ABSTRACT
Bioaerosols generated during livestock and poultry production are significant occupational hazards. This study investigates the characteristics of bioaerosols released from animal feces. Fresh feces from pigs and chickens were obtained and tested in a controlled-environment facility. Airborne viable (culturable) bacteria and fungi were sampled hourly for 48 hr. The predominant species were identified via polymerase chain reaction analysis. The number of bacterial colonies released from chicken feces increased gradually, peaked at approximately 20 hr, and remained relatively constant to test end; however, the bacterial colonies released from swine feces did not increase significantly. The chicken feces released significantly (P < 0.05) more bacterial aerosols than swine feces over 40 hr, by approximately 1 order of magnitude. However, the difference in total fungal aerosols released from the two feces types was relatively small (30–40%) and insignificant (P > 0.05). Aerosols sized between approximately 0.65 and 1.1 μm were predominant for bacteria, whereas aerosols sized between approximately 2.1 and 3.3 μm prevailed for fungi. Genera Stenotrophomonas were the predominant bacterial aerosols, whereas Cladosporium and Acremonium accounted for the greatest amounts of fungi from chicken and swine feces, respectively. More than 1000 culturable bacterial colonies can be released from 1 g of chicken feces per hour, and approximately 80% of these bioaerosols are respirable. Most bacterial aerosols released from swine and chicken feces were opportunistic human pathogens; thus, the significance of their presence warrants further investigations.
Bioaerosols existing in livestock and poultry farms have been identified as significant occupational health hazards; however, their sources are hardly assessed. This study explored the characteristics of viable microbial aerosols released from chicken and swine feces and found that chicken feces released significantly more bacterial aerosols (>1000 CFU/g/hr) than those from swine, and approximately 80% of these particles are classified respirable. Nonetheless, differences in the total number of fungal aerosols released from swine and chicken feces were small. Most bacterial aerosols released from swine and chicken feces were Gram-negative opportunistic human pathogens.
INTRODUCTION
Airborne contaminants that are generated in the raising of livestock and poultry detrimentally affect the health of animals and air quality in nearby areas and pose occupational health hazards to workers.Citation1–4 These contaminants include toxic gases (such as ammonia [NH3] and hydrogen sulfide [H2S]), inorganic particulates (such as soil dusts), nonviable organic particles (such as feed/feces droplets and feathers/dandruff), and viable particulates (such as bacteria and fungi) and their byproducts (such as fragments and endotoxins), which are sometimes referred to as “bioaerosols.”Citation4–9
High animal densities are typical of modern large-scale poultry and swine production, and they increase the load of airborne contaminants. The concentration and characteristics of a contaminant depend on the production process. For example, open-air swine houses generally have lower levels of airborne dust, endotoxins, NH3, carbon dioxide (CO2), H2S, and microorganisms than do enclosed buildings, although the benefits of open-air houses may be compromised by irregular cleaning, the accumulation of water, and poor waste separation.Citation4,Citation10 Swine confinement buildings that are equipped with systems that separate solid and liquid manure have better air quality than conventional systems without such separation.Citation9 Workers who manually feed nursery pigs suffer from high exposure to airborne bacteria, odors, and H2S.Citation3,Citation11–13 Similarly, the size distribution and concentrations of particulates in swine buildings are affected by the degree of animal activity, ventilation, bedding materials, and floor type.Citation12,Citation13 Oppliger and co-workersCitation2 demonstrated that occupational exposure to inhalable dusts, endotoxins, and bacteria is related to the growth stage of the animals because the biomass of feces and feather dandruff increase drastically during fattening periods. However, contamination sources have seldom been identified.
Microbial aerosols in swine buildings are composed primarily Gram-positive bacteria, actinomycetes, molds, and viruses.Citation14–16 Nehme and co-workersCitation17 demonstrated that swine manure is a principal source of microbial contamination in confined pig houses, and workers in the swine waste recycling industry are exposed to very high levels of microorganisms.Citation18,Citation19 Similarly, fecal matter disposal systems affect the composition of bioaerosol in chicken egg production farms.Citation20 Accordingly, an investigation of the characteristics of bioaerosols that are released from animal feces is important to identifying the impact of contaminants in relevant areas and in determining their potential as biohazards in support of the development of appropriate control measures.
MATERIALS AND METHODS
Study Design
This investigation examines the characteristics of airborne viable (culturable) bacteria and fungi that are released from animal feces in a controlled-environment facility (CEF). Fresh feces from swine and chickens were obtained and tested in the CEF. Microbial aerosols were sampled hourly for 48 hr. The kinetics of the total colonies and the size distribution of these microorganisms were determined. Predominant colonies were purified and identified by polymerase chain reaction (PCR) analysis.
Controlled Facility
The CEF herein was a double-layer stainless-steel test chamber with dimensions 83 cm (width) × 80 cm (depth) × 100 cm (height) (internal dimensions). The dilution air was supplied via an oil-free pump, and a high-efficiency particulate air (HEPA) filter removed background particles. The moisture in the air was removed by copper sulfate (CuSO4) and then enriched using an ultrasonic water bath to the desired humidity. The water bath was kept dry when not in use, and the water (deionized) was sterilized before use. The temperature and relative humidity (RH) of the system were controlled electronically and the air-exchange rate was determined from the outlet flow rate. Diffusion discs were placed at the inlet and outlet of the CEF to ensure that air mixed in the system. On the basis of the CO2 decay protocol, an ASTM5116-97 protocol, the average mixing level of air in the CEF under ambient conditions was 84%. Air samples were obtained at the system outlet. The experimental temperature and RH were set to 30 °C (± 5%) and 65% (±5%), respectively. These values were adopted from a study that was previously carried out in Taiwan.Citation6 The air-exchange rate (ACH) was 1 hr−1 (± 3%), which is typical of buildings with mild ventilation. Such setup yielded an airflow of 0.01 m/sec above the sample. The outlet gas was directed to a chemical hood and exhausted.
The CEF was cleaned with 75% ethanol and then purged with pure nitrogen gas before each use. Experimental conditions were equilibrated for 2–3 hr before the test, and a background sample was obtained before the experiment began to correct the data.
Test Protocol
Samples of feces from chicken and swine were collected from local farms in central Taiwan. The chickens were being raised in an enclosed farm ∼4.5 kg per animal; density of 233.9 kg/m2) and fed on forage (a mixture of soybean, corn, fish powder, and cattle fat). The pigs were being raised in an enclosed house (∼75 kg per animal; density of 157.2 kg/m2) and fed domestic food waste, bran, and locally harvested plants. A clean sheet of cardboard was placed on the floor to collect chicken feces. The swine samples were collected from a washed floor. After a fresh sample was collected, it was placed in a sterilized Petri dish, sealed with Parafilm, stored in a cooler at approximately 5 °C, and shipped to the laboratory within 30 min. The mean (± standard deviation [SD]) moisture levels of similar chicken and swine fecal samples (n = 3 for each), determined based on the weight difference before and after heating at 105 °C for 2 hr, were 77.5 ± 3.1% and 66.9 ± 3.7%, respectively.
After the CEF had stabilized and a background sample was obtained, approximately 14.5 g of the samples was transferred to another Petri dish and loaded into the CEF for tests. Air samples were then taken hourly for 48 hr. Bacteria were sampled first, and then fungi were sampled. Airborne viable bacteria and fungi were collected using a size-selective six-stage Andersen cascade impactor (Thermo Fisher Scientific, Inc.) that was operated at 28.3 L/min. The aerodynamic diameters (μm) were 7 or greater, between 4.7 and 7, between 3.3 and 4.7, between 2.1 and 3.3, between 1.1 and 2.1, and between 0.65 and 1.1 for stages 1, 2, 3, 4, 5, and 6, respectively. These sizes are related to human lung deposition. The sampler was disinfected with 70% ethanol before sampling. The sampling time was 2 min for bacteria and 3 min for fungi to prevent overloading (i.e., >300 colony-forming units [CFUs] per plate). Each experiment was performed in triplicate.
Viable bacteria were collected on tryptic soy agar (TSA) (Merck, Germany) plates (90 mm in diameter), whereas viable fungi were collected on malt extract agar (MEA) plates (Merck). Bacteria samples were then incubated at 37 °C for 24 hr and fungi samples were incubated at 25 °C for 3 days before enumeration. Colonies were counted using a colony counter (model 570, Suntex Company). The count was divided by the volume of the air samples to determine microbial concentrations (CFU/m3).
Identifying Species via PCR Analysis
The colonies that had been counted in the third experiment on each sample type were used to identify the constituent species (genus). Colonies were first counted and categorized based on their morphological and color characteristics. The five most abundant colonies were transferred to new plates for purification and identification by a PCR procedure at a certified laboratory. The identification of bacteria was based on 16S rDNA primers whereas that of fungi was based on internal transcribed spacer (ITS) or 18S rDNA primers. The results were compared with the entries in the National Center for Biotechnology Information (NCBI) databank. The most likely (matching quality > 99%) genus was presented.
Data Analysis
Total and size-specific colonies from swine and chicken feces samples across all experiments were obtained. The areas under the CFU-time curve (AUC), determined using the linear trapezoidal methodCitation21 for total colonies and colonies of a specific size, were calculated from 0 to 40 hr because the test system failed after 40 hr with one chicken sample. Differences in the AUCs of total colonies between test animals were analyzed using Student's t test, whereas differences among colonies of six sizes were identified by performing an analysis of variance (ANOVA) with Tukey's HSD (honest significant difference) as a post hoc test. A value of P < 0.05 was regarded as statistically significant. The respirable fraction of the bioaerosols (i.e., aerosols with aerodynamic diameter of <4 μm)Citation22 was estimated from the colonies that were collected in the size ranges of between 3.3 and 4.7, between 2.1 and 3.3, between 1.1 and 2.1, and between 0.65 and 1.1 μm on the basis of the assumption that the number of bioaerosols collected in the size range of between 3.3 and 4.7 μm were distributed equally. The emission factors of culturable bioaerosols from chicken and swine feces were calculated using the following equation:
RESULTS
presents individual and overall means of the total numbers of colonies of bacteria and fungi that were collected from swine and chicken feces samples throughout the experimental period. The number of total fungal colonies did not generally increase until approximately 40 hr after the experiment began. The AUCs of total fungal colonies from the swine and chicken feces samples did not differ significantly (P > 0.05). Conversely, with respect to the chicken feces samples, the total number of bacterial colonies increased gradually in time, peaking at approximately 20 hr, and then remained stable to the end of the test. For the swine feces samples, the increase in the number of bacterial colonies over time was less than that of the chicken samples, as were the total number of colonies collected. Differences in the total number of bacterial colonies released over time, AUC, between the swine and chicken feces samples were significant (P < 0.05).
Figure 1. Total number of (a, b) fungal and (c, d) bacterial colonies released from (a, c) chicken and (b, d) swine feces throughout the experimental period.
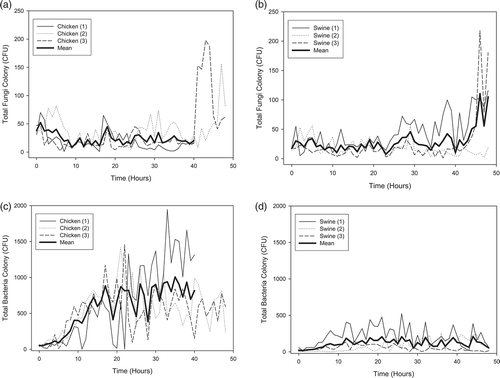
displays the mean number of bacteria and fungi colonies in the six size ranges throughout the experimental period. Most of the fungi were of in the size range between 2.1 and 3.3 μm (stage 4). The AUC for this size range was significantly (P < 0.05) greater than those of size of 7 or greater, between 4.7 and 7, between 1.1 and 2.1, and between 0.65 and 1.1 μm for swine samples and significantly (P < 0.05) greater than those of size 7 or greater, between 4.7 and 7, and between 0.65 and 1.1 μm for chicken samples. Conversely, most of the bacteria that were released from swine and chicken feces were in the size range of 0.65–1.1 μm (stage 6), and the corresponding AUC was significantly (P < 0.05) greater than those of the other size ranges.
Figure 2. Size-specific (a, b) bacterial and (c, d) fungal colonies released from (a, d) swine and (b, c) chicken feces throughout the experimental period.
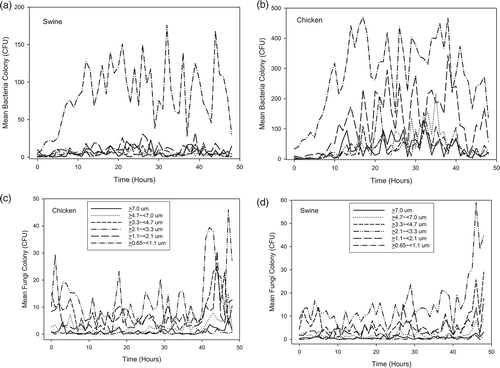
shows the size distributions, expressed as individual and grand means, for bacteria and fungi that were collected from three swine and three chicken feces samples. For swine and chicken feces samples, fungi of sizes between 2.1 and 3.3 μm had the greatest mean concentration, followed by those in size ranges between 3.3 and 4.7 μm and between 1.1 and 2.1 μm. However, for chicken feces samples, bacteria between 0.65 and 1.1 μm in size had the greatest mean concentration, followed by those between 1.1 and 2.1 μm in size, whereas for swine feces samples, the size range between 0.65 and 1.1 μm dominated.
Figure 3. Distributions of (a, c) fungal and (b, d) bacterial aerosol concentrations in six size ranges released from (a, b) chicken and (c, d) swine feces.
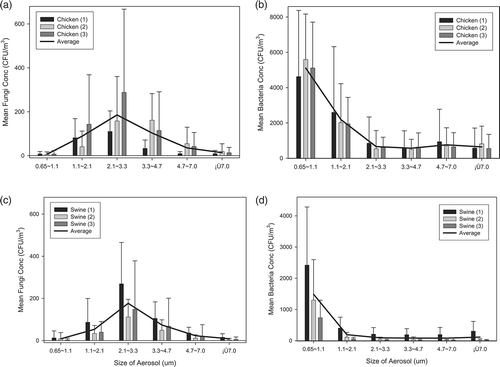
lists the results of PCR analysis for the most abundant bacteria/fungi genus that was released from swine and chicken feces. Genera Stenotrophomonas and Sphingomonas were the predominant bacteria in swine and chicken feces samples; however, their contributions (%) to the total number of colonies varied (39.3 and 33.5%, and 56.9 and 35.2% for swine and chicken feces samples, respectively). The genera Cladosporium (65.9%) and Acremonium (29.4%) represented the greatest proportions of fungi in chicken and swine feces samples, respectively.
Table 1. Identification of predominant bacteria/fungi emitted from swine and chicken feces
presents the emission factors (CFU/hr/g) of culturable bioaerosols from chicken and swine feces under current test conditions. Chicken and swine feces had comparable (P > 0.05) mean emission factors for total and respirable fungi—35 and 26.8 CFU/hr/g and 37.7 and 27.9 CFU/hr/g, respectively—whereas chicken feces had significantly higher (P < 0.05) mean emission factors for total and respirable bacteria (1284 and 1072 CFU/hr/g, respectively) than swine feces (259 and 228 CFU/hr/g, respectively). Mean respirable fractions (<4 μm) ranged from 76.6% (chicken) to 74% (swine) for fungi and from 83.5% (chicken) to 88% (swine) for bacteria.
Table 2. Emission factors of culturable bioaerosols (CFU/hr/g) from chicken and swine feces
DISCUSSION
Although the characteristics of microbial aerosols at swine/ poultry farms have been investigated extensively,Citation3,Citation4,Citation6,Citation14–16,Citation23,Citation24 most measurements have been made on air samples from inside of the facilities. Hence, analytical results can be utilized to assess overall contamination or worker exposure, but not to identify sources. In this investigation, environmental factors were controlled and the kinetics of the microbial aerosols that were released from animal feces, which is regarded as an important source of micro-organisms in swine/poultry production,Citation9,Citation17 was examined. Notably, because this study was carried out under controlled conditions, the results obtained may not be directly compared with field results for aerosols from various sources and with varying loadings.
Current experimental results indicate that the total number of bacterial colonies that were released from chicken feces increased gradually, peaking at approximately 20 hr, and then remained relatively constant until the end of the test, but no such initial increase in the number of bacterial colonies from swine feces was observed (). Chicken feces released significantly (P < 0.05, evaluated by AUCs) more (approximately an order of magnitude) bacterial aerosols than swine feces over 40 hr. The respective differences in the numbers of fungal colonies released from chicken and swine feces was small (30–40%) and insignificant (P > 0.05).
Notably, the feces were tested under undisturbed conditions, but in the field, chicken/swine are likely to disturb the manure, increasing the number of airborne particles. Field studies have also verified that swine/chicken farms that are equipped with slatted floors or systems that separate solid and liquid manure or remove feces regularly have better air quality than farms using conventional systems without separation.Citation9,Citation20 Therefore, feces, especially chicken feces, should be removed quickly to minimize bacterial aerosols.
Analysis of the size distribution of aerosols reveals that sizes between 0.65 and 1.1 μm were dominant for bacteria from chicken and swine feces, and the respective AUC significantly (P < 0.05) exceeded those associated with the other five size ranges (). For fungi, aerosols in between 2.1 and 3.3 μm were predominant, followed by those between 3.3 and 4.7 μm and between 1.1 and 2.1 μm. The AUC of fungal aerosols between 2.1 and 3.3 μm significantly (P < 0.05) exceeded those associated with all other size ranges, except for those between 3.3 and 4.7 μm and between 1.1 and 2.1 μm. Further analysis of the size-specific AUCs indicates that the mean respirable fractions (<4 μm) were 83.5 and 88% for bacteria and 76.6 and 74% for fungi in chicken and swine feces, respectively.
Emission factors of bioaerosols from livestock and poultry farms are important parameters in determining the risk of biohazard in the relevant areas.Citation5 A field study has estimated mean emission factors of 0.98 log(CFU)/hr/ pig for bacteria and 0.73 log(CFU)/hr/pig for fungi on the basis of mean microbial concentrations measured near the air outlet and mean ventilation rate of the facilities from swine houses.Citation8 However, source-specific emission factors of bioaerosols are hardly assessed. The current data indicate that chicken feces had significantly higher mean emission factors for total and respirable bacteria than did swine feces (1284 and 1072 vs. 259 and 228 CFU/hr/g, respectively), whereas chicken and swine feces had comparable mean emission factors for total and respirable fungi (35 and 26.8 vs. 37.7 and 27.9 CFU/hr/g, respectively) over the 40-hr test period. These data are useful in estimating the loadings of bioaerosols from feces.
Bacteria have been shown to be able to aggregate with other airborne particles to form larger particles, whereas most fungi are present as single particles.Citation23 However, current data () indicate that bacterial aerosols were present predominantly as single particles (≥0.65 to <1.1 μm). This result can be reasonably explained by the fact that the air in the test facility was relatively clean (free from other particles), and so the likelihood of aerosol conglomeration was low.
The results of species identification reveal that genera Stenotrophomonas and Sphingomonas were the two most abundant bacterial aerosols that were generated from swine and chicken feces, accounting for 72.8 and 92.1% of the total number of colonies, respectively (). Stenotrophomonas and Sphingomonas are Gram-negative germs and opportunistic human pathogens. Clark co-workersCitation14 found that airborne concentrations of total and Gram-negative bacteria in swine and poultry confinement units were as high as, or higher than, those found in other environments such as wastewater treatment plants. Another study of airborne bacteria in pig houses in TaiwanCitation6 identified high levels (∼10 times those in other areas) of Gram-negative bacteria (species not specified) in the air in finishing stalls, where mature swine reside in high densities and their waste typically remains on nonslatted floors for long periods. Conversely, other parts of swine houses (e.g., nurseries with slatted floors, where waste is regularly cleaned away) had significantly lower amounts of total culturable and Gram-negative bacteria.Citation6 Moreover, the genus Stenotrophomonas has been identified in samples of compost of swine manure.Citation24 Hence, swine feces seem to be a significant source of airborne Gram-negative bacteria in swine houses.
By contrast, the bacterium Staphylococcus has been identified as the dominant species in air samples from poultry farms and slaughterhouses.Citation25,Citation26 Similarly, genera Micrococcus and Staphylococcus, which are Gram-positive bacteria, have been identified as the predominant airborne bacteria in pig houses in TaiwanCitation4 and the United KingdomCitation27 and in factories for manufacturing feedstuff.Citation28 However, Staphylococcus was not a predominant bacterium in the emissions from current chicken and swine fecal samples, and Micrococcus represented approximately 0.3% of the total colonies that were emitted by chicken feces. Therefore, Micrococcus and Staphylococcus, which have been previously identified in the air of poultry/swine farms, likely originate from feedstuffs, not feces. This claim holds in particular for swine because the feed (mainly food wastes) is boiled before serving to reduce the number of microorganisms in the feed. The bacteria that are present in the feces samples were not measured in this study; however, the species from the feces that were identified in the air differ from those identified in the fecal biota in the literature.Citation29,Citation30 Therefore, future studies of biota in air and solid phase will be useful in understanding the mechanism of microorganism transferring.
The genus Cladosporium, the predominant fungus that is released from chicken (65.9%) and swine (13.4%) feces, is a common fungus in the natural environment and has been identified in 31% of poultry feed samples.Citation31 Furthermore, the genera Cladosporium, Penicillium, and Aspergillus were also identified as the predominant airborne fungi in a factory that manufactures feedstuffCitation28 and in some pig houses.Citation6 However, Cladosporium was not identified in another swine study.Citation16 Acremonium was the most abundant (29.4%) fungi in the swine fecal sample in the study presented here. This finding is consistent with a previous finding that Acremonium was the second-most predominant (7.6%) airborne fungal species during the composting of pig manure.Citation32 Thus, swine feces may be a source of airborne Acremonium in pig houses. Penicillium has also been identified from chicken and swine feces, accounting for less than 10% of all colonies. This fungus has been identified frequently in swine buildings, but not as a dominant species,Citation6,Citation15,Citation16 and it has been identified as a predominant airborne fungus in factories that manufacture feedstuff.Citation28 Although Penicillium may originate from feed, Penicillium, identified in the current fecal sample, may have also resulted from the poor sanitation of the humid and poorly ventilated pig house.
Various factors may also determine the amount of bioaerosol in an environment. For example, the temperature and RH of air statistically significantly affect the generation of bioaerosols within different indoor environments.Citation1,Citation5,Citation23 Biological particulates from solid and relatively dry surfaces can be released as individual cells or with other particlesCitation5; therefore, factors that affect the disassociation of bioaerosols from a matrix, such as air current (wind speed), may also be important. However, only common values of environmental parameters (30 °C, 65% RH, ACH of 1 hr−1, and an airflow of 0.01 m/sec) were set in this exploratory study. Nonetheless, chicken feces released significantly more bacterial aerosols than did swine feces (), consistent with the fact that the chicken feces had higher mean moisture content than the swine feces (77.5% vs. 66.9%). Hence, the relationship between the moisture of feces and bio-aerosol generation requires further elucidation.
CONCLUSIONS
Although bioaerosols in livestock and poultry farms have been identified as significant occupational health hazards, their sources are rarely identified. Swine feces have been regarded as a source of contamination because poorly cleaned finishing stalls with high swine densities have high levels of airborne bacteria. The emission characteristics of bioaerosols from swine and chicken feces must be identified before control measures can be implemented.
Chicken feces released approximately 1 order of magnitude more culturable bacterial aerosols than swine feces over 40 hr. Data on emission factors demonstrate that more than 1000 culturable bacterial colonies can be emitted from 1 g of chicken feces per hour for over more than 40 hr, and approximately 80% of these particles are smaller than 4 μm and are therefore respirable. Accordingly, feces should be removed as soon as possible. However, differences between the total numbers of fungal aerosols released from swine and chicken feces were small.
Most bacterial aerosols released from swine and chicken feces were Gram-negative opportunistic human pathogens, and the significance of their presence warrants further investigation.
ACKNOWLEDGMENTS
Partial financial support from the National Science Council (Taiwan, Republic of China, project no. NSC98-2815-C-241-004) is gratefully acknowledged. No competing interest is declared.
REFERENCES
- Dungan , R.S. 2010 . BOARD-INVITED REVIEW: Fate and Transport of Bio-aerosols Associated with Livestock Operations and Manures . J. Anim. Sci. , 88 : 3693 – 3706 .
- Oppliger , A. , Charrière , N. , Droz , P.O. and Rinsoz , T. 2008 . Exposure to Bioaerosols in Poultry Houses at Different Stages of Fattening: Use of Real-Time PCR for Airborne Bacterial Quantification . Ann. Occup. Hyg. , 52 : 405 – 412 .
- Kim , K.Y. , Ko , H.J. , Kim , Y.S. and Kim , C.N. 2008 . Assessment of Korean Farmer's Exposure Level to Dust in Pig Buildings . Ann. Agric. Environ. Med. , 15 : 51 – 58 .
- Donham , K. , Haglind , P. , Peterson , Y. , Rylander , R. and Belin , L. 1989 . Environmental and Health Studies of Farm Workers in Swedish Swine Confinement Buildings . Br. J. Ind. Med. , 46 : 31 – 37 .
- Cambra-Lopez , M. , Aarnink , A.J.A. , Zhao , Y. , Calvet , S. and Torres , A.G. 2010 . Airborne Particulate Matter from Livestock Production Systems: a Review of an Air Pollution Problem . Environ. Pollut. , 158 : 1 – 17 .
- Chang , C.W. , Chung , H. , Huang , C.F. and Su , H.J. 2001 . Exposure of Workers to Airborne Microorganisms in Open-Air Swine Houses . Appl. Environ. Microbiol. , 67 : 155 – 161 .
- Douwes , J. , Thorne , P. , Pearce , N. and Heederik , D. 2003 . Bioaerosol Health Effects and Exposure Assessment: Progress and Prospects . Ann. Occup. Hyg. , 47 : 187 – 200 .
- Kim , K.Y. , Ko , H.J. , Kim , H.T. , Kim , Y.S. , Roh , Y.M. , Lee , C.M. and Kim , C.N. 2007 . Monitoring of Aerial Pollutants Emitted from Swine Houses in Korea . Environ. Monit Assess. , 133 : 255 – 266 .
- Létourneau , V. , Nehmé , B. , Mériaux , A. , Massé , D. and Duchaine , C. 2010 . Impact of Production Systems on Swine Confinement Buildings Bioaerosols . J. Occup. Environ. Hyg. , 7 : 94 – 102 .
- Chang , C.W. , Chung , H. , Huang , C.F. and Su , H.J. 2001 . Exposure Assessment to Airborne Endotoxin, Dust, Ammonia, Hydrogen Sulfide and Carbon Dioxide in Open Style Swine Houses . Ann. Occup. Hyg. , 45 : 457 – 465 .
- Kim , K.Y. , Ko , H.J. , Kim , H.T. , Kim , C.N. , Kim , Y.S. and Roh , Y.M. 2008 . Effect of Manual Feeding on the Level of Farmer's Exposure to Airborne Contaminants in the Confinement Nursery Pig House . Ind. Health. , 46 : 38 – 43 .
- Attwood , P. , Brouwer , R. , Ruigewaard , P. , Versloot , R. , de Wit , R. , Heederik , D. and Boleij , J.S.M. 1987 . A Study of the Relationship between Airborne Contaminants and Environmental Factors in Dutch Swine Confinement Buildings . Am. Ind. Hyg. Assoc. J. , 48 : 745 – 751 .
- Preller , L. , Heederik , D. , Kromhout , H. , Boleij , J.S. and Tielen , M.J. 1995 . Determinants of Dust and Endotoxin Exposure of Pig Farmers: Development of a Control Strategy Using Empirical Modelling . Ann. Occup. Hyg. , 39 : 545 – 557 .
- Clark , S. , Rylander , R. and Larsson , L. 1983 . Airborne Bacteria, Endotoxin and Fungi in Dust in Poultry and Swine Confinement Buildings . Am. Ind. Hyg. Assoc. J. , 44 : 537 – 541 .
- Donham , K.J. , Scallon , L.J. , Popendorf , W. , Treuhaft , M.W. and Roberts , R.C. 1986 . Characterization of Dusts Collected from Swine Confinement Buildings . Am. Ind. Hyg. Assoc. J. , 47 : 404 – 410 .
- Cormier , Y. , Tremblay , G. , Meriaux , A. , Brochu , G. and Lavoie , J. 1990 . Airborne Microbial Contents in Two Types of Swine Confinement Buildings in Quebec . Am. Ind. Hyg. Assoc. J. , 51 : 304 – 309 .
- Nehme , B. , Letourneau , V. , Forster , R.J. , Veillette , M. and Duchaine , G. 2008 . Culture-Independent Approach of the Bacterial Bioaerosol Diversity in the Standard Swine Confinement Buildings, and Assessment of the Seasonal Effect . Environ. Microbiol. , 10 : 665 – 675 .
- Van Tongeren , M. , Van Amelsfoort , L. and Heederik , D. 1997 . Exposure to Organic Dusts, Endotoxins, and Microorganisms in the Municipal Waste Industry . Int. J. Ind. Med. , 33 : 384 – 391 .
- Douwes , J. , Dubbeld , H. and Van Zwieten , L. 2000 . Upper Airway Inflammation Assessed by Nasal Lavage in Compost Workers: a Relation with Bio-Aerosol Exposure . Am. J. Ind. Med. , 37 : 459 – 469 .
- Venter , P. , Lues , J.F. and Theron , H. 2004 . Quantification of Bioaerosols in Automated Chicken Egg Production Plants . Poult. Sci. , 83 : 1226 – 1231 .
- Matthews , J.N.S. , Altman , D.G. , Campbell , M.J. and Royston , P. 1990 . Analysis of Series Measurements in Medical Research . Br. Med. J. , 300 : 230 – 235 .
- Air Quality-Particle Size Fraction Definitions for Health Related Sampling , 1995 Geneva , , Switzerland : International Organization for Standardization . ISO 7708
- Górny , R.L. , Dutkiewicz , J. and Krysińska-Traczyk , E. 1999 . Size Distribution of Bacterial and Fungal Bioaerosols in Indoor Air . Ann. Agric. Environ. Med. , 6 : 105 – 113 .
- Guo , Y. , Zhu , N. , Zhu , S. and Deng , C. 2007 . Molecular Phylogenetic Diversity of Bacteria and Its Spatial Distribution in Composts . J. Appl. Microbiol. , 103 : 1344 – 1354 .
- Nielsen , B.H. and Breum , N.O. 1995 . Exposure to Air Contaminants in Chicken Catching . Am. Ind. Hyg. Assoc. J. , 56 : 804 – 808 .
- Haas , D. , Posch , J. and Schmidt , S. 2005 . A Case Study of Air-Borne Culturable Microorganisms in a Poultry Slaughterhouse in Styria, Austria . Aerobiologia , 21 : 193 – 201 .
- Crook , B. , Robertson , J.F. , Glass , S.A. , Botheroyd , E.M. , Lacey , J. and Top-ping , M.D. 1991 . Airborne Dust, Ammonia, Microorganisms, and Antigens in Pig Confinement Houses and the Respiratory Health of Exposed Farm Workers . Am. Ind. Hyg. Assoc. J. , 52 : 271 – 279 .
- Kim , K.Y. , Kim , H.T. , Kim , D. , Nakajima , J. and Higuchi , T. 2009 . Distribution Characteristics of Airborne Bacteria and Fungi in the Feedstuff-Manufacturing Factories . J. Hazard. Mater. , 169 : 1054 – 1060 .
- Lee , J.E. , Lee , S. , Sung , J. and Ko , G. 2011 . Analysis of Human and Animal Fecal Microbiota for Microbial Source Tracking . ISME J. , 5 : 362 – 365 .
- Salanitro , J.P. , Blake , I.G. and Muirhead , P.A. 1977 . Isolation and Identification of Fecal Bacteria from Adult Swine . Appl. Environ. Microbiol. , 33 : 79 – 84 .
- Labuda , R. and Tancinová , D. 2006 . Fungi Recovered from Slovakian Poultry Feed Mixtures and Their Toxinogenity . Ann. Agric. Environ. Med. , 13 : 193 – 200 .
- Cho , K.M. , Kwon , E.J. , Kim , S.K. , Kambiranda , D.M. , Math , R.K. , Lee , Y.H. , Kim , J. , Yun , H.D. and Kim , H.J. 2009 . Fungal Diversity in Composting Process of Pig Manure and Mushroom Cultural Waste Based on Partial Sequence of Large Subunit RRNA . Microbiol. Biotechnol. , 19 : 743 – 748 .