Abstract
Preclinical animal models of psychiatric disorders are of critical importance for advances in development of new psychiatric medicine. Regrettably, behavior-only models have yielded no novel targeted treatments during the past half-century of vigorous deployment. This may reflect the general neglect of experiential aspects of animal emotions, since affective mental states of animals supposedly cannot be empirically monitored. This supposition is wrong—to the extent that the rewarding and punishing aspects of emotion circuit arousals reflect positive and negative affective states. During the past decade, the use of such affective neuroscience-based animal modeling has yielded three novel antidepressants (i) via the alleviation of psychic pain with low doses of buprenorphine; (ii) via the amplification of enthusiasm by direct stimulation of the medial forebrain bundle); and (iii) via the facilitation of the capacity for social joy with play facilitators such as rapastinel (GLYX13). All have progressed to successful human testing. For optimal progress, it may be useful for preclinical investigators to focus on the evolved affective foundations of psychiatrically relevant brain emotional disorders for optimal animal modeling.
Los modelos animales preclínicos de los trastornos psiquiátricos son de gran importancía para el avance en el desarrollo de la nueva medicina psiquiátrica. A pesar del importante desarrollo durante la segunda mitad del siglo pasado, los modelos puramente conductuales no han dado origen a nuevos blancos terapéuticos. Esto puede reflejar el rechazo general a los aspectos experienciales de las emocíones en los animales, ya que los estados mentales afectivos de ellos supuestamente no se pueden monitorear empíricamente. Esta suposición es incorrecta, dado que los aspectos de recompensa y castigo que activan los circuítos de las emociones reflejan estados afectivos positivos y negativos. Durante la última década el empleo de estos modelos animales basados en la neurociencia-afectiva ha producido tres nuevos antidepresivos: 1) aliviando el dolor psíquico con bajas dosis de buprenorfina, 2) amplificando el entusiasmo por estimulación directa del haz medial del cerebro anterior y 3) facilitando la capacidad de goce social con facilitadores del juego como el rapastinel (GLYX-13). Todos han avanzado con pruebas exitosas en humanos. Para un desarrollo óptimo, podría ser útil para los investigadores preclínicos enfocarse en las evolucion adas bases afectivas de los trastornos cerebrales emocionales importantes en psíquíatría para generar óptimos modelos animales.
Les modèles animaux précliniques de troubles psychiatriques sont d'une importance cruciale pour les avancées dans le développement de nouveaux médicaments psychiatriques. Malheureusement, durant ces 50 dernières années d'essor dynamique, aucun nouveau médicament ciblé n'est né de modèles fondés sur le seul comportement. Cela traduit peut-être le fait que les expériences négligent généralement les émotions animales, les états mentaux affectifs animaux n'étant pas supposés contrôlables empiriquement. Cette hypothèse est fausse dans la mesure où les dimensions de récompense et de punition de l'excitation des circuits de l'émotion reflètent des états affectifs positifs et négatifs. Ces 10 dernières années, trois nouveaux antidépresseurs sont issus de modèles animaux fondés sur cette neuroscience affective: 1) en soulageant la douleur psychique par de faibles doses de buprénorphine ; 2) en amplifiant l'enthousiasme par stimulation directe du faisceau médian du téléncéphale ; et 3) en facilitant la capacité de joie sociale par le biais de facilitateurs de jeu tels le rapastinel (GLYX-13). Ils ont tous franchi avec succès les étapes jusqu'aux essais chez l'homme. Pour un progrès maximal, les chercheurs en recherche préclinique devraient peut-être s'intéresser aux bases affectives évoluées des troubles émotionnels cérébraux psychiatriquement appropriés pour une modélisation animale optimale.
Introduction
It is increasingly clear that the behavior-only modeling of psychiatric disorders, during the past half-century of intensive implementation, has yielded few new therapeutic strategies.Citation1 Why is this? Animal neuroscience has certainly been eminently successful in clarifying the neuroanatomies, neurochemistries, and neurodynamics of brain systems that are implicated in various behavioral models of anxiety (eg, via studies of fear conditioning)Citation2 and depression (eg, from imposition of various stressors).Citation3 However, most such models have deployed environmental threats such as foot shock, and various other stressors, with little explicit consideration of the emotional-affective brain circuits that engender negative feelings in both animals and humans. This reflects the prevailing tradition in animal behavioral research whereby the emotional feelings of animals are neglected (as experimentally unworkableCitation2). This review encourages movement to deeper psychobehavioral levels than is typical for the field: three novel therapeutics arising from cross-species affective neuroscience thinking (also discussed in a previous issue of this journalCitation4) are summarized.
The belief that scientists cannot illuminate relevant experiential (eg, affective) processes in animals goes back to Anglo-American behaviorism initiated by psychologists John WatsonCitation5 and Burrhus Frederic Skinner,Citation6, who asserted there were no rigorous scientific ways to evaluate the existence of animal minds. For instance, SkinnerCitation6 claimed that “The emotions are the fictional causes to which we attribute behavior.” Concurrently, European ethologists, while providing expert observations of diverse animal behaviors in nature, noted that their empirical approaches could not go to experiential levels: Niko Tinbergen,Citation7 in The Study of Instinct, famously asserted, “Because subjective phenomena cannot be observed objectively in animals, it is idle to claim or deny their existence.” Thus, 20th-century behaviorists and ethologists (who disagreed on many scientific issues) agreed that even if experiences exist in other animals, they are outside the realm of scientific inquiry. For instance, only recently one of Tinbergen's prominent students, Marian Dawkins,Citation8 working with great sensitivity on animal-welfare issues, noted that in animals “there is nothing to say that the emotion of fear has to be consciously experienced.”
This, in fact, has not been the case for the past 60 years, ever since Jim Olds and Peter MilnerCitation9 demonstrated that there are many types of rewarding (self-stimulation) sites throughout subcortical brain systems, and Pedro Delgado and colleaguesCitation10 found many subcortical sites where animals would escape similar fear-inducing deep brain stimulation (DBS). Of course, such neuroscientific approaches were essential to proceed to experiential levels in nonspeaking animals, but few followed that road. Those rewarding and punishing effects in animals (for an extensive summary, see ref 11) are reasonably interpreted as credible indices of various positive and negative affective states, especially since such DBS evokes positive and negative emotional feelings in humans.Citation12-Citation13 Still, to the present day, prominent neuroscientists claim that “We can never know whether another animal has conscious emotional feeling.”Citation2(p665) and “We will never know what other animals feel.”Citation2(p660) (present author's italics) without recognizing that such absolute opinions are not scientific assertions. Science never provides proof, but only the “weight of evidence” and various new “testable predictions,” and by that standard, emotional feelings in animals have been repeatedly demonstrated.
At present, a coherent working hypothesis, both logically as well as evolutionarily, is that animals, like humans, experience diverse subcortically mediated rewarding and punishing DBS-evoked emotional states of mind.Citation14,Citation15 What has prevented that from becoming accepted neuropsychological wisdom? Historical beliefs, passed down through generations before the maturation of functional neuroscience. One of the most influential beliefs was encapsulated in the famous James-Lange theory of human emotional feelings, which postulated that bodily autonomic arousals become emotional feelings only when they interact with higher cortical/mental processes. However, the ensuing century of research yielded little robust evidence for that reasonable conjecture.
Admittedly, some prominent neuroscientists still subscribe to corticocentric perspectives—for instance, that human emotional feelings arise from our higher-order syntactic thoughts (HOSTs), namely human language, as Edmund Rolls advanced in Emotion and Decision Making ExplainedCitation16 (see also Rolls,Citation17 with half a dozen critiques, one by the present author). Rolls' conjecture, if true, again restricts emotional feelings just to human brains and minds. However, that idea remains without evidence, although, as he discusses, higher emotional decision-making does surely require neocortex, since all primary-process emotional arousals survive radical decortication early in life.Citation15,Citation18 LeDoux and Rolls have left the scientific door (of differential predictions) open for other animals. In any event, abundant neurobehavioral evidence indicates that emotional feelings (probably homeostatic and sensory ones also) inferred from rewarding and punishing DBS—are widespread in the animal world, with evolutionarily conserved neuroanatomical and neurochemical controls. This allows us to model primal human emotional feelings by studying the brains of other mammals. Primal affective states are presumably intrinsic “survival value” indicators—just consider the utility of pain for survival—with species-typical variations, of course.
In sum, the cross-species affective neuroscience perspective is based on the remarkable fact that practically all DBS sites that evoke distinct emotional behavior patterns evoke rewarding (of SEEKING, LUST, CARE, and PLAY systems) or punishing states (RAGE, FEAR, and PANIC/GRIEF systems) in animals, and desirable or aversive ones in humans.Citation12,Citation13 This correspondence affirms that primal emotional feelings in humans arise from the same subcortical circuits as in animals.Citation15 Thus, humans have neither rewards nor punishments that they do not experience affectively, and there are no lines of evidence indicating that it is otherwise in animals.
Indeed, it has long been clear that many of the desirable and aversive effects of drugs arise from the same neurochemical systems in all mammals (as demonstrated by conditioned place-preference and operant reward paradigms). Such confluences led our group to develop explicit affectively guided cross-species strategies to study the neurochemical infrastructure of raw emotional feelings of humans by modeling homologous subcortical processes in other mammals, in the hope of developing better psychiatric therapeutics, especially antidepressants.Citation19-Citation22
Modern studies of human emotions
During the past half-century of human psychological science, the topic of emotional behaviors and feelings has grown from the 1970s onward with pioneers like Paul Ekman and Cal Izard,Citation23 with a concurrent neuroscience renaissance, increasing till the present, especially as functional brain imaging technologies became increasingly available. However, a critical issue to remember is that functional magnetic resonance imaging (fMRI) signals are rather ambiguous indicators of what is functionally transpiring in the brain, and very susceptible to the seesaw balance between emotional-affective arousals and cognitive processes.Citation21
Obviously, cognitive decision making can be strongly guided by affective states, but also disrupted by excessive emotional arousals. A serious problem is that emotional-affective shifts, controlled heavily by subcortical circuits, are very hard to resolve with fMRI-based brain imaging. Such imaging is more capable of detecting associated cognitive decision-making arousals in the cortex than the origin of associated affective states, although with some methodological savvy (eg, monitoring affective responses to emotional stimuli offline) there is fMRI evidence for subcortical origins of emotional affects.Citation24 Overall, positron emission tomography (PET) imaging is capable of much better resolution of the major loci of control for the origin of affective feelings ().Citation5
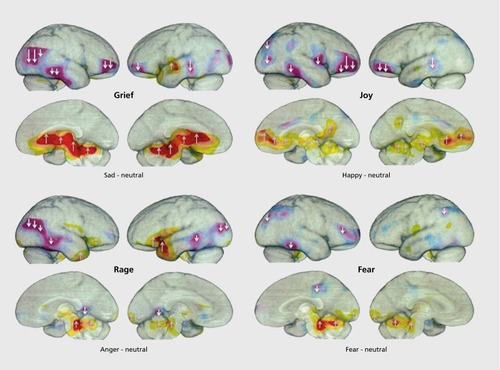
PET imaging in Figure 1 shows that all arousals (reds and yellows) in humans experiencing four basic emotions were subcortical, while many cortical areas exhibited diminished blood flow (purples). Indeed, these patterns correspond to brain regions implicated by studies with DBS induction of the four corresponding emotional/affective behaviors in animals (RAGE, FEAR, PLAY, and PANIC/GRIEF; for justification of capitalized nomenclature for primary emotional processes see below). Indeed, considering that our preclinical work had already highlighted the importance of sustained arousal of the psychological-pain-mediating PANIC/GRIEF system in instigating depression, it would be reasonable to consider using medicines that dramatically reduce this affective response in animals—namely, safe opioids (eg, buprenorphine) as well as potentially new oxytocinergic or prolactinergic agents (both are robust reducers of separation-distress calls in animal models)—as targets for antidepressant development. Indeed, it is known that human depression and sadness are accompanied by low-opioid states in the midline structures,Citation26 just where separation-distress PANIC calls are generated in animals.Citation15,Citation27
The general principle arising from the above human brain imaging and our animal brain mechanisms work is that the loci of control for prominent affective shifts in psychiatric disorders may originate subcortically, from primal emotional networks that engender raw emotional feelings in other animals (as indexed by rewarding and punishing properties of DBS). Why is this shift to affective issues important? This naturalistic perspective may allow our increasing understanding of animal emotional feelings to guide more evidence-based and hence better-targeted treatments for human affective disorders, especially depression, as discussed below.
Evolutionary levels of control within the brain and mind and the resulting semantic issues
Before considering the three new antidepressant treatments that our group has been involved in developing, it would be useful to detail the brain evolutionary and resulting semantic problems we must confront in clearly communicating issues where no standard scientific language exists for the psychological functions of the central nervous system (CNS) in animals: We have to deal somehow with the intrinsic linguistic ambiguities when we try to speak clearly and coherently about the experiential capacities of nonspeaking animals, as already discussed in a previous review for this journal.Citation4 Beyond standard neuroanatomical terminologies, we must consider the evolved behavioral and psychological functional “layers” of the brain and mind (). For simplicity, a classical tripartite analysis will be used, discussing primary, secondary, and tertiary processes successively as we ascend the brain-mind axis (each of which requires some clear nomenclatures for the functional scientific languages we use).Citation4,Citation28
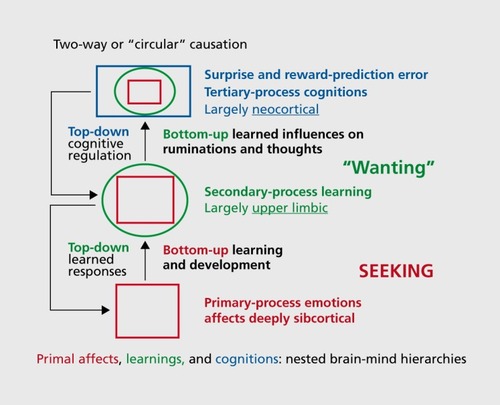
Thus, beside the difficult (hard to define) semantics of mind, what is equally problematic is that the brain is an evolutionarily structured organ (of course all bodily organs are), but it is of great advantage that the brain is the only organ where one can relatively clearly see the “layerings” of evolutionary progressions: What came first in brain-mind evolution remains situated more medially and caudally in the brain—thus, the brain stem emerged earlier in brain evolution than more rostral regions, with the medial and caudal brain regions being generally more ancient than lateral and rostral ones. Thus, in general, the upper brain stem (the mature hypothalamus and thalamus) evolved more recently than midbrain, which evolved more recently than the medulla, etc. In a sense, the functions of the primary-process levels (to deploy concepts with some sense of evolutionary layering) are instinctual—they are heavily (but not completely) functionally coded by the shared evolutionary history of vertebrates. It is important to recognize that the evolutionary layering of brain functions poses major challenges for the development of clear functional semantics for discussing neuromental abilities, and hopefully justifies our capitalization of the emotional primes—from SEEKING to PANIC, in presumed evolutionary progression, all of which connect up with higher cortices via the intervening basal ganglia, the secondary-process level where raw instincts interact with learning and memory mechanisms.
There are differences in species-typical details throughout the brain, but many specific functional solutions for living (on which we focus here) are more highly conserved in the brain stem (home for primary processes) than within the more encephalized tertiary-process cortical layer of the brain, where few of the final functions are coded by evolutionary selection, but by the learning and memory specialization of the basal ganglia. Indeed, based on modern genetics and various functional studies, we can provisionally conclude that no specific psychological function has been evolutionarily “learned” within the vast random access memory (RAM)-type neural “fabric” of neocortical networks. In other words, at birth, neocortex remains a very “open” system where all functions are developmentally programed, a view necessitated by various lines of evidence. For instance, we know that even the capacity for highly resolved neocortical vision is learned, in the sense that if you don't use it you lose it—eg, David Hubel and Torsten Wiesel'sCitation29 demonstration of complete loss of sight in kittens when one set of eyelids had been sutured shut soon after birth. Equally compelling is the demonstration of Mriganga Sur's groupCitation30 that removal of all occipital tissue that would normally become ”visual cortex“ in prenatal mice led to no visual deficits at maturity; the surviving adjacent cortex ”welcomes“ inputs from the lateral geniculate body of thalamus, thereby laying down visual competence in surviving neocortical regions that would have otherwise been programmed for other functions (eg, nearby auditory and somatosensory sensitivities).
There are many other relevant lines of evidence for this generalization, perhaps the most recent being the likelihood that the massive expansion of neocortex in the human brain was controlled by a single gene variant which did exist in Neanderthals and Denisovans, but not in our surviving “great ape” cousins.Citation31 Since neocortex is constructed from self-similar columns (resembling empty RAM chips) much of mammalian brain expansion was guided by the simple rule of “more of the same please.” In short, there is very little evidence for genetic programming of higher mental functions in humans (above that found in other animals)—namely, functions such as our superb linguistic and related cognitive abilities were probably all learned (developmentally programmed by primary-process affects and unconscious learning-memory mechanisms)—a mental sophistication that emerges developmentally from having so much available empty RAM space. It is through the reprogramming (perhaps unraveling) of these higher acquired mental representations that most psychotherapies probably work.Citation32,Citation33 In contrast, new medicines for mood disorders that will more effectively re-establish mind states that facilitate effectiveness and satisfaction with living will probably need to more directly impact lower brain-mind affective and learning/memory functions, especially emotional ones (rather than homeostatic and sensory ones—for clarifications see ref 4, Table I)
For our purposes here, we envision a middle level of secondary processes nestled in intermediate “limbic-brain” areas: from diverse “basal ganglia” structures (amygdala, nucleus accumbens, etc) where raw affective experiences arising from below (from upper brain stem primary-process structures, as highlighted in Figure 1)—where instinctual behaviors and feelings come to be accommodated/adjusted to world events—yield diverse memories that are essential for thinking and decision-making (namely the diverse higher cognitive tertiary processes for refined/complex actions based on external information processing). This synopsis of hierarchical brain-mind controls can be encapsulated in a conceptual vision of “nested hierarchies” that explicitly recognizes that what came earlier in brain-mind evolution (eg, primary affective processes) developmentally controls what came later. In this view, the cardinal behaviorist construct of “reinforcement” of learning and memory is naturalized into the way the neurochemistries of intrinsic primary-process affective values, arising from middle and upper (mesencephalic and diencephalic) brain stem emotional-action networks, are critical components for stimulus-response learning and instrumental memory consolidations. Those memory mechanisms are ultimately manifested in our neocortical capacity to have complex perceptions and plans—namely, to think.Citation20,Citation33
From this bottom-up neuroevolutionary perspective of developmental-functional hierarchies, not only were various primal positive and negative affects among the first kinds of mental experience to exist, but the neural infrastructure of the brain's various affective systems are critical for the emergence of higher forms of consciousness, reflected in what animals have learned and remembered.Citation20,Citation28,Citation33 In other words, the first affective glimmers of consciousness may still permit higher-order forms of consciousness and decision-making to emerge from neocortex. Also, because of the recursive nature of the brain's nested hierarchies, with an initial bottom-up developmental emergence, top-down control of raw experiences and behaviors typically emerge with maturation. Thus, not only are primal affective systems important for rapid instinctual decision-making (constituting adaptive unconditioned responses), but they control conditioning and, ultimately, the qualities of our cognition. It is certainly possible, even likely, that the progression of primary processes in the construction of the upper mind is also able to develop a variety of learned affective-behavioral states that complexify the emotional feelings of homo sapiens beyond anything experienced by other species (eg, as exemplified by our various arts). However, we should also be willing to keep such options, in simplified forms, open for how higher mental abilities emerge in other animals as well. Unfortunately, good constitutive neuropsychological science on such developmental topics is hard enough to do with humans (requiring sophisticated psychological analyses along with brain imaging), and the task is surely much harder in other species, since interspecies cognitive communication, to put it mildly, remains scientifically difficult. A key problem in discussing such issues is that we currently have no agreed-upon functional languages for these higher levels of brain-mind analyses, so critical for understanding the phenomenology of psychiatric disorders, suggesting we may need to cultivate new neuropsychoanalytic perspectives.Citation33,Citation34 Conversely, good constitutive research on the primary-process emotions is very hard to do in humans, but considerably easier in animal models.Citation15,Citation20 That is what the capitalization of emotional primes is all about—a first pass at functionally labeling primary-process brain affective systems in ways that can be deployed in cross-species research, thereby promoting better modeling of psychiatric disorders.
For those who might still doubt the intrinsic affective/psychological powers of mammalian subcortical brain regions, let me share an experiment carried out in the author's lab in the mid-1980s when studying the play of decorticate rats (published much later; see Panksepp et alCitation35). There were 16 students in an affective neuroscience laboratory class, where beside lectures, they had 14 laboratory practicums on brain and behavior using laboratory rats. In the first week of the semester, I prepared two animals for each student: one in which the neocortex had been aspirated away to give a clear view of the structures below (eg, basal ganglia, septal area, and hippocampus; see ref Citation15, p292, Figure 15.6) and one control rat that also at 3 days of age received full surgery, but with brain left intact. During the final practicum, all students received a pair of such animals to observe individually, as they wished, for half an hour, with the assignment of deciding which animal had been decorticated. I expected random choices, but was surprised (and ultimately delighted) to find that 12 of 16 students chose their decorticate rat to be the neurologically intact, normal one (a P<0.05 mistake). In debriefing, a common theme was that students who chose decorticates to be intact, chose on the basis of which animal explored more—the one seemingly more highly interested in the world—a manifestation of the highly “rewarding” subcortical SEEKING (enthusiasm) system.
Of course, it has long been known that decortication (as well as restricted septal disconnection damageCitation22) releases emotionality in animals (eg, in cats, usually manifested by “decorticate rage”), and in young rats this was expressed as “curiosity”—a disinhibited SEEKING/foraging/enthusiasm system in action, or so I surmised. Please also note that DBS induced “reward” and “aversion”—our primary validated measures of affect in animals—remain operational in decorticate animals. This is an old lesson, but perhaps too neglected in modern psychiatry: Our primal mammalian emotional powers are all subcortically concentrated and perhaps of critical importance for development of better psychiatric therapeutics (perhaps for understanding consciousness itselfCitation15,Citation18,Citation34). In any event, the thesis here is that cross-species affective neuroscience research is critical for decoding the neural nature of our own primal emotional feelings and hence development of new therapeutics for human affective disorders.
In sum, we have long known that the subcortical primary-process brain functions are essential for the continued functioning of our higher brain-mind apparatus. If one damages the largest of the emotional systems—SEEKING—by bilaterally lesioning the medial forebrain bundle (MFB) (), Citation36 animals are psychologically crippled for life. Apparently, higher cognitions can't exist without the diverse lower brain functions that include unconditional affective potentials, while lower brain-mind functions survive destruction of upper ones.Citation15,Citation34 Perhaps the easiest experiences of human minds to be decoded neuroscientifically will be the primary-process valenced states of the brain, especially emotional affects, with hopefully homeostatic ones, especially HUNGER (so important for developing truly effective appetite control agents), next in line.
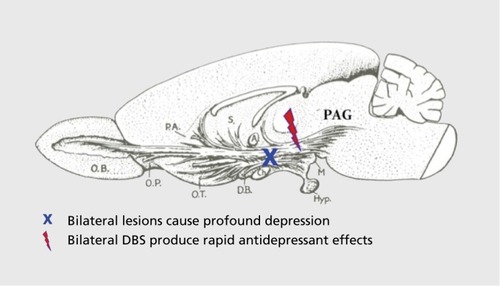
Because the primal affects are shared evolutionary solutions for living in all mammals, subcortical emotion research in other animals may be able to reveal the neural nature of core human affective processes more readily than any other strategy currently available. Comparable cross-species feats have already been achieved in endocrinology, physiology, and genetics. Indeed, with that aspiration, I shifted from clinical psychology training to physiological psychology and brain research in 1965, in the hope of helping decode human emotional feelings. I did not anticipate that the radical-behaviorist juggernaut, so useful for rigorous studies of learning and memory (with a general neglect of the nature of unconditioned emotional-affective responses), would become a major obstacle. But that is gradually changing. The rest of this paper will summarize our thinking about three possible antidepressant strategies that have arisen from our affectively focused research program. However, before proceeding, I would briefly re-emphasize the linguistic difficulties that confront us when we seek to pursue an understanding of primal human affective-experiential states in other animals that cannot speak, especially when there is no agreed-upon scientific language for such processes across species. For progress, we need standard nomenclatures, especially for the genetically controlled emotional powers of animal minds.
The “affective turn” and the semantics of emotions: conceptual conundrums
So how shall we talk about the emotional feelings of other animals? Just as positive or negative? Or desirable or aversive? That may be too general if affects come in diverse varieties. Even though there are clear evolutionary relationships among the neuroanatomical and neurochemical organizations of all mammalian brains, human languages are so rich with ambiguities that vernacular terms commonly used to discuss human feelings could easily lead to confusions when applied to nonspeaking animals. The deployment of vernacular affective terms common in human languages opens up enormous potential for confusions, since they have no consistent CNS reference points. Accordingly, the field needs specialized scientific-descriptive/functional terminologies for the primary-process homologous emotional systems that all mammals still share because of shared evolutionary descent, a terminological convention that captures the unconditional categorical function of the systems without asserting that they are identical across species (gene polymorphisms—different allelic forms of a gene—may be an apt illustrative metaphor).
In short, what functional terminology could be developed where none exists? I eventually selected full capitalizations for officially labeling the seven DBS-identified brain emotional systems, four of which yieided self-stimulation (hence positive valence), being called SEEKING, LUST, CARE, and PLAY (with surely distinct, but perhaps overlapping, evolutionary affective attributes). Those that were punishing were labeled RAGE, FEAR, and PANIC/GRIEF systems. Distinct positively valenced systems were proposed since it has long been clear that DBS of affectively overlapping, but behaviorally specific, subcortical regions in all mammals can provoke distinct emotional-behavioral arousals, which in the vernacular might be called (i) curiosity/interest/enthusiasm, as manifested by an exploratory drive (SEEKING); (ii) male and female sexual arousal, as monitored by copulatory behaviors (LUST); (iii) maternal nurturance (CARE); and (iv) PLAYfulness (all four systems course through the MFB [Figure 3]), and are rewarding, with a diversity of inputs and outputs (). Citation15,Citation37 In contrast, other nearby brain sites yieided behaviors indicative of (v) anger (RAGE); (vi) anxiety (FEAR); and (vii) separation-distress-anxiety (PANIC/GRIEF). All of those brain sites were aversive (punishing).
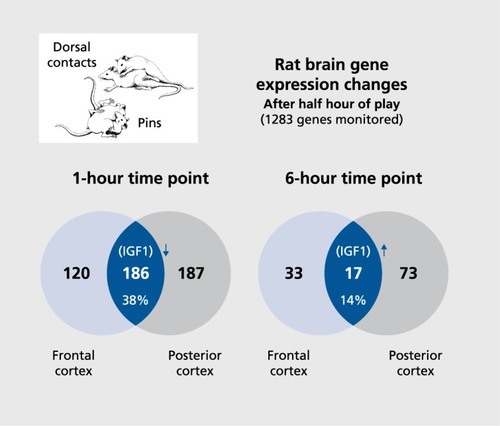
All such behavior tendencies were evoked with DBS of subcortical brain regions; no sites were found in neocortex. The capitalization promotes clarity with regard to which brain/mind processes are being discussed, and will be used here as described more fully elsewhere.Citation18-Citation21 This nomenclature informs readers that specific brain emotional/affective systems are being designated.
It is noteworthy that there is abundant data that the brain regions in which primal emotional systems are concentrated are essential for consciousness in higher brain regions.Citation15,Citation34 There are many reasons to believe that neural systems that control affective shifts participate in the brain's learning mechanisms, and also perhaps higher cognitive decision-making that emerges from neocortical functions.Citation21,Citation24 Namely, behavioral data has long indicated that unconditioned stimulus and response circuits (both reflecting genetically inborn “values” of the nervous system) are essential for conditioning to proceed. Animals also surely experience other types of affective states such as homeostatic imbalances (eg, HUNGER and THIRST) as well as diverse sensory affects (various PAINs and PLEASUREs). Although those types of feelings are less important than emotional ones for understanding psychiatric disorders, they are critical for developing better medicines for various bodily problems, such as obesity. The fact that the experiential concept of HUNGER is not more widely studied in preclinical feeding and energy-balance research has surely contributed to the dearth of effective medicines that have emerged from animal feeding-behavior research.
Models of depression: a turn toward understanding the relevant negative and positive affects
It is understandable why the emotional feelings of animal brains were empirically neglected by scientists: it required neuroscientific analysis. Regrettably, many behaviorally oriented scientists still believe such states may not exist in animal brains, or that if they do, they cannot be empirically studied. To reiterate, such ultra-skeptical views are simply a residue of reasonable preneuroscientific restrictions. The discovery of rewarding and punishing properties of subcortical DBS could have reversed that in 1954,Citation9,Citation10 but understandably, that conversation was not engaged by behaviorists during their height of power. More surprisingly, the conversation did not change when “nevermind” behaviorists flooded into physiological psychology (my chosen field) in the late 1970s, a time when a key journal of the era, the Journal of Comparative and Physiological Psychology, was renamed Behavioral Neuroscience (in 1981).
However, the relevant facts have been available for a long time. With the discovery of brain reward and punishment, we had empirical evidence that animals do, in fact, experience desirable and undesirable states of mind,Citation9,Citation10 and human data has provided relevant semantic self-reports.Citation11-Citation13 A solid understanding of brain emotional systems has the potential to reconstruct the scientific foundations of psychiatry,Citation32,Citation33 eg, promoting the discovery of new treatments of affective disorders that accompany many mental illnesses. Here, I focus on our research and clinical thinking about three of the relevant systems: (i) the neurochemistry of the PANIC system, namely separation-distress (in the vernacular, feelings of sadness/grief), as a major affective pathway that promotes depression,Citation38,Citation39 and (ii) PLAY, whose power to facilitate positive social engagements and promote feelings of playful joy, may guide identification of neurochemical pathways to promote feelings that could counteract depression,Citation39-Citation41 as well as (iii) facilitation of SEEKING, a key energizer of our exploratory-investigatory urge, which engenders feelings of enthusiasm, and which is depleted in the dysphoria of depressive disorders.Citation38 The clinical implications of these ideas are currently being evaluated.Citation40,Citation41
Thus, the ideas guiding our attempts to identify new treatments for depression have been straightforward. Firstly, depression is promoted by elevated arousal of the PANIC system, because this “loneliness/sadness” type system, at its most intense, promotes what is commonly called the “psychic pain” of social loss, which can readily be reversed with “safe opiates” such as buprenorphine that stimulate mu-opioid receptors, promoting affective homeostasis. Such treatment is thereby a potentially life-saving maneuver, as in suicidal cases. Similar work could be pursued with the safer commercially available analgesics, such as tramadol and tianeptine, which also increase synaptic norepinephrine and serotonin. Secondly, natural playfulness is facilitated by neurochemistries of joy, which if gently promoted, may counteract depressive affects; this strategy led to the selection of a target molecule called rapastinel (GLYX-13) as a potential antidepressant (currently in successful human testing). Finally, stress-induced diminution of the resources of the dopamine-driven SEEKING system (the so-called “brain reward” system) has now been envisioned to be deficient in depression by many groups. Our approach encourages therapeutic DBS of MFB to reverse chronic dysphoria.
Overall, these new and direct affective approaches have now provided three new therapeutic strategies that highlight the utility of focusing on relevant affective processes in animal brains to guide ways to counteract depressive negative affective feelings in human brains. A synopsis of these strategies, as also described in more detail elsewhere, will be provided below.Citation40,Citation41
First hypothesis: the “psychic pain” and resulting depressive feelings promoted by the separation-distress PANIC system may be counteracted by safe opioids such as buprenorphine
John Bowlby's early theoretical workCitation42 promoted the idea that excessive social separation led to insecure parental bonding, setting up the nervous system for future depression. In the 1970s, we initiated comparative neuroscientific analysis of separation distress (aka PANIC): Our pharmacological work highlighted the truly remarkable power of opioids to reduce this type of emotional arousal. Initial canine studies highlighted efficacy as low as 0.1 mg/kg with 0.25 mg/kg almost eliminating separation calls in young beagles.Citation43 These robust opioid effects were replicated in guinea pigsCitation44 and newborn chickens,Citation45 and extended to a large variety of social behaviors.Citation45 Of pharmaceuticals and neuropeptides evaluated, the only ones beside opioids that were as robust in reducing separation-distress vocalizations were oxytocin and prolactin.Citation27
It is widely accepted that excessive early separation distress can lead to insecure parental bonding, providing an endophenotype that is especially susceptible to future depression. From this perspective, it is no surprise that prior to the modern era of psychopharmacology, the only highly effective medicinal ways to reduce depressive affect were opioids, and hence morphine was commonly used to treat depression in the 19th and first half of the 20th century.Citation46 Indeed, Emil Kraepelin provided explicit advice on how to use morphine for depression, with substantial care taken to minimize addiction. Indeed depression and low positive affect are, in part, low-brain-opioid states.Citation26 The likelihood of traditional full-agonist opioids, all of which are highly addictive, ever being part of modern psychiatric practice is remote. However, being a partial agonist of mu receptors, buprenorphine is more likely to receive acceptance, especially since it also has useful kappa-opioid-receptor antagonist properties—a major target for current antidepressant drug development (Box 1).
Still, from a cross-species affective neuroscience perspective, there have long been substantial reasons to formally evaluate low-dose buprenorphine efficacy in depression, especially for individuals who have not responded well to other medications: (i) very low doses of all mu-opioid receptor agonists tested so far robustly reduce negative affects of separation-distress (PANIC arousal) in animal models, thereby promoting positive social affect that is missing in chronic dysphoria; and (ii) low doses of mu agonists promote the positive social joy of playfulness.Citation15 Buprenorphine is “safe” because of its “partial agonist” properties, where activation of mu-opioid receptors is only promoted at modest doses, dramatically reducing problems associated with respiratory depression (albeit with the relatively minor side effect of elevated nausea evident in a small fraction of individuals). Thus, at ultra-low doses, buprenorphine is safe, and since “psychic pain” of depression may normally be due to low-opoiod activity in relevant brain emotional systems, the potential benefits of ultralow-dose buprenorphine, in pure form, especially in treatment-resistant depression, clearly deserves more thorough evaluation. Since several open trials have reported dramatic antidepressant effects with low doses of buprenorphine,Citation46,Citation47 we initiated plans to conduct an adequately powered double-blind placebo-controlled (DB-PC) trial for depression, as well as suicidal thoughts, with comparably low doses of buprenorphine.
After several failed attempts to get such a study completed in the United States, in 2009, Yoram Yovell, a distinguished psychiatrist in Jerusalem, agreed to supervise such a study. His group has successfully carried out the project (with 40 depressed people and 20 controls): the buprenorphine medication (initial dose = 0.2 mg/day sublingually, gradually increased to 1.6 mg/day in divided doses) was given to depressed patients as an adjunct to ongoing treatments. Although the placebo effect after 1 week was substantial, by the second week the buprenorphine had significantly greater benefits than placebo, and this effect continued to grow across the subsequent 6 weeks of testing. As of this writing, pilot work and general summaries have been noted in a few review papersCitation40,Citation41 and the full study is currently in press.Citation48 In any event, beside a substantial reduction in depressive symptoms, as measured with the Beck Depression Inventory, suicidality monitored with the Beck Suicide Inventory was even more substantially reduced. Since there are no robust medications to reduce suicidality, this finding is of potential life or death importance. For instance, the deployment of this strategy may help quell the current suicide epidemic among wounded and shell-shocked soldiers being cared for by the Veterans Administration in the United States.
Considering the resistance against the use of buprenorphine in psychiatry, it may be appropriate to bring up a relevant sociopolitical issue: A substantial part of clinical depression is brought on by excessive social isolation and the resulting chronic loneliness, resulting in sustained psychological distress/pain that may emerge, in part, from diminished opioid tone in the brain. Also, it should be clear that at least a part of opioid addiction arises from self-medication for depressive affects. Without recognition of this dimension of opiate abuse, draconian laws have often worked at cross purposes with the need for better treatment practices (and education) to reduce human misery. There needs to be a better recognition in society that brain-opioid activities help mediate practically all the simple everyday pleasures of life, from good food and warmth to social joy and sexual gratification. Had a scientifically based conversation about the natural function of brain opioids been pursued in the 1970s (eg, that brain opioids control the psychological pain of social loss, namely separation distress) and disseminated in society, more countries may have avoided social incarceration policies that promoted rather than reduced human misery during the past half-century. Thus, the fact that there remains substantial resistance to the careful use of safe opioids in psychiatry may reflect sociopolitical forces (no doubt economic ones also) more than sensible medical concerns. In other words, if an old, off-patent medicine works better than most currently patented agents, resistance may also reflect economic considerations rather than medical/scientific ones. It will be most interesting to see if the amplification of kappa-antagonist effects of buprenorphine as achieved with ALKS-5461 (achieved by buprenorphine at the relatively high 2- to 8-mg range, combined with the mu-opioid receptor antagonist samidorphan [ALKS-33]) will be different than the implementation of buprenorphine's mu-agonist effects at much lower doses and yield different patterns/spectra of antidepressant effects (Box 1).
Box 1
It is currently well appreciated from preclinical work that kappa-opioid-receptor antagonists are likely to be excellent antidepressants. Indeed, this aspect of buprenorphine can be maximized if the mu-opioid-receptor agonist, and hence potential addictive effects, of buprenorphine could be diminished with a dual-agent medicine whereby the kappa-receptor-blocking effect of buprenorphine could be maximized, while the mu-receptor effects are minimized with a proprietary mu-opioid-receptor antagonist. These two properties should yield a completely safe buprenorphine-based antidepressant that maximizes kappa-antagonist effects. Indeed, pharmaceutical firm Alkermes has been developing such a new buprenorphine-based antidepressant, which, combined with their proprietary mu-receptor blocker samidorphan (ALKS-33) allows the novel Alkermes medication to block the potential mu-receptor-mediated addictive potentials of buprenorphine, while maintaining kappa-receptor-blockade-instigated antidepressant effects (for description and relevant references see https://en.wikipedia.org/wiki/ALKS-5461). This recipe reflects the abundant preclinical evidence indicating that the dysphoria of depression is promoted by excessive brain kappa-opioid-receptor activation. By blocking mu-opioid effects with ALKS-33, the kappa-receptor-antagonism effects of buprenorphine have been optimized to provide the novel antidepressant code-named ALKS-5461 (for further information, see http://investor.alkermes.com/phoenix. zhtml c=92211&p=irol-newsArticle&ID=2003398, as well as http://mentalhealtlidaily.com/2014/08/05/new-antidepressant-alks-5461-trials-2016-expected-availability/).
Second hypothesis: depression may arise from diminished capacity for social joy
A second example of our affective-neuroscience approach to developing new antidepressant therapies has arisen from our study of rodent PLAYCitation49 We initiated formal experimental analyses of play through the simple approach of deploying short periods of social deprivation to amplify the urge of juvenile rats to exhibit rough and tumble physical PLAY and thereby bring it under experimental control.Citation50 After extensive analysis of various environmental and bodily variables, we discovered an approximately 50-kHz ultrasonic vocalization (USV) that seemed to have measureable properties suggestive of a laughter-joy-type process.Citation51 We developed a simple “tickling” assay to phenotype animals in terms of their capacity for social joy. This led to a breeding program where high chirpers were bred with each other, along with a comparable group of low chirpers.Citation52 After four generations of selective breeding, these lines of animals were well separated in terms of our psychobehavioral assay of positive affect.Citation53 DBS mapping of the brain for such USV indicated that most sites were situated along the MFB and sustained self-stimulation reward; namely, each animal would press a lever to obtain electrical stimulation of essentially all 50-kHz USV evoking sites in their brainsCitation54 (our interpretation was that such DBS produced an unconditioned positive hedonic state akin to social joy robustly exhibited during social PLAY activities). Additional behavior genetic studies indicated that animals exhibiting abundant 50-kHz chirps were more resistant to depressogenic stressors than control animals, while those bred for low 50-kHz USVs were more prone to depressive behavior patterns.
These findings encouraged us to seek novel joy-promoting neurochemistries within the brain, using standard microarray gene-expression analyses. Briefly, of roughly 1200 genes evaluated, about a third were significantly over- or underexpressed in anterior and/or posterior neocortical brain regions 1 hour after half an hour of social PLAYCitation55 This, of course, yielded too many candidates for subsequent functional studies. So another cohort of animals was sacrificed 6 hours after half an hour of purely positive PLAY (as monitored by the abundance of 50-kHz ”chirps“ and absence of 22-kHz ”complaints“). Gene-expression measures at 6 hours post-play diminished down to about 10% of the 1-hour levels, with 33 genes exhibiting significant changes in anterior-frontal executive/motor cortical regions and 72 genes showing changes in posterior sensory/perceptual regions, with only 17 being changed in both regions (Figure 4). Two of those 17 genes—namely, the gene coding for the insulin-like growth factor 1 (IGF-1) and the glutamate sensitive N-methyl-D-aspartate receptor subtype 2B (NMDA NR2B)—were prioritized as optimal candidates for development of new antidepressive therapeutics, as evaluated for capacity to elevate positive-affect-indicative emission of 50-kHz chirps.Citation55,Citation56 Indeed, both were found to elevate our validated 50-kHz USV measure of positive affect, but since IGF-1 can promote tumor growth, only glutamate facilitation was targeted for medicinal development. Of course, imbalanced glutamate transmission has long been implicated in depression,Citation57 and our preclinical emotion work had long indicated that glutamate is a primary transmitter in practically all emotional responses.Citation58 Indeed, our group at the Falk Center for Molecular Therapeutics (Northwestern University) had already identified and purified a novel glycine-receptor partial agonist (the aforementioned GLYX-13, a tetrapeptide, threonine-proline-proline-threonine, that has to be administered intravenously) that gently promoted glutamatergic transmission at low doses (but became an antagonist at high doses, in a manner akin to D-cycloserine).Citation57,Citation59
Preclinical work affirmed potential efficacy in our affective models, facilitating 50-kHz USVs as well as learning and memory.Citation55,Citation57 GLYX-13 also easily passed animal toxicity testing (no lethality, including at very high doses), as well as US Food and Drug Administration (FDA) Phase 1 human safety evaluation. Positive results in FDA Phase 2A human proof-of-concept DB-PC evaluation for antidepressant effects in humans have been successfully conductedCitation60: Subjects receiving single intravenous doses of 1, 5, or 10 mg/kg all exhibited antidepressant effects, with the two higher doses exhibiting statistically significant benefits within a day after administration, and significant benefits lasting for a week. The highest dose exhibited no benefits, as expected from a partial agonist profile. Those promising results are currently leading to sustained efficacy evaluation of GLYX-13 as not only an antidepressant, but eventually as a cognitive enhancer that may facilitate efficacy of psychotherapy by intensifying reconsolidation of beneficial memories.Citation32 There are reasons to believe this cognitive facilitator may have benefits in other disorders, perhaps even autism.Citation61
Third hypothesis: reduced SEEKING activity can diminish enthusiasm for life
The “brain reward system” concept has been with us since 1954, ever since James Olds and Peter Milner discovered self-administered DBS in animals (for full summary, see ref 11). The most robust “self-stimulation” effects were obtained from practically all regions of the MFB (for earliest depiction, see Figure 3; for diverse neurochemistries, see Panksepp and HarroCitation62) that courses upward from the ventral tegmental area (VTA) situated at the midbrain-hypothalamic transition, distributing neural influences, especially dopamine release, to various terminal regions such as the nucleus accumbens and further up into orbitofrontal and medial frontal cortical regions, long implicated in emotional processing (for a more comprehensive summary of the connectivity, see (). The evoked feeling has been commonly assumed to reflect a vaguely defined “pleasure” category, but the possibility that the feeling resembles any sensory pleasure known to humans seems remote. Based on ethological changes during relevant rat DBS studies, namely induction of intense exploration and foraging, accompanied by forward locomotion and sniffing, we have called this massive, affectively positive “brain reward” emotion circuitry the SEEKING system to better convey the affect of “enthusiasm” that this system mediates.Citation14,Citation15,Citation20
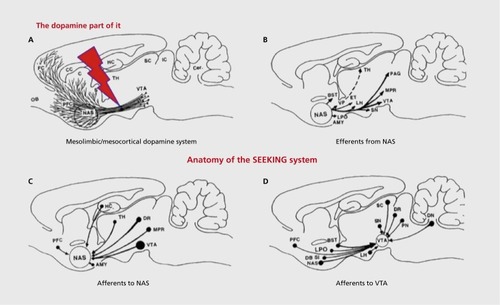
There is now abundant evidence for the somewhat surprising hypothesis that this system mediates positive affective feelings organisms experience as they are enthusiastically engaging their environments in search of all resources needed for survival. Still, the old “brain reward system” nomenclature remains most commonly used, although newer concepts have been emerging for a long time (See Figure 2, for three major variants, with primary- [SEEKING], secondary- [“wanting”] and tertiary-process [“reward prediction error”] perspectives). It has also long been known that damage to this system produces a global depressive phenotype: animals become sluggish and stop exploring and self-grooming. With large bilateral lesions, they often stop eating and drinking, gradually dying unless diligently nursed back toward self-maintenance.Citation63 Yet, the traditional “pleasure/reward system” interpretation for MFB arousal, which many still agree is basically incorrect, remains the commonly preferred historical label. However, the ethological behavior patterns provoked by DBS of this circuitry closely resemble the natural appetitive-exploratory phases of motivated behavior. Such states of mind are more akin to “interest” and “enthusiasm”—namely, feelings in short supply among depressed individuals. In short, such positively valenced psychobehavioral “arousals” encourage organisms to energetically pursue all rewards that support survival (including reproduction)—accompanied by an affective state that is more “appetitive” rather than “consummatory” (a state not well described simply as “rewarding”).
Accordingly, our group contemplated that prolonged social loss, as reflected in affectively negative overarousal of separation distress (PANIC), would diminish SEEKING, an effect that was preclinically easily modeled.Citation38 Accordingly, DBS of the MFB was deemed a reasonable treatment for treatment-resistant depression.Citation64 Since these emotional systems had not been well characterized in humans, we conducted relevant diffusion tensor imaging (DTI), as summarized by Coenen et al,Citation65 that provided needed stereotaxic coordinates for potential MFB-DBS clinical studies for depression.
Preliminary results from seven treatment-resistant patients treated at University of Bonn have been published.Citation66 Beneficial effects in six of the seven patients ranged from modest to substantial, with antidepressant effects typically evident within a day, and generally larger than those reported for other DBS brain sites such as nucleus accumbens and subgenual cingulate.
Conclusions and future directions
Our desire to understand the feelings of core emotional processes of animals in preclinical affective modeling of psychiatric disorders has so far guided the development of new antidepressant therapies more productively than mere behavioral modeling. Clearly, a sincere, scientifically guided conversation about the affective processes of animal brains is needed and is gradually emerging.Citation67 As resistance to the use of experiential (eg, affective) concepts in animal models diminishes, we should be able to develop new treatments for other disorders related to irritable imbalances of RAGE, FEAR, and PANIC systems. For instance, with the characterization of preclinical effects of many neuropeptides identified along emotional circuitsCitation62 we can anticipate, just for instance, that: (i) the Substance P-receptor antagonist aprepitant should exert measureable anti-irritability effects in humans, (ii) diverse neuropeptide antagonists, ranging from cholecystokinin (CCK) to corticotropin-releasing factor (CRF) antagonists may ameliorate diverse subtypes of anxiety; and (iii) to the degree that existing work on separation-distress circuitry of animals illuminates the neural infrastructure of panic attacks and borderline personality disorders, beside safe opioids, future molecules that stimulate oxytocin and prolactin receptors of the brain should provide benefits, especially when combined with various psychotherapies.Citation32,Citation33,Citation68
Also, we need better models of how the primordial subcortical substrates of consciousness, heavily laden with affective circuits, control the developmental progressions of upper brain-mind maturation. This may be achieved through detailed understanding of the neuroaffective underpinnings of commonly used animal learning and memory models, facilitated by the most recent technologies (from optogenetics to Designer Receptors Exclusively Activated by Designer Drugs [DREADD]Citation69). These may provide deeper understanding of how affective processes regulate the paths of higher-order secondary- and tertiary-process learning and cognitive mechanisms that integrate feelings into higher-order thoughts and cognitive decision-making processes. The core argument here is that preclinical research for illuminating the neural nature of affective processes will enrich our conceptualizations of how human psychiatric disorders arise. It also provides a novel approach to begin scientifically understanding the minds of other animals. The most important criterion for the accuracy of our scientific visions in preclinical modeling needs to be measured by the new treatments that various approaches generate.
A cross-species affective neuroscience offers a heuristic vision to empirically understand the neural controls of our primal emotional feelings, which suggests ways to develop new therapeutics for human affective disorders. Although the return of opiates into psychiatric practice (which Kraepelin advocated in extreme cases of depression) remains a cultural challenge, we might wish to consider the following three points: (i) Kraepelin did advocate a careful deployment of such maneuvers when nothing else had worked; (ii) many opiate addicts have probably self-medicated them-selves, while not realizing that by elevating doses they were promoting the intensity of withdrawal effects and thereby promoting even deeper depression; and (iii) the loving touch of human care and kindness, promoted by endogenous opioid release, constitutes what we now call placebo effects.Citation70
REFERENCES
- PankseppJ.The vicissitudes of preclinical psychiatric research: justified abandonment by big pharma?Future Neurol.201272113115
- LeDouxJ.Rethinking the emotional brain.Neuron.201273465367622365542
- MarkouA.Animal models of depression and antidepressant activity.Neurosci Biobehav Rev.2005294-5501
- PankseppJ.Affective neuroscience of the emotional BrainMind: evolutionary perspectives and implications for understanding depression.Dialogues Clin Neurosci.201012453354521319497
- WatsonJB.Behaviorism. New York, NY: W. W. Norton;1929
- SkinnerBF.Science and Human Behavior. New York, NY: Simon & Schuster;195360
- TinbergenN.The Study of Instinct. New York, NY: Oxford University Press;195115
- DawkinsMS.Why Animals Matter: Animal Consciousness, Animal Welfare, and Human Well-being. Oxford, UK: Oxford University Press;2012
- OldsJ.MilnerP.Positive reinforcement produced by electrical stimulation of septal area and other regions of rat brain.J Comp Physiol Psychol.195447641942713233369
- DelgadoJM.RobertsWW.MillerNE.Learning motivated by electrical stimulation of the brain.Am J Physiol.1954179358759313228630
- PankseppJ.Hypothalamic integration of behavior: rewards, punishments, and related psychobiological process. In: Morgane PJ, Panksepp J, eds.Handbook of the Hypothalamus; vol 3, part A: Behavioral Studies of the Hypothalamus. New York, NY: Marcel Dekker;1981289487
- HeathR.Exploring the Mind-Body Relationship. Baton Rouge, LA: Moran Printing;1996
- PankseppJ.Mood changes. In: Vinken P, Bruyn G, Klawans H, eds.Handbook of Clinical Neurology. vol 45. Amsterdam, the Netherlands: Elsevier;1985271285
- PankseppJ.Toward a general psychobiological theory of emotions.Behav Brain Sci.19825407422
- PankseppJ.Affective Neuroscience: The Foundations of Human and Animal Emotions. New York, NY: Oxford University Press;1998
- RollsET.Emotion and Decision-Making Explained. Oxford, UK: Oxford University Press;2014
- RollsET.Emotion and decision-making explained: response to commentators.Cortex.20156220321024981275
- MerkerB.Consciousness without a cerebral cortex: a challenge for neuroscience and medicine.Behav Brain Sci.2007306313417475053
- PankseppJ.Emotional endophenotypes in evolutionary psychiatry.Prog Neuropsychopharmacol Biol Psychiatry.200630577478416554114
- PankseppJ.BivenL.Archaeology of Mind. New York, NY: Norton;2011
- LiottiM.PankseppJ.On the neural nature of human emotions and implications for biological psychiatry. In: Panksepp J, ed.Textbook of Biological Psychiatry. Hoboken, NJ: Wiley;20043374
- BradyJV.NautaWJ.Subcortical mechanisms in emotional behavior: the duration of affective changes following septal and habenular lesions in the albino rat.J Comp Physiol Psychol.195548541242013271610
- PankseppJ.Toward the constitution of emotional feelings: synergistic lessons from Izard's differential emotions theory and affective neuroscience.Emotion Rev.201572110115
- NorthoffG.SchneiderF.RotteM.et alDifferential parametric modulation of self-relatedness and emotions in different brain regions.Human Brain Mapping.200930236938218064583
- DamasioAR.GrabowskiTJ.BecharaA.et alSubcortical and cortical brain activity during the feeling of self-generated emotions.Nat Neurosci.20003101049105611017179
- ZubietaJK.KetterTA.BuellerJA.et alRegulation of human affective responses by anterior cingulate and limbic mu-opioid neurotransmission.Arch Gen Psychiatry.200360111145115314609890
- PankseppJ.NormansellLA.HermanB.et alNeural and neurochemical control of the separation distress call. In: Newman JD, ed.The Physiological Control of Mammalian Vocalizations. New York, NY: Plenum;1988263300
- PankseppJ.Cross-species affective neuroscience decoding of the primal affective experiences of humans and related animals.PLoS One.201169e2123621915252
- HubelDH.WieselTN.Receptive fields, binocular interaction and functional architecture in the cat's visual cortex.J Physiol.196216010615414449617
- SurM.RubinsteinJL.Patterning and plasticity of the cerebral cortex.Science.2005310574980581016272112
- FlorioM.AlbertM.TavernaE.et alHuman-specific gene ARHGAP11B promotes basal progenitor amplification and neocortex expansion.Science.201534762291465147025721503
- LaneRD.RyanL.NadelL.GreenbergL.Memory reconsolidation, emotional arousal, and the process of change in psychotherapy: new insights from brain science.Behav Brain Sci.201538e124827452
- SolmsM.The Feeling Brain. London, UK: Karnac Books;2015
- SolmsM.PankseppJ.The “id” knows more than the “ego” admits: neuropsychoanalytic and primal consciousness perspectives on the interface between affective and cognitive neuroscience.Brain Sci.20122214717524962770
- PankseppJ.NormansellLA.CoxJF.SiviyS.Effects of neonatal decortication on the social play of juvenile rats.Physiol Behav.19945634294437972392
- LeGros ClarkWE.BeattieJ.RiddochG.DottNM.The Hypothalamus: Morphological, Functional, Clinical and Surgical Aspects. Edinburgh, UK: Oliver &Boyd;1938
- IkemotoS.PankseppJ.The role of nucleus accumbens dopamine in motivated behavior: a unifying interpretation with special reference to reward seeking.Brain Res Rev.19993164110611493
- WrightJS.PankseppJ.Toward affective circuit-based preclinical models of depression: sensitizing dorsal PAG arousal leads to sustained suppression of positive affect in rats.Neurosci Biobehav Rev.20113591902191521871918
- WattDF.PankseppJ.Depression: an evolutionarily conserved mechanism to terminate separation-distress? A review of aminergic, peptidergic, and neural network perspectives.Neuropsychoanalysis.20091115104
- PankseppJ.WrightJS.DöbrössyMD.SchlaepferTE.CoenenVA.Affective neuroscience strategies for understanding and treating depressions: from preclinical models to novel therapeutics.Clin Psychol Sci.201424472494
- PankseppJ.YovellY.Preclinical modeling of primal emotional affects (SEEKING, PANIC and PLAY): gateways to the development of new treatments for depression.Psychopathology.201447638339325341411
- BowlbyJ.Loss: Sadness and Depression. London, UK: Hogarth Press; 1980. Attachment and Loss. Vol 3
- PankseppJ.HermanB.ConnerR.BishopP.ScottJP.The biology of social attachments: opiates alleviate separation distress.Biol Psychiatr.1978135607618
- HermanBH.PankseppJ.Ascending endorphinergic inhibition of distress vocalization.Science.19812114486106010627466377
- PankseppJ.HermanBH.VilbergT.BishopP.DeEskinaziFG.Endogenous opioids and social behavior.Neurosci Biobehav Rev:1980444734876258111
- TenorePL.Psychotherapeutic benefits of opioid agonist therapy.J Addict Dis.2008273496518956529
- BodkinJA.ZornbergGL.LukasSE.ColeJO.Buprenorphine treatment of refractory depression.J Clin Psychopharmacol.199515149577714228
- YovellY.BarG.MashiahM.et alUltra- low-dose buprenorphine as a time-limited treatment for severe suicidal ideation: a randomized, controlled trial.Am J Psychiatry. In press.
- PankseppJ.SiviyS.NormansellLA.The psychobiology of play: theoretical and methodological perspectives.Neurosci Biobehav Rev1984844654926392950
- PankseppJ.BeattyWW.Social deprivation and play in rats.Behav Neural Biol.19803021972067447871
- PankseppJ.BurgdorfJ.50k-Hz chirping (laughter?) in response to conditioned and unconditioned tickle-induced reward in rats: Effects of social housing and genetic variables.Behav Brain Res.20001151253810996405
- BurgdorfJ.PankseppJ.BrudzynskiSM.MoskalJR.Breeding for 50k-Hz positive affective vocalizations in rats.Behav Genet.2005351677215674533
- BurgdorfJ.PankseppJ.BrudzynskiSM.et alThe effects of selective breeding for differential rates of 50-kHz ultrasonic vocalizations on emotional behavior in rats.Dev Psychobiol.2009511344618819097
- BurgdorfJ.WoodPL.KroesRA.MoskalJR.PankseppJ.Neurobiology of 50-kHz ultrasonic vocalizations in rats: electrode mapping, lesion, and pharmacology studies.Behav Brain Res.2007182227428317449117
- BurgdorfJ.PankseppJ.MoskalJR.Frequency-modulated 50 kHz ultrasonic vocalizations: a tool for uncovering the molecular substrates of positive affect.Neurosci Biobehav Rev20113591831183621144859
- BurgdorfJ.KroesRA.BeinfeldMC.PankseppJ.MoskalJR.Uncovering the molecular basis of positive affect using rough-and-tumble play in rats: a role for insulin-like growth factor I.Neuroscience.2010168376977720350589
- MoskalJR.BurchR.BurgdorfJ.et alGLYX-13, an NMDA receptor glycine site functional partial agonist enhances cognition and produces antidepressant effects without the psychotomimetic side effects of NMDA receptor antagonists.Expert Opin investig Drugs.2014232243254
- NormansellL.PankseppJ.Glutamatergic modulation of separation distress: profound emotional effects of excitatory amino acids in chicks.Neurosci Biobehav Rev.20113591890190121704069
- BurgdorfJ.ZhangXL.NicholsonKL.et alGLYX-13, a NMDA receptor glycine-site functional partial agonist, induces antidepressant-like effects without ketamine-like side effects.Neuropsychopharmacol.2013385729742
- PreskornS.MacalusoM.MehraV.et alRandomized proof of concept trial of GLYX-13, an N-Methyl-D-Aspartate receptor glycine site partial agonist, in major depressive disorder nonresponsive to a previous antidepressant agent.J Psychiatr Pract.201 521214014925782764
- MoskalJR.BurgdorfJ.KroesRA.BrudzynskiSM.PankseppJ.A novel NMDA receptor glycine-site partial agonist, GLYX-13, has therapeutic potential for the treatment of autism.Neurosci Biobehav Rev.20113591982198821718719
- PankseppJ.HarroJ.Future of neuropeptides in biological psychiatry and emotional psychopharmacology: goals and strategies. In: Panksepp J, ed.Textbook of Biological Psychiatry. Hoboken, NJ: Wiley-Liss;2004627660
- TeitelbaumP.EpsteinAN.The lateral hypothalamic syndrome: recovery of feeding and drinking after lateral hypothalamic lesions.Psychol Rev.196269749013920110
- CoenenVA.SchlaepferTE.MaedlerB.PankseppJ.Cross-species affective functions of the medial forebrain bundle—implications for the treatment of affective pain and depression in humans.Neurosci Biobehav Rev.20113591971198121184778
- CoenenVA.PankseppJ.HurwitzTA.UrbachH.MädlerB.Human medial forebrain bundle (MFB) and anterior thalamic radiation (ATR): Diffusion tensor imaging of two major subcortical pathways that may promote a dynamic balance of opposite affects relevant for understanding depression.J Neuropsychiatry Clin Neurosci.201224222323622772671
- SchlaepferTE.BewernickB.KayserS.MädlerB.CoenenVA.Rapid effects of deep brain stimulation for treatment resistant depression.Biol Psychiatr.2013731212041212
- PankseppJ.Foreword: Perspectives on passages toward an affective neurobiology of mind? In: Koob GF, Le Moal M, Thompson RF, eds.Encyclopedia of Behavioral Neuroscience. Boston, MA: Elsevier Inc;2010iviii
- ClariciA.PellizzoniS.GuaschinoS.et alIntranasal administration of oxytocin in postnatal depression: implications for psychodynamic psychotherapy from a randomized double-blind pilot study.Front Psychol.2015642625941501
- KravitzAV.BonciA.Optogenetics, physiology and emotions.Front Behav Neurosci.2013716924312032
- PeciñaM.BohnertAS.SikoraM.et alAssociation between placebo-activated neural systems and antidepressant responses: Neurochemistry of placebo effects in major depression.JAMA Psychiatry201572111087109426421634