2. In Exp-2, an additional simplified organic phase with the chemical properties of gas-oil was utilized, whereas the soot-mode particle flux of the Ctrl was used (see ). The organic emissions were assumed to consist of 1 × 10−6 kg sec−1 condensable material (gas-oil C16H29 “surrogate”). This is assumed to correspond to 40% of the emission standard for HC Euro 3 diesel cars in Germany 2005 (i.e., 24 mg km−1 condensable HC emissions). This facilitates a simplified growth study in a rapidly diluting plume (see ).
Table 1. Boundary conditions utilized within all experiments
Table 2. Boundary conditions for the three experiments
In contrast to the H2SO4-H2O system, information on organic species, their thermodynamic properties, and interaction is very limited. Hence, no liquid-liquid interaction between the inorganic and organic phases was assumed. On the basis of an estimation of Tobias et al.,Citation16 the equilibrium vapor pressure was set to 10−10 Pa. The dilution conditions were identical for the set of simulations.
The spatial distribution of the number concentration of Exp-1 is shown in . The total number of nucleating particles in the exhaust wake of the car is given in . Compared with the Ctrl, nucleation-mode particle number concentrations are increased by approximately 1 order of magnitude because of the effect of reduced soot particles emissions. Compared with the soot-mode particle number concentrations, nucleation particles dominate within the plume (Exp-1). In this numerical experiment (Exp-1), only the effect of a significantly reduced condensational sink due to soot particles was considered. However, catalytic surfaces and particle filters can also adsorb sulfur. At high engine speed and related high exhaust temperatures and velocities, this stored sulfur may lead to a considerable increase of exhaust H2SO4.Citation26 This second effect may also have a significant influence on secondary particle formation by nucleation. However, if particles do not grow fast enough, particle concentrations may not reach the detection limit (e.g., the SMPS 7-nm detection limit used here).Citation13,Citation19 Critical factors here are the amount of available condensable species under rapid dilution conditions encountered at high vehicle speed and high exhaust gas flow. In the model runs, Ctrl and Exp-1 H2SO4-H2O growth is too slow to reach detectable size ranges. In both runs the simulated particle sizes are between 2 and 3 nm in diameter at approximately 1-m distance.
Table 3. Simulated number of nucleation particles in a box sized 2 × 2 × 10 m behind the exhaust out
Figure 1. Cross section (extent 22.5 m in length) for simulated nucleation-mode concentration (in #m−3) in the exhaust pipe plane for the simulation with the reduced particle flux (Exp-1; velocity = 148 km/hr, 4000 rpm). Different colors represent particle number concentration ranges (i.e., red colors represent higher values, and blue colors represent lower values). The length of the car is 4.28 m, the height is 1.51 m, and the extent of the represented plume is ∼15 m.
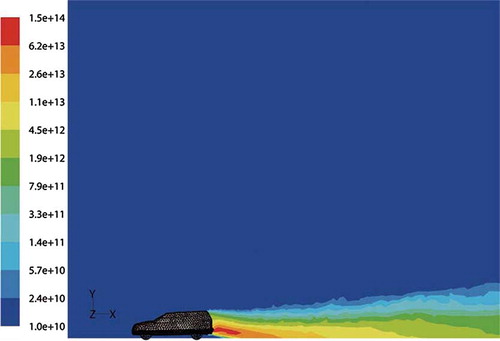
However, in Exp-2 the simulated nucleation particles attain detectable size ranges (up to 15 nm). shows the simulated geometric mean diameter for the nucleation mode within approximately 2.5 m of the exhaust plume (Exp-2). shows the flow pattern in the plume of the car for exactly the same section in the same plane as the simulated particle geometric mean diameter in . The flow vectors in the exhaust plane indicate a recirculation zone in the vehicle wake. The largest growth is located in areas with the lowest relative flow speeds, in particular within the recirculation zone ().
Figure 2. Cross section (extent 3.1 × 2.4 m) for the simulated nucleation mode d gn (in m) in the exhaust pipe plane (Exp-2; velocity = 148 km/hr, 4000 rpm). Red colors represent higher values (i.e., a d gn, of up to 15 nm, blue colors represent lower values [down to 1 nm]). The extent of the plume is ∼2.5 m identical with .
![Figure 2. Cross section (extent 3.1 × 2.4 m) for the simulated nucleation mode d gn (in m) in the exhaust pipe plane (Exp-2; velocity = 148 km/hr, 4000 rpm). Red colors represent higher values (i.e., a d gn, of up to 15 nm, blue colors represent lower values [down to 1 nm]). The extent of the plume is ∼2.5 m identical with Figure 2.](/cms/asset/a7b4405d-deb9-4c7e-983d-7fad2ec445c2/uawm_a_10412107_o_f0002g.jpg)
Figure 3. Cross section (extent 3.1 × 2.4 m) for simulated velocity vectors (in m/sec) in the exhaust pipe plane (Ctrl, Exp-1, Exp-2; 148 km/hr, 4000 rpm) relative to car speed. The length of the car is4.28 m, the height is 1.51 m, and the extent of the plume is ∼2.5 m. The different colors indicate different ranges of the velocity magnitude (in m/sec).
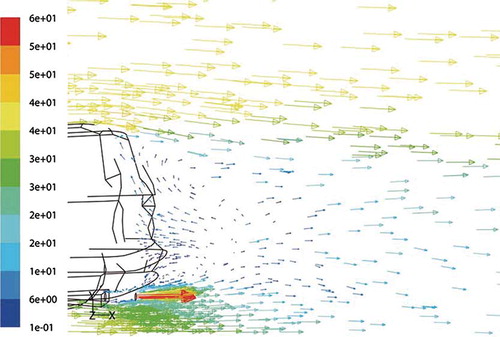
Within this set of numerical experiments, H2SO4 and H2O are the triggering species for new particle formation by nucleation. The additional condensable mass (Exp-2) in combination with decreased plume temperatureCitation19 and the reduced exhaust flow speed due to rapid dilution (see ) allow for particle growth to attain the necessary detectable range. The time scales behind the exhaust pipe, which can be inferred from the velocity vectors shown in , are within tens of microseconds within the exhaust pipe plane and below 1 sec within the recirculation zone.
Compared with the Ctrl, particle number concentrations within the exhaust wake of the car, stated in , are slightly smaller for Exp-2. The reason for this small reduction is the rapidly increased surface area of the nucleation particles consisting of H2SO4-H2O and gas-oil. The additional condensable mass also leads to increased H2SO4-H2O growth and the Kelvin effect is weakened compared with the Ctrl. As a consequence, because of lower saturation ratios the nucleation rate is weakened, yielding fewer nucleation particles. However, the effect on the actual number of nucleation-mode particles is relatively small, giving some indication that after initial formation by nucleation, particle growth is the crucial process enabling nucleation particles to reach detectable size ranges. Can engine operating conditions be identified that yield increased precursor species emissions?
Carbonaceous precursor species or HCs were not measured with the measurement setup. Thus, HC measurements from a chassis dynamometer were used as an indicator for low- and semi-volatile organic precursor species in subsequent growth. In the above cited measurements, large nucleation particle number concentrations (>1013 #m−3) were recorded only under driving conditions such as strong acceleration or high speed (>140 km/hr) and high revolutions per minute (>3800 rpm).Citation19 Under such engine operating conditions lube oil consumption is certainly increased, which could lead to higher organic and H2SO4 precursor concentrations. Chassis dynamometer measurement conditions (vehicle speed and engine speed), comparable to those used in the numerical simulations or the measurements described, were not available. shows HC emissions measured on a chassis dynamometer for four steady-state conditions for a different medium-size car equipped with a Euro 3 1.9-L diesel engine. Different engine power settings were reached by selecting different gears to obtain similar car speeds but with different engine speed. Interestingly, HC emissions are increased by up to a factor of 5.5 at higher engine rotations but for a similar car speed. Similar results were obtained for lower vehicle speeds and two other cars with different Euro 4 engine sizes (see ). This gives some indication that under high engine speed the amount of potential precursor gases is significantly increased.
Table 4. HC EFs measured on a chassis dynamometer for a Euro 3 1.9-L diesel car (different from the test car) at constant speed using different gear settings in mg km−1
Table 5. HC EFs measured on a chassis dynamometer for three different Euro 3/4 diesel engines at constant speed using different gear settings in mg km−1 and during acceleration (i.e., high engine load)
Interesting details about formation and growth can be inferred from the set of experiments presented in . shows measured size distributions at 45 and 90 cm within the exhaust plume for three different car operating conditions recorded in one experiment on the freeway. At approximately 105 km/hr and 4000 rpm () and at 150 km/hr and 3200 rpm (), nucleation particles were recorded at the 45-cm distance but significantly smaller numbers at the 90-cm distance. The number of nucleation-mode particles recorded at the 45-cm distance is approximately 105 km/hr at 4000 rpm and 150 km/hr at 3200 rpm more than 1 order of magnitude lower compared with approximately 150 km/hr at 4000 rpm. On the basis of simulation results,Citation13,Citation19 dilution leads to a decline in the number of nucleation-mode particles by a factor of 2–3 between the 45- and 90-cm sampling points. It is inferred that between 45 and 90 cm from the exhaust pipe no significant new particle formation takes place and the comparatively low number of particles (order 1012 #cm−3) recorded at 45 cm is due to dilution and to the very fast growth up to larger size ranges (15–20 nm). Hence, a distinct nucleation mode is no longer apparent in the size distribution at the second measurement point (90 cm). Consequently, the nucleation rate related to precursor flux and dilution is low. At approximately 150 km/hr and 4000 rpm (), the nucleation rate is apparently high and the exhaust precursor flux related to dilution is also sufficiently high to result in large detectable nucleation particle concentrations at both measurement points. Under these conditions, abundant nucleation-mode particles were able to grow to reach sizes of 7 nm or more. The size distribution measured at 45 cm for approximately 150 km/hr and 4000 rpm () indicates another interesting feature: The particle number increases toward the detection limit (7 nm), indicating even higher concentrations below 7 nm. In this case, the nucleation rate is comparably high in relation to the available precursors, and the time for particles to reach detectable size ranges is simply too short in the rapidly diluting plume (see ).
Figure 4. Measured size distributions at 45- and 90-cm distance behind the exhaust pipe exit for three different steady-state car operating conditionsCitation13 of (a) velocity = 105 km hr−1 and rpm = 4000 min−1, (b) velocity = 150 km hr−1 and rpm = 3200 min−1, and (c) velocity = 150 km hr−1 and rpm = 4000 min−1.
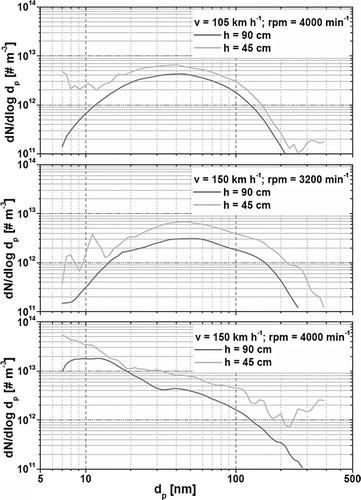
On the basis of the interpretation of those measurements and the simulation results, schematically summarizes these proposed interrelated mechanisms of nucleation and subsequent growth into detectable size ranges. The main factors related to volatile formation of new detectable particles are the interactions concerning various parameters such as the mass flux of low- and semi-volatile precursor species related to rapid dilution, the pre-existing particle surface area, and the nucleation rate itself.
Figure 5. Schematic interpretation based on measurements and results from coupled CFD-aerosol model simulations to summarize the main findings and interaction of processes for in-plume particle nucleation and growth to detectable size ranges. “Nuc rate” denotes the nucleation rate, and “prec” denotes low- and semi-volatile precursor species. The circles symbolize different effects in different “regions” dependent on the nucleation rate and the precursor flux related to dilution. In gray color, an “external” formation path outside of the plume is indicated. Precursor species originating from other sources or from the impact of atmospheric chemistry may convert these 2- to 5-nm-sized nanoparticles into size ranges that may be detectable at curbsides.
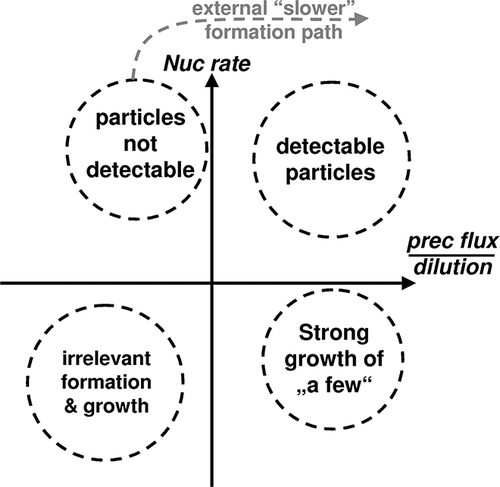
Several uncertainties and limitations encountered in the study presented here need to be addressed. Major uncertainties still remain concerning the nucleation rate and the subsequent growth to detectable limits for lowand semi-volatile precursors. There is still considerable room for improvement concerning transient simulations, time-varying boundary conditions, specification of precursor fluxes, and measurement strategy.
The concept of the nucleation rate usedCitation24 originates from atmospheric studies. Over the last few years, intense research has been undertaken to identify the precursor species involved in the initial formation process,Citation30–33 the nucleation mechanisms,Citation32,Citation34 and the impact of ambient atmospheric conditions on nucleation.Citation29,Citation30 Other researchers noted that favorable atmospheric conditions, specifically turbulent mixing processes, can enhance nucleation rates by up to several orders of magnitude.Citation35–38 Hellmuth and HelmertCitation38 pointed out that second-order turbulence statistics should be used to represent spatio-temporal variations acting upon the nucleation rate and that there is a strong effect increasing the H2SO4-H2O nucleation rate. On the basis of these findings, a second-order turbulence closure approach was utilized in the study presented here, and the mesh was chosen to be smallest near the region where the strongest dilution takes place. Remaining uncertainties relate to the interaction of H2SO4-H2O with other species and its impact on nucleation rates. Mathis et al.Citation39 investigated the role of organic vapors in the dilution air of diesel exhaust. Organic compounds such as alcohols, and to a lesser extent toluene, increased the number of nucleation particles. The exhaust H2SO4 concentration is, in addition to humidity and temperature, one of the most sensitive parameters in the study presented here. Several studies indicated that exhaust secondary particle formation is related to high FSC and exhaust aftertreatment such as the use of oxidation catalysts.Citation9,Citation10,Citation40 Measurements of gaseous H2SO4 are extremely difficult to perform.Citation41 So far, exhaust gaseous H2SO4 measurements have been carried out only by Arnold et al.,Citation42 indicating maximum SO2-to-SO3 conversion of up to 90%. In conclusion, in any assessment of the nucleation rate, there is plenty of scope for uncertainty. In the simulations described here, 10-parts per million (ppm) FSC and a conversion rate of 100% were used; hence, the simulated sulfate is actually overestimated (results in higher nucleation rate). However, on the basis of the possibility of additional sulfur contributions under high load conditions as discussed in Uhrner et al.Citation19 or Du and Yu,Citation23 it is assumed that the modeled nucleation rate still lies within in a reasonable range, given the uncertainties concerning nucleation rate parameterization.
In contrast, the subsequent growth after the initial particle formation process is probably the most crucial and challenging part in studying volatile nanoparticle formation. Knowledge about nucleation particle composition, their gaseous-precursor-related functional groups, and thermodynamic properties (e.g., activities in mixtures in wide temperature ranges) is very limited.Citation16–18,Citation39 Sakurai et al.Citation17 analyzed nucleation-mode particles and concluded that they are related to lube oil. Kittelson et al.Citation12 found most of the mass of particles in the 12- to 30-nm range to be volatile, with properties indicating the C24–C32 n-alkane compounds typical of lubricating oil. Moreover, species composition may even depend on the impact of vehicle operation conditions (e.g., load, creeping, idling, or cold start) on precursors within the vehicle exhaust plume over short time periods. The HC measurements presented here do in fact give some indication that at high engine speed the amount of precursor species may be increased. On the basis of the low-sulfur fuel used in Uhrner et al.,Citation19 the model simulations indicate that even with the highest SO2-to-SO3 conversion, the recorded nucleation particles must consist mainly of other volatile and semi-volatile matter. In contrast to simulations, nucleation-mode particle (size distribution) measurements generally represent the product of nucleation and subsequent growth up to detectable size ranges (depending on the measurement equipment used). Thus, resultsCitation9–11,Citation39 attributed to particle formation and related to high FSC (>300 ppm) fulfilled both conditions indicated in (i.e., a sufficiently high nucleation rate and enough lowand semi-volatile precursor species, with respect to dilution, to grow particles into detectable size ranges). Du and YuCitation23 concluded, based on a detailed modeling study representing heavy-duty vehicle conditions, that vehicles equipped with continuously regenerating diesel particle filters (CRDPFs) using low-sulfur fuel generate nucleation particles comparable in number to those without CRDPFs using high-sulfur fuel (>300 ppm). However, the simulated nanoparticles under CRDPF/low-sulfur fuel conditions exhibited a mean size of approximately 3 nm within 45 sec of plume age, whereas under high-sulfur fuel simulations an additional “detectable” mode (mean size 10 nm) was reproduced. Therefore, high nucleation rates and insufficient or slow growth (see ) within vehicle exhaust plumes might be a relevant mechanism resulting in stable particles growing during a second and comparatively long time scale into detectable particles. Recent smog chamber experiments with diesel exhaustCitation43,Citation44 indicate the significant growth potential on time scales from tens of minutes to a few hours. Du and YuCitation23 estimated the lifetime of 2- to 3-nm mean-sized nanoparticles, related to vehicles equipped with CRDPFs using low-sulfur fuel (<15 ppm), to approximately 300 sec. As a result of the relatively short periods involved in nucleation particle growth, instruments used in chasing experiments, or even “in-plume” measurements, need to feature a low detection limit coupled with high time resolution. The assumed spherical particle shape in the modeling work is a further constraint. For example, the fractal shape of soot particles affects condensation via surface area and coagulation. This and other such effects need to be considered in the modeling process.
The primary objective of the research work presented here was to study new particle formation in a rapidly diluting plume with particular focus on diesel cars. The most meaningful results about nucleation particles were recorded under high vehicle speed and engine speed conditions. On the basis of these measurements, simulations for constant speed conditions were performed to study the interaction of various processes under rapid dilution. Initially, one major motivation of this project was to explain the nucleation mode typically monitored at roadsides. An example is given in . This represents an urban street canyon site (Eisenbahnstrabe measurement siteCitation45) passed by the test car during urban measurements.Citation13 The street canyon size distribution shows a dominant nucleation mode (maximum particle number concentrations ∼2 × 1010 #m−3 at ∼15 nm) and a soot mode (maximum particle number concentrations ∼2 × 1010 #m−3 at 50–60 nm).
Figure 6. Median number size distributions from urban traffic measurements within the plume of the diesel car and at the Eisenbahnstrabe measurement siteCitation45 in Leipzig on March 31, 2006.
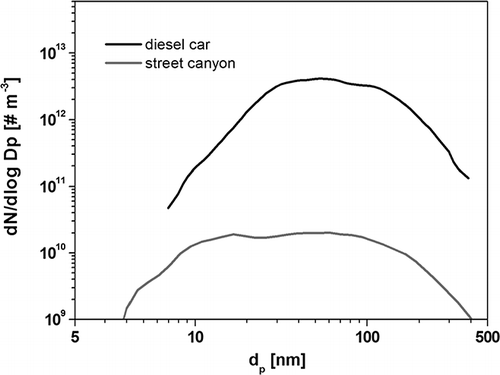
Nucleation particle number concentrations (order 1011 #m−3 at ∼15 nm), approximately 1 order of magnitude lower than soot-mode particle number concentrations (∼4 × 1012 #m−3 at ∼50 nm), were recorded with the measurement setup under urban conditions.Citation13 The most probable reasons for the lack of clarity concerning results obtained for nucleation-mode particles under urban driving conditions relate to the SMPS scanning time, the use of low-sulfur fuel, and the relatively high background particle number concentrations.Citation13
Urban traffic with frequent acceleration and deceleration cycles may result in short periods of high engine load and engine speed operating conditions favorable for the generation of nucleation particles and strong growth at very short time scales within the vehicle exhaust plume. Thus, results found under steady-state conditions for higher vehicle speeds might be transferable to some extent to urban driving conditions. It can be seen in that HC emissions measured on a chassis dynamometer are increased by up to a factor of 3.2 for higher engine rotational speed conditions at a given car speed. HC emissions measured under acceleration situations at approximately 35–50 km/hr (see , last row) also increased. On the basis of the simulations presented, the HC emissions given in must be viewed as being comparatively small (given the fact that not all of the mass is lowor semi-volatile). Because of limitations concerning the numerical approach and the missing information on time-varying boundary conditions, it was impossible to simulate the time-dependent interaction of dilution, nucleation, and growth. Most likely, the conditions for in-plume particle formation and growth to detectable size ranges are suitable for short-term periods (a few seconds). Eventually particles form but do not grow to reach detectable size ranges (see ). In urban street canyons in particular, additional contributions of organic and inorganic precursor gases (e.g., from other vehicles) are likely to lead to increased condensable gaseous precursor concentrations. Compared with light diesel vehicles, light gasoline vehicle HC emissions are usually significantly higher for the same vehicle type for a given emission standard (i.e., for the same Euro class).Citation13 Moreover, the influence of radiation may further generate low vapor pressure products leading to further growth.Citation43 For these reasons, in an external formation path based on a comparatively long time scale is also indicated. This is likely to be important for urban conditions. When analyzed in combination, the processes may explain the abundance of nucleation particles still recorded near busy urban roads.
SUMMARY
The paper presented here analyzes initial UFP formation and subsequent particle growth in the exhaust plume of a passenger car under typical on-road dilution conditions. CFD software and an aerosol model were used to compute 3D particle concentrations to describe the interaction of flow, dilution of precursor species, nucleation-mode particles, and soot-mode particles as well as their aerosol dynamics. In this connection, the interpretation of detailed measurements was also undertaken.
The synopsis of measurements and simulations indicates the complexity of nucleation and subsequent particle growth related to the dilution pattern, the available condensable mass, and soot-mode particles acting as a condensational sink. A prescribed reduction of soot particle emissions by 2 orders of magnitude resulted in approximately 1 order of magnitude larger nucleation particle concentrations within the exhaust plume. The simulations show that the growth of nucleation particles up to approximately 10 nm in diameter has two important prerequisites:
1. | Favorable conditions to trigger new particle formation by nucleation. | ||||
2. | Enough rapidly condensing, most likely organic, low- and semi-volatile species. |
The spatial distribution in nucleation particle size and the flow pattern show the interaction of flow and dilution acting upon the condensation time scale. The largest particles were simulated within the recirculation zone in the wake of the test car. Additional chassis dynamometer HC emission measurements indicate that low- and semi-volatile organic precursor species are increased at high engine speeds. Urban driving conditions may provide temporarily suitable conditions for nucleation and particle growth within the exhaust plume of light diesel vehicles using low-sulfur fuel (<10 ppm). On the basis of these results, the contributions from light diesel vehicle exhaust to the observed abundance of nucleation particles close to busy urban roads might be attributable to the effect of two different time scales:
1. | A short one with very fast-growing particles reaching detectable size within the plume. Growth here is due to the interaction between sufficient emissions of precursor species and rapid dilution. | ||||
2. | A comparatively long time scale resulting from nanoparticle formation (2–5 nm in size) and slower subsequent growth, determined by the availability of precursors from other vehicles and the effect of atmospheric chemistry. |
ACKNOWLEDGMENTS
The German Research Council (DFG WI 1449/8-1-2) funded this project.
REFERENCES
- Jacobson , M.Z. 2002 . Control of Fossil-Fuel Particulate Black Carbon and Organic Matter, Possibly the Most Effective Method of Slowing Global Warming . J. Geophys. Res , 107 : 4410
- Penner , J.E. , Zhang , S.Y. and Chuang , C.C. 2003 . Soot and Smoke Aerosol May Not Warm Climate . J. Geophys. Res. , 108 : 4657
- Pope , C.A. 2000 . What do Epidemiologic Findings Tell Us about Heath Effects of Environmental Aerosols? . J. Aerosol Med , 1314 : 335 – 354 .
- Somers , C.M. , McCarry , B.E. , Malek , F. and Quinn , J.S. 2004 . Reduction of Particulate Air Pollution Lowers the Risk of Heritable Mutations in Mice . Science , 304 : 1008 – 1010 .
- Peters , A. , Wichmann , H.E. , Tuch , T. , Heinrich , J. and Heyder , J. 1997 . Respiratory Effects are Associated with the Number of Ultrafine Particles . Am. J. Respir. Crit. Care Med , 155 : 1376 – 1383 .
- Donaldson , K. , Li , X.Y. and MacNee , W. 1998 . Ultrafine (Nanometre) Particle Mediated Lung Injury . J. Aerosol Sci , 29 : 553 – 560 .
- Sydbom , A. , Blomberg , A. , Parnia , S. , Stenfors , N. , Sandstrom , T. and Dahlen , S.E. 2001 . Health Effects of Diesel Exhaust Emissions . Eur. Resp. J , 17 : 733 – 746 .
- Harris , S.J. and Maricq , M.M. 2001 . Signature Size Distributions for Diesel and Gasoline Engine Exhaust Particulate Matter . J. Aerosol Sci , 32 : 749 – 764 .
- Maricq , M.M. , Chase , R.E. , Xu , N. and Laing , P. 2002 . The Effects of the Catalytic Converter and Fuel Sulfur Level on Motor Vehicle Particulate Matter Emissions: Light Duty Diesel Vehicles . Environ. Sci. Technol , 36 : 283 – 289 .
- Vogt , R. , Scheer , V. , Casati , R. and Benter , T.H. 2003 . On-Road Measurement of Particle Emission in the Exhaust Plume of a Diesel Passenger Car . Environ. Sci. Technol , 37 : 4070 – 4076 .
- Giechaskiel , B. , Nziachristos , L. , Samaras , Z. , Scheer , V. , Casati , R. and Vogt , R. 2005 . Formation Potential of Vehicle Exhaust Nucleation Mode Particles On-Road and in the Laboratory . Atmos. Environ , 39 : 3191 – 3198 .
- Kittelson , D.B. , Watts , W.F. and Johnson , J.P. 2006 . On-Road and Laboratory Evaluation of Combustion Aerosols. Part 1: Summary of Diesel Engine Results . J. Aerosol Sci , 37 : 913 – 930 .
- Wehner , B. , Uhrner , U. , von Löwis , S. , Zallinger , M. and Wiedensohler , A. 2009 . Aerosol Number Size Distributions within the Exhaust Plume of a Diesel and a Gasoline Passenger Car under On-Road Conditions and Determination of Emission Factors . Atmos. Environ , 43 : 1235 – 1245 .
- Wahlin , P. 2009 . Measured Reduction of Kerbside Ultrafine Particle Number Concentrations in Copenhagen . Atmos. Environ , 43 : 3645 – 3647 .
- Khalek , I.A. , Kittelson , D.B. and Brear , F. 2000 . Nanoparticle Growth during Dilution and Cooling of Diesel Exhaust: Experimental Investigation and Theoretical Assessment , Warrendale , PA : Society of Automotive Engineers (SAE) Paper 2000–01–0515; SAE .
- Tobias , H.J. , Beving , D.E. , Ziemann , P.J. , Sakurai , H. , Zuk , M. , Mc-Murry , P.H. , Zarling , D. , Waytulonis , R. and Kittelson , D.B. 2001 . Chemical Analysis of Diesel Engine Nanoparticles Using a Nano-DMA/Thermal Desorption Particle Beam Mass Spectrometer . Environ. Sci. Technol , 35 : 2233 – 2243 .
- Sakurai , H. , Tobias , H.J. , Park , K. , Zarling , D. , Docherty , S. , Kittelson , D.B. , McMurry , P.H. and Ziemann , P.J. 2003 . On-Line Measurements of Diesel Nanoparticles Composition and Volatility . Atmos. Environ , 37 : 1199 – 1210 .
- Sehlstedt , M. , Forsberg , B. , Westerholm , R. , Boman , C. and Sandström , T. 2007 . The Role of Particle Size and Chemical Composition for Health Risks of Exposure to Traffic-Related Aerosols—A Review of the Current Literature , Stockholm , , Sweden : Swedish Road Administration .
- Uhrner , U. , von Löwis , S. , Vehkamäki , H. , Wehner , B. , Bräsel , S. , Hermann , M. , Stratmann , F. , Kulmala , M. and Wiedensohler , A. 2007 . Dilution and Aerosol Dynamics within a Diesel Car Exhaust Plume-CFD Simulations of On-Road Conditions . Atmos. Environ , 41 : 7440 – 7461 .
- Kim , D.-H. , Gautam , M. and Gera , M. 2001 . On the Prediction of Concentration Variations in a Dispersing Heavy-Duty Truck Exhaust Plume Using k-ϵ Turbulent Closure . Atmos. Environ , 25 : 5267 – 5275 .
- Kim , D.-H. , Gautam , M. and Gera , M. 2002 . Parametric Studies on the Formation of Diesel Particulate Matter via Nucleation and Coagulation Modes . J. Aerosol Sci , 33 : 1609 – 1621 .
- Jiang , P. , Lignell , D.O. , Kelly , K. , Lighty , J.S. , Sarofim , A.F. and Montgomery , C.J. 2005 . Simulation of the Evolution of Particle Size Distributions in a Vehicle Exhaust Plume with Unconfined Dilution by Ambient Air . J. Air & Waste Manage. Assoc , 55 : 437 – 445 .
- Du , H. and Yu , F. 2008 . Nanoparticles Formation in the Exhaust of Vehicles Running on Ultra-Low Sulphur Fuel . Atmos. Chem. Phys , 8 : 4729 – 4739 .
- Vehkamäki , H. , Kulmala , M. , Lehtinen , K.E.J. and Noppel , M. 2003 . Modelling Binary Homogeneous Nucleation of Water-Sulfuric Acid Vapours: Parameterisation for High Temperature Emissions . Environ. Sci. Technol , 37 : 3392 – 3398 .
- Zeleznik , F.J. 1991 . Thermodynamic Properties of the Aqueous Sulphuric System to 350 K . J. Phys. Chem. Ref. Data , 20 : 1157 – 1200 .
- Wilck , M. and Stratmann , F. 1997 . A 2-D Multicomponent Modal Aerosol Model and its Application to Laminar Flow Reactors . J. Aerosol Sci , 28 : 959 – 972 .
- Wilck , M. 1999 . Modal Modelling of Multicomponent Aerosols , Berlin , , Germany : Verlag für Wissenschaft und Forschung .
- Pirjola , L. , Kulmala , M. , Wilck , M. , Bischoff , A. , Stratmann , F. and Otto , E. 1999 . Formation of Sulphuric Acid Aerosols and Cloud Condensation Nuclei: An Expression for Significant Nucleation and Model Comparison . J. Aerosol Sci , 30 : 1079 – 1094 .
- Birmili , W. , Berresheim , H. , Plass-Dülmer , C. , Elste , T. , Gilge , S. , Wiedensohler , A. and Uhrner , U. 2003 . The Hohenpeissenberg Aerosol Formation Experiment (HAFEX): A Long-Term Study Including Size-Resolved Aerosol, H2SO4, OH, and Monoterpenes Measurements . Atmos. Chem. Phys , 3 : 361 – 376 .
- Weber , R.J. , McMurry , P.H. , Mauldin , L. , Tanner , D.J. , Eisele , F.L. , Brechtel , F.J. , Kreidenweis , S.M. , Kok , G.L. , Schillawski , R.D. and Baum-gardner , D. 1998 . A Study of New Particle Formation and Growth Involving Biogenic Gas Species Measured during ACE 1 . J. Geophys. Res , 103 : 16385 – 16396 .
- Coffmann , D.J. and Hegg , D.A. 1995 . A Preliminary Study of the Effect of Ammonia on Particle Nucleation in the Marine Boundary Layer . J. Geophys. Res , 100 : 7147 – 7160 .
- Kulmala , M. , Pirjola , L. and Mäkelä , J.M. 2000 . Stable Sulphate Clusters as a Source of New Atmospheric Particles . Nature , 404 : 66 – 69 .
- Berndt , T. , Böge , O. , Stratmann , F. , Heintzenberg , J. and Kulmala , M. 2005 . Rapid Formation of Sulfuric Acid Particles at Near-Atmospheric Conditions . Science , 307 : 698 – 700 .
- Yu , F. and Turco , R.P. 2000 . Ultrafine Aerosol Formation via Ion-Mediated Nucleation . J. Geophys. Res , 27 : 883 – 886 .
- Easter , R.C. and Peters , L.K. 1994 . Binary Homogeneous Nucleation: Temperature and Relative Humidity Fluctuations, Nonlinearity, and Aspects of New Particle Formation in the Atmosphere . J. Appl. Met , 33 : 775 – 784 .
- Nilsson , E.D. and Kulmala , M. 1998 . The Potential for Atmospheric Mixing Processes to Enhance the Binary Nucleation Rate . J. Geophys. Res , 103 : 1381 – 1389 .
- Uhrner , U. , Birmili , W. , Stratmann , F. , Wilck , M. , Ackermann , I.J. and Berresheim , H. 2003 . Particle Formation at a Continental Background Site: Comparison of Model Results with Observations . Atmos. Chem. Phys , 3 : 347 – 359 .
- Hellmuth , O. and Helmert , J. 2002 . In Air Pollution Modeling and Its Application XV , Edited by: Borrego , C. and Schayes , G. 295 – 304 . New York : Kluwer Academic/ Plenum .
- Mathis , U. , Mohr , M. and Zenobi , R. 2004 . Effect of Organic Compounds on Nanoparticles Formation in Diluted Diesel Exhaust . Atmos. Chem. Phys , 4 : 609 – 620 .
- Baumgard , K.J. and Johnson , J.H. 1996 . The Effect of Fuel and Engine Design on Diesel Exhaust Particle Size Distributions; Society of Automotive Engineers (SAE) 960131 , 37 – 50 . Warrendale , PA : SAE .
- Berresheim , H. , Elste , T. , Plass-Dülmer , C. , Eisele , F.L. and Tanner , D.J. 2000 . Chemical Ionisation Mass Spectrometer Long-Term Measurements of Atmospheric OH and H2SO4 . Int. J. Mass Spectrom , 202 : 91 – 109 .
- Arnold , F. , Pirjola , L. , Aufmhoff , H. , Schuck , T. , Lahde , T. and Hameri , K. 2007 . First Gaseous Sulphuric Acid Measurements in Automobile Exhaust: Implications for Volatile Nanoparticle Formation . Atmos. Environ , 40 : 7097 – 7105 .
- Robinson , A.L. , Donahue , N.M. and Pandis , S.N. 2007 . Rethinking Organic Aerosols: Semivolatile Emissions and Photochemical Aging . Science , 315 : 1259 – 1262 .
- Chirico , R. , DeCarlo , P.F. , Heringa , M.F. , Tritscher , T. , Richter , R. , Prévôt , A.S.H. , Dommen , J. , Weingartner , E. , Wehrle , G. , Gysel , M. , Laborde , M. and Baltensperger , U. 2010 . Impact of Aftertreatment Devices on Primary Emissions and Secondary Organic Aerosol Formation Potential from In-Use Diesel Vehicles: Results from Smog Chamber Experiments . Atmos. Chem. Phys. Discuss , 10 : 16055 – 16109 .
- Tuch , T. , Herbarth , O. , Franck , U. , Peters , A. , Wehner , B. , Wiedensohler , A. and Heintzenberg , J. 2006 . Weak Correlation of Ultrafine Aerosol Particle Concentrations < 800 nm between Two Sites within One City . J. Expo. Sci. Environ. Epidemiol , 16 : 486 – 490 .